Development of an affordable extrusion 3D bioprinter equipped with a temperature-controlled printhead
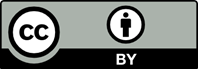
Bioprinters show great promise as enablers of regenerative medicine and other biomedical engineering applications. In this work, we present a flexible and cost-effective design for a do-it-yourself bioprinter capable of printing/bioprinting gelatin methacryloyl (GelMA) and Pluronic constructs at flow rates of 0.05–0.1 mL/min and effective resolutions of 500–700 μm. The most distinctive feature of this bioprinter is its ability to control the rheology of bioinks simply by adjusting the extrusion temperature during printing. This is achieved by circulating temperature-controlled water within the printhead, which is engineered as a single 3D-printed component consisting of a water-recirculation jacket surrounding the ink/bioink cartridge. The flexibility to circulate either warm or cold water allows the system to be adapted according to the needs dictated by the bioink composition. Herein, we demonstrate the ability to control the printability of GelMA or Pluronic fibers by decreasing or increasing the temperature, respectively, thereby regulating its viscosity. In addition, any commercial needle with a Luer lock can be incorporated into the printhead, allowing the easy fabrication of fibers of different diameters with a single printhead. We showed that our bioprinter is capable of printing simple 2D constructs with high fidelity (i.e., lines of GelMA with a thickness of ~522 ± 36.83 μm can be printed at linear speeds of 100 mm min−1) and 3D constructs composed of as many as five layers of cell-laden 5% GelMA. We also demonstrated that C2C12 cells bioprinted through needle tips (300 μm in diameter) exhibit adequate post-printing viability (~90%), as well as spreading after 7 days of culture. The presentation of this bioprinter may contribute appreciably to the expansion of bioprinter use due to its low overall cost of manufacture, flexibility and open-source character, amenability to modification and adaptation for use with different 3D-printed printheads, and ability to bioprint using GelMA.
- Atala A, Forgacs G, 2019, Three-dimensional bioprinting in regenerative medicine: Reality, hype, and future. Stem Cells Transl Med, 8(8):744–745. https//doi.org/10.1002/sctm.19-0089
- Garciamendez-Mijares CE, Agrawal P, García Martínez G, et al., 2021, State-of-art affordable bioprinters: A guide for the DiY community. Appl Phys Rev, 8(3):031312. https//doi.org/10.1063/5.0047818
- Thakur PC, Cabrera DD, DeCarolis N, et al., 2018, Innovation and commercialization strategies for three-dimensional-bioprinting technology: A lean business model perspective. J Commer Biotechnol, 24(1):78–87. https//doi.org/10.5912/jcb856
- Zou Q, Grottkau BE, He Z, et al., 2020, Biofabrication of valentine-shaped heart with a composite hydrogel and sacrificial material. Mater Sci Eng C, 108:110205. https//doi.org/10.1016/j.msec.2019.110205
- Osouli-Bostanabad K, Masalehdan T, Kapsa RM, et al., 2022, Traction of 3D and 4D printing in the healthcare industry: From drug delivery and analysis to regenerative medicine. ACS Biomater Sci Eng, 8(7):2764–2797. https//doi.org/10.1021/ACSBIOMATERIALS.2C00094
- Choudhury D, Anand S, Naing MW, 2018, The arrival of commercial bioprinters—Towards 3D bioprinting revolution! Int J Bioprinting, 4(2):139. https//doi.org/10.18063/IJB.v4i2.139
- Ke D, Niu C, Yang X, 2022, Evolution of 3D bioprinting-from the perspectives of bioprinting companies. Bioprinting, 25:e00193. https//doi.org/10.1016/J.BPRINT.2022.E00193
- Xu K, Han Y, Huang Y, et al., 2022, The application of 3D bioprinting in urological diseases. Mater Today Bio, 16:100388. https//doi.org/10.1016/J.MTBIO.2022.100388
- Shinkar K, Rhode K, 2022, Could 3D extrusion bioprinting serve to be a real alternative to organ transplantation in the future? Ann 3D Print Med, 7:100066. https//doi.org/10.1016/J.STLM.2022.100066
- Panda S, Hajra S, Mistewicz K, et al., 2022, A focused review on three-dimensional bioprinting technology for artificial organ fabrication. Biomater Sci, 10(18):5054–5080. https//doi.org/10.1039/D2BM00797E
- Zhang B, Luo Y, Ma L, et al., 2018, 3D bioprinting: An emerging technology full of opportunities and challenges. Bio-Design Manuf, 1(1):2–13. https//doi.org/10.1007/S42242-018-0004-3
- De Maria C, Díaz Lantada A, Jämsä T, et al., 2022, Biomedical engineering in low- and middle-income settings: Analysis of current state, challenges and best practices. Health Technol (Berl), 12(3):643–653. https//doi.org/10.1007/S12553-022-00657-8/FIGURES/7
- McElheny C, Hayes D, Devireddy R, 2017, Design and fabrication of a low-cost three-dimensional bioprinter. J Med Devices Trans ASME, 11(4):1–9. https//doi.org/10.1115/1.4037259
- Bisram RY, Bender LM, Niemi SR, et al., Toward Economical 3D Bioprinters for High School Science Laboratories.
- Allevi, Allevi 1. https://www.allevi3d.com/allevi-1/.
- CellInk, Holograph X. https://www.cellink.com/bioprinting/holograph-x/
- Ozbolat IT, Hospodiuk M, 2016, Current advances and future perspectives in extrusion-based bioprinting. Biomaterials, 76:321–343. https//doi.org/10.1016/j.biomaterials.2015.10.076
- Kahl M, Gertig M, Hoyer P, et al., 2019, Ultra-low-cost 3D bioprinting: Modification and application of an off-the-shelf desktop 3D-printer for biofabrication. Front Bioeng Biotechnol, 7(July):1–12. https//doi.org/10.3389/fbioe.2019.00184
- Yenilmez B, Temirel M, Knowlton S, et al., 2019, Development and characterization of a low-cost 3D bioprinter. Bioprinting, 13(March):e00044. https//doi.org/10.1016/j.bprint.2019.e00044
- Krige A, Haluška J, Rova U, et al., 2021, Design and implementation of a low cost bio-printer modification, allowing for switching between plastic and gel extrusion. HardwareX, 9:e00186. https//doi.org/10.1016/J.OHX.2021.E00186
- Pérez Cortez JE, Sánchez VH, Vázquez-Lepe E, et al., 2022, Retrofitting of an affordable 3D printer: Towards a material efficient and low-cost bioprinting system. Procedia CIRP, 110:150–155. https//doi.org/10.1016/J.PROCIR.2022.06.028
- Wagner M, Karner A, Gattringer P, et al., 2021, A super low-cost bioprinter based on DVD-drive components and a raspberry pi as controller. Bioprinting, 23:e00142. https//doi.org/10.1016/J.BPRINT.2021.E00142
- McCormack A, Highley CB, Leslie NR, et al., 2020, 3D printing in suspension baths: Keeping the promises of bioprinting afloat. Trends Biotechnol, 38(6):584–593. https//doi.org/10.1016/j.tibtech.2019.12.020
- Liu F, Liu C, Chen Q, et al., 2018, Progress in organ 3D bioprinting. Int J Bioprint, 4(1):1–15. https//doi.org/10.18063/IJB.v4i1.128
- Liu W, Heinrich MA, Zhou Y, et al., 2017, Extrusion bioprinting of shear-thinning gelatin methacryloyl bioinks. Adv Healthc Mater, 6(12):1601451. https//doi.org/10.1002/adhm.201601451
- Maciel BR, Baki K, Oelschlaeger C, et al., 2022, The influence of rheological and wetting properties of hydrogel-based bio-inks on extrusion-based bioprinting. Chem Ing Tech, 94(3):393–401. https//doi.org/10.1002/CITE.202100139
- Talluri DJS, Nguyen HT, Avazmohammadi R, et al., 2022, Ink rheology regulates stability of bioprinted strands. J Biomech Eng, 144(7):074503. https//doi.org/10.1115/1.4053404/1131087
- Yue K, Trujillo-de Santiago G, Alvarez MM, et al., 2015, Synthesis, properties, and biomedical applications of gelatin methacryloyl (GelMA) hydrogels. Biomaterials, 73:254–271. https//doi.org/10.1016/j.biomaterials.2015.08.045
- Bolívar-Monsalve EJ, Ceballos-González CF, Borrayo- Montaño KI, et al., 2021, Continuous chaotic bioprinting of skeletal muscle-like constructs. Bioprinting, 21:e00125. https//doi.org/10.1016/J.BPRINT.2020.E00125
- Celikkin N, Mastrogiacomo S, Dou W, et al., 2022, In vitro and in vivo assessment of a 3D printable gelatin methacrylate hydrogel for bone regeneration applications. J Biomed Mater Res Part B Appl Biomater, 110(9):2133–2145. https//doi.org/10.1002/JBM.B.35067
- Ying G, Jiang N, Yu C, et al., 2018, Three-dimensional bioprinting of gelatin methacryloyl (GelMA). Bio-Design Manuf, 1(4):215–224. https//doi.org/10.1007/s42242-018-0028-8
- West J, Kuk G, 2016, The complementarity of openness: How MakerBot leveraged Thingiverse in 3D printing. Technol Forecast Soc Change, 102:169–181. https//doi.org/10.1016/J.TECHFORE.2015.07.025
- Magrisso S, Zoran A, 2019, Digital joinery for hybrid carpentry. Lect Notes Civ Eng, 24:441–461. https//doi.org/10.1007/978-3-030-03676-8_16
- Gillispie GJ, Han A, Uzun-Per M, et al., 2020, The influence of printing parameters and cell density on bioink printing outcomes. Tissue Eng Part A, 26(23–24):1349–1358. https//doi.org/10.1089/TEN.TEA.2020.0210
- Tavares-Negrete JA, Aceves-Colin AE, Rivera-Flores DC, et al., 2021, Three-dimensional printing using a maize protein: Zein-based inks in biomedical applications. ACS Biomater Sci Eng, 7(8):3964–3979. https//doi.org/10.1021/ACSBIOMATERIALS.1C00544/ SUPPL_FILE/AB1C00544_SI_004.MOV
- Ioannidis K, Danalatos RI, Champeris Tsaniras S, et al., 2020, A custom ultra-low-cost 3D bioprinter supports cell growth and differentiation. Front Bioeng Biotechnol, 8:1279. https//doi.org/10.3389/FBIOE.2020.580889/BIBTEX
- Bolívar-Monsalve EJ, Ceballos‐González CF, Chávez‐ Madero C, et al., 2022, One-step bioprinting of multi-channel hydrogel filaments using chaotic advection: Fabrication of pre-vascularized muscle-like tissues. Adv Healthc Mater, 11(24):2200448. https//doi.org/10.1002/ADHM.202200448
- Kolesky DB, Truby RL, Gladman AS, et al., 2014, 3D bioprinting of vascularized, heterogeneous cell-laden tissue constructs. Adv Mater, 26(19):3124–3130. https//doi.org/10.1002/ADMA.201305506
- Jalaal M, Cottrell G, Balmforth N, et al., 2016, On the rheology of Pluronic F127 aqueous solutions. J Rheol (NY), 61(1):139. https//doi.org/10.1122/1.4971992
- Lenaerts V, Triqueneaux C, Quartern M, et al., 1987, Temperature-dependent rheological behavior of Pluronic F-127 aqueous solutions. Int J Pharm, 39(1–2):121–127. https//doi.org/10.1016/0378-5173(87)90206-7
- Xu C, Zhang M, Huang Y, et al., 2014, Study of droplet formation process during drop-on-demand inkjetting of living cell-laden bioink. Langmuir, 30(30):9130–9138. https//doi.org/10.1021/la501430x
- Schwartz R, Malpica M, Thompson GL, et al., 2020, Cell encapsulation in gelatin bioink impairs 3D bioprinting resolution. J Mech Behav Biomed Mater, 103:103524. https//doi.org/10.1016/J.JMBBM.2019.103524