Bioprinting of hydrogel beads to engineer pancreatic tumor-stroma microtissues for drug screening
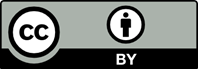
Pancreatic ductal adenocarcinoma (PDAC) having features of dense fibrotic stromal and extracellular matrix (ECM) components has poor clinical outcome. In vitro construction of relevant preclinical PDAC models recapitulating the tumor-stroma characteristics is therefore in great need for the development of pancreatic cancer therapy. In this work, a three-dimensional (3D) heterogeneous PDAC microtissue based on a dot extrusion printing (DEP) system is reported. Gelatin methacryloyl (GelMA) hydrogel beads encapsulating human pancreatic cancer cells and stromal fibroblasts were printed, which demonstrated the capacity of providing ECM-mimetic microenvironments and thus mimicked the native cell-cell junctions and cell-ECM interactions. Besides, the spherical structure of the generated hydrogel beads, which took the advantage of encapsulating cells in a reduced volume, enabled efficient diffusion of oxygen, nutrients and cell waste, thus allowing the embedded cells to proliferate and eventually form a dense pancreatic tumor-stroma microtissue around hundred microns. Furthermore, a tunable stromal microenvironment was easily achieved by adjusting the density of stromal cells in the hydrogel beads. Based on our results, the produced heterogeneous pancreatic microtissue recapitulated the features of cellular interactions and stromal-like microenvironments, and displayed better anti-cancer drug resistance than mono-cultured pancreatic cancer spheroids. Together, the DEP system possesses the ability to simply and flexibly produce GelMA hydrogel beads, providing a robust manufacturing tool for the pancreatic cancer drug screening platform fabrication. In addition, the engineered pancreatic tumor-stroma microtissue based on bioprinted GelMA hydrogel beads, other than being ECM-biomimetic and stroma-tunable, can be used for observation in situ and may serve as a new drug screening platform.
1. Makohon-Moore A, Iacobuzio-Donahue CA, 2016, Pancreatic cancer biology and genetics from an evolutionary perspective. Nat Rev Cancer, 16: 553–565. https://doi.org/10.1038/nrc.2016.66
2. Wolfgang CL, Herman JM, Laheru DA, et al., 2013, Recent progress in pancreatic cancer. CA Cancer J Clin, 63: 318–348. https://doi.org/10.3322/caac.21190
3. Siegel RL, Miller KD, Jemal A, 2018, Cancer statistics, 2018. CA Cancer J Clin, 68(1): 7–30. https://doi.org/10.3322/caac.21442
4. Stathis A, Moore MJ, 2010, Advanced pancreatic carcinoma: Current treatment and future challenges. Nat Rev Clin Oncol, 7: 163–172. https://doi.org/10.1038/nrclinonc.2009.236
5. Yang J, Xu R, Wang C, et al., 2021, Early screening and diagnosis strategies of pancreatic cancer: A comprehensive review. Cancer Commun (Lond), 41: 1257–1274. https://doi.org/10.1002/cac2.12204
6. Heinrich MA, Mostafa AM, Morton JP, et al., 2021, Translating complexity and heterogeneity of pancreatic tumor: 3D in vitro to in vivo models. Adv Drug Deliv Rev, 174: 265–293. https://doi.org/10.1016/j.addr.2021.04.018
7. Ren B, Cui M, Yang G, et al., 2018, Tumor microenvironment participates in metastasis of pancreatic cancer. Mol Cancer, 17(1): 108. https://doi.org/10.1186/s12943-018-0858-1
8. Huang X, Ding L, Liu XK, et al., 2021, Regulation of tumor microenvironment for pancreatic cancer therapy. Biomaterials, 270: 120680. https://doi.org/10.1016/j.biomaterials.2021.120680
9. Uzunparmak B, Sahin IH, 2019, Pancreatic cancer microenvironment: A current dilemma. Clin Transl Med, 8: 2. https://doi.org/10.1186/s40169-019-0221-1
10. Wang S, Zheng Y, Yang F, et al., 2021, The molecular biology of pancreatic adenocarcinoma: Translational challenges and clinical perspectives. Signal Transduct Target Ther, 6(1): 249. https://doi.org/10.1038/s41392-021-00659-4
11. Valkenburg KC, De Groot AE, Pienta KJ, 2018, Targeting the tumour stroma to improve cancer therapy. Nat Rev Clin Oncol, 15: 366–381. https://doi.org/10.1038/s41571-018-0007-1
12. Monteiro MV, Ferreira LP, Rocha M, et al., 2022, Advances in bioengineering pancreatic tumor-stroma physiomimetic biomodels. Biomaterials, 287: 121653. https://doi.org/10.1016/j.biomaterials.2022.121653
13. Tomás-Bort E, Kieler M, Sharma S, et al., 2020, 3D approaches to model the tumor microenvironment of pancreatic cancer. Theranostics, 10(11): 5074–5089. https://doi.org/10.7150/thno.42441
14. Schnittert J, Bansal R, Prakash J, 2019, Targeting pancreatic stellate cells in cancer. Trends Cancer, 5: 128–142. https://doi.org/10.1016/j.trecan.2019.01.001
15. Thomas D, Radhakrishnan P, 2019, Tumor-stromal crosstalk in pancreatic cancer and tissue fibrosis. Mol Cancer, 18(1): 14. https://doi.org/10.1186/s12943-018-0927-5
16. Zhan HX, Zhou B, Cheng YG, et al., 2017, Crosstalk between stromal cells and cancer cells in pancreatic cancer: New insights into stromal biology. Cancer Lett, 392: 83–93. https://doi.org/10.1016/j.canlet.2017.01.041
17. Yang WG, Cai SX, Yuan Z, et al., 2019, Mask-free generation of multicellular 3D heterospheroids array for high-throughput combinatorial anti-cancer drug screening. Mater Design, 183: 108182. https://doi.org/10.1016/j.matdes.2019.108182
18. Zhuang P, Chiang YH, Fernanda MS, et al., 2021, Using spheroids as building blocks towards 3D bioprinting of tumor microenvironment. Int J Bioprint, 7: 444.
https://doi.org/10.18063/ijb.v7i4.444
19. Antunes J, Gaspar VM, Ferreira L, et al., 2019, In-air production of 3D co-culture tumor spheroid hydrogels for expedited drug screening. Acta Biomater, 94: 392–409. https://doi.org/10.1016/j.actbio.2019.06.012
20. Monteiro MV, Gaspar VM, Mendes L, et al., 2021, Stratified 3D microtumors as organotypic testing platforms for screening pancreatic cancer therapies. Small Methods, 5: 2001207. https://doi.org/10.1002/smtd.202001207
21. Monteiro MV, Rocha M, Gaspar VM, et al., 2022, Programmable living units for emulating pancreatic tumor-stroma interplay. Adv Healthc Mater, 11: e2102574. https://doi.org/10.1002/adhm.202102574
22. Murphy SV, De Coppi P, Atala A, 2020, Opportunities and challenges of translational 3D bioprinting. Nat Biomed Eng, 4: 370–80. https://doi.org/10.1038/s41551-019-0471-7
23. Ayan B, Heo DN, Zhang Z, et al., 2020, Aspiration-assisted bioprinting for precise positioning of biologics. Sci Adv, 6: eaaw5111. https://doi.org/10.1126/sciadv.aaw5111
24. Matai I, Kaur G, Seyedsalehi A, et al., 2020, Progress in 3D bioprinting technology for tissue/organ regenerative engineering. Biomaterials, 226: 119536. https://doi.org/10.1016/j.biomaterials.2019.119536
25. Xie MJ, Gao Q, Zhao HM, et al., 2019, Electro-assisted bioprinting of low-concentration GelMA microdroplets. Small, 15: 1804216. https://doi.org/10.1002/smll.201804216
26. Clua-Ferré L, De Chiara F, Rodríguez-Comas J, et al., 2022, Collagen-tannic acid spheroids for β-cell encapsulation fabricated using a 3D bioprinter. Adv Mater Technol-US, 7: 2101696. https://doi.org/10.1002/admt.202101696
27. Vennin C, Murphy KJ, Morton JP, et al., 2018, Reshaping the tumor stroma for treatment of pancreatic cancer. Gastroenterology, 154: 820–838. https://doi.org/10.1053/j.gastro.2017.11.280
28. Rubiano A, Delitto D, Han S, et al., 2018, Viscoelastic properties of human pancreatic tumors and in vitro constructs to mimic mechanical properties. Acta Biomater, 67: 331–340. https://doi.org/10.1016/j.actbio.2017.11.037
29. Looi CK, Chung FF, Leong CO, et al., 2019, Therapeutic challenges and current immunomodulatory strategies in targeting the immunosuppressive pancreatic tumor microenvironment. J Exp Clin Canc Res, 38: 162. https://doi.org/10.1186/s13046-019-1153-8
30. Rimal R, Desai P, Daware R, et al., 2022, Cancer-associated fibroblasts: Origin, function, imaging, and therapeutic targeting. Adv Drug Deliv Rev, 189: 114504. https://doi.org/10.1016/j.addr.2022.114504
31. Han CC, Liu TY, Yin R, 2020, Biomarkers for cancer-associated fibroblasts. Biomark Res, 8: 64. https://doi.org/10.1186/s40364-020-00245-w
32. Lee JH, Kim SK, Khawar IA, et al., 2018, Microfluidic co-culture of pancreatic tumor spheroids with stellate cells as a novel 3D model for investigation of stroma-mediated cell motility and drug resistance. J Exp Clin Canc Res, 37: 4. https://doi.org/10.1186/s13046-017-0654-6
33. Mehrpouya M, Pourhashem Z, Yardehnavi N, et al., 2019, Evaluation of cytokeratin 19 as a prognostic tumoral and metastatic marker with focus on improved detection methods. J Cell Physiol, 234: 21425–21435. https://doi.org/10.1002/jcp.28768
34. Von Ahrens D, Bhagat TD, Nagrath D, et al., 2017, The role of stromal cancer-associated fibroblasts in pancreatic cancer. J Hematol Oncol, 10: 76. https://doi.org/10.1186/s13045-017-0448-5
35. Ligorio M, Sil S, Malagon-Lopez J, et al., 2019, Stromal microenvironment shapes the intratumoral architecture of pancreatic cancer. Cell, 178: 160–175. https://doi.org/10.1016/j.cell.2019.05.012
36. Ware MJ, Keshishian V, Law JJ, et al., 2016, Generation of an in vitro 3D PDAC stroma rich spheroid model. Biomaterials, 108: 129–142. https://doi.org/10.1016/j.biomaterials.2016.08.041
37. Berlin J, Benson AB 3rd, 2010, Gemcitabine remains the standard of care for pancreatic cancer. Nat Rev Clin Oncol, 7: 135–137. https://doi.org/10.1038/nrclinonc.2010.16
38. Liu HQ, Shi Y, Qian F, 2021, Opportunities and delusions regarding drug delivery targeting pancreatic cancer-associated fibroblasts. Adv Drug Deliv Rev, 172: 37–51. https://doi.org/10.1016/j.addr.2021.02.012
39. Benton G, Arnaoutova I, George J, et al., 2014, Matrigel: From discovery and ECM mimicry to assays and models for cancer research. Adv Drug Deliv Rev, 79-80: 3–18. https://doi.org/10.1016/j.addr.2014.06.005
40. Liu HY, Korc M, Lin CC, 2018, Biomimetic and enzyme-responsive dynamic hydrogels for studying cell-matrix interactions in pancreatic ductal adenocarcinoma. Biomaterials, 160: 24–36. https://doi.org/10.1016/j.biomaterials.2018.01.012
41. Ying GL, Jiang N, Yu CJ, et al., 2018, Three-dimensional bioprinting of gelatin methacryloyl (GelMA). Biodes Manuf, 1: 215–224. https://doi.org/10.1007/s42242-018-0028-8
42. Gudapati H, Dey M, Ozbolat I, 2016, A comprehensive review on droplet-based bioprinting: Past, present and future. Biomaterials, 102: 20–42. https://doi.org/10.1016/j.biomaterials.2016.06.012
43. Miri AK, Hosseinabadi HG, Cecen B, et al., 2018, Permeability mapping of gelatin methacryloyl hydrogels. Acta Biomater, 77: 38–47. https://doi.org/10.1016/j.actbio.2018.07.006
44. Zhou MM, Lee BH, Tan LP, 2017, A dual crosslinking strategy to tailor rheological properties of gelatin methacryloyl. Int J Bioprint, 3(2): 130–137. https://doi.org/10.18063/IJB.2017.02.003
45. Xu P, Guan J, Chen Y, et al., 2021, Stiffness of photocrosslinkable gelatin hydrogel influences nucleus pulposus cell properties in vitro. J Cell Mol Med, 25: 880–991. https://doi.org/10.1111/jcmm.16141
46. Yang Y, Xu R, Wang C, et al., 2022, Recombinant human collagen-based bioinks for the 3D bioprinting of full-thickness human skin equivalent. Int J Bioprint, 8: 611. http://doi.org/10.18063/ijb.v8i4.611
47. Yang T, Zhang QY, Xie L, et al., 2021, hDPSC-laden GelMA microspheres fabricated using electrostatic microdroplet method for endodontic regeneration. Mater Sci Eng C Mater Biol Appl, 121: 111850. https://doi.org/10.1016/j.msec.2020.111850
48. Tanaka HY, Kurihara T, Nakazawa T, et al., 2020, Heterotypic 3D pancreatic cancer model with tunable proportion of fibrotic elements. Biomaterials, 251: 120077. https://doi.org/10.1016/j.biomaterials.2020.120077
49. Wu FL, Yang J, Liu J, et al., 2021, Signaling pathways in cancer-associated fibroblasts and targeted therapy for cancer. Signal Transduct Target Ther, 6: 218. https://doi.org/10.1038/s41392-021-00641-0
50. Boyd LN, Andini KD, Peters GJ, et al., 2022, Heterogeneity and plasticity of cancer-associated fibroblasts in the pancreatic tumor microenvironment. Semin Cancer Biol, 82: 184–196. https://doi.org/10.1016/j.semcancer.2021.03.006
51. Yang J, Li YZ, Sun ZW, et al., 2021, Macrophages in pancreatic cancer: An immunometabolic perspective. Cancer Lett, 498: 188–200. https://doi.org/10.1016/j.canlet.2020.10.029
52. Nielsen MF, Mortensen MB, Detlefsen S, 2016, Key players in pancreatic cancer-stroma interaction: Cancer-associated fibroblasts, endothelial and inflammatory cells. World J Gastroenterol, 22: 2678–2700. https://doi.org/10.3748/wjg.v22.i9.2678