Mammalian-specific decellularized matrices derived bioink for bioengineering of liver tissue analogues: A review
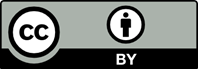
The absolute shortage of compatible liver donors and the growing number of potential recipients have led scientists to explore alternative approaches to providing tissue/ organ substitutes from bioengineered sources. Bioartificial regeneration of a fully functional tissue/organ replacement is highly dependent on the right combination of engineering tools, biological principles, and materiobiology horizons. Over the past two decades, remarkable achievements have been made in hepatic tissue engineering by converging various advanced interdisciplinary research approaches. Three-dimensional (3D) bioprinting has arisen as a promising state-of-the-art tool with strong potential to fabricate volumetric liver tissue/organ equivalents using viscosity- and degradation-controlled printable bioinks composed of hydrous microenvironments, and formulations containing living cells and associated supplements. Source of origin, biophysiochemical, or thermomechanical properties and crosslinking reaction kinetics are prerequisites for ideal bioink formulation and realizing the bioprinting process. In this review, we delve into the forecast of the potential future utility of bioprinting technology and the promise of tissue/organspecific decellularized biomaterials as bioink substrates. Afterward, we outline various methods of decellularization, and the most relevant studies applying decellularized bioinks toward the bioengineering of in vitro liver models. Finally, the challenges and future prospects of decellularized material-based bioprinting in the direction of clinical regenerative medicine are presented to motivate further developments.
1. Abouna GM, 2001, The humanitarian aspects of organ transplantation. Transpl Intl, 14:117–123. https://doi.org/10.1007/s001470050859
2. Godown J, McKane M, Wujcik K, et al., 2016, Expanding the donor pool: Regional variation in pediatric organ donation rates. Pediatr Transplant, 20:1093–1097. https://doi.org/10.1111/petr.12779
3. Cippà PE, 2019, New ideas for old problems: How scientific advances can change the future of organ transplantation. Transpl Intl, 32:561–562. https://doi.org/10.1111/tri.13419
4. Kitajima T, Kuno Y, Ivanics T, et al., 2022, Improved survival with higher-risk donor grafts in liver transplant with acute-on-chronic liver failure. Transplant Direct, 8:e1283. https://doi.org/10.1097/TXD.0000000000001283
5. Amin A, Panayotova G, Guarrera JV, 2022, Hypothermic machine perfusion for liver graft preservation. Curr Opin Organ Transplant, 27:98–105. https://doi.org/10.1097/MOT.0000000000000973
6. Kojima H, Yasuchika K, Fukumitsu K, et al., 2018, Establishment of practical recellularized liver graft for blood perfusion using primary rat hepatocytes and liver sinusoidal endothelial cells. Am J Transplant, 18:1351–1359. https://doi.org/10.1111/ajt.14666Citation
7. Sampaziotis F, Muraro D, Tysoe OC, et al., 2021, Cholangiocyte organoids can repair bile ducts after transplantation in the human liver. Science, 371:839–846. https://doi.org/10.1126/science.aaz6964
8. Antarianto RD, Pragiwaksana A, Septiana WL, et al., 2021, Hepatocyte differentiation from iPSCs or MSCs in decellularized liver scaffold: Cell-ECM adhesion, spatial distribution, and hepatocyte maturation profile. Organogenesis, 18:2061263. https://doi.org/10.1080/15476278.2022.2061263
9. Langer R, Vacanti JP, 1999, Tissue engineering. Science, 260:920–926. https://doi.org/10.1126/science.8493529
10. Ashammakhi N, GhavamiNejad A, Tutar R, et al., 2022, Highlights on advancing frontiers in tissue engineering. Tissue Eng Part B Rev, 28:633–664. https://doi.org/10.1089/ten.TEB.2021.0012
11. Park KM, Shin YM, Kim K, et al., 2018, Tissue engineering and regenerative medicine 2017: A year in review. Tissue Eng Part B Rev, 24:327–344. https://doi.org/10.1089/ten.TEB.2018.0027
12. Kobayashi J, Kikuchi A, Aoyagi T, et al., 2019, Cell sheet tissue engineering: Cell sheet preparation, harvesting/ manipulation, and transplantation. J Biomed Mater Res A, 107:955–967. https://doi.org/10.1002/jbm.a.36627
13. Mehrban N, Teoh GZ, Birchall M, 2015, 3D bioprinting for tissue engineering: Stem cells in hydrogels. Int J Bioprint, 6-19. https://doi.org/10.18063/IJB.2016.01.006
14. Fayon A, Menu P, Omar RE, 2021, Cellularized small-caliber tissue-engineered vascular grafts: Looking for the ultimate gold standard, NPJ Regen Med, 6:46. https://doi.org/10.1038/s41536-021-00155-x
15. Sadtler K, Singh A, Wolf MT, et al., 2016, Design, clinical translation and immunological response of biomaterials in regenerative medicine. Nat Rev Mater, 1:1–17. https://doi.org/10.1038/natrevmats.2016.40
16. Ohashi K, Yokoyama T, Yamato M, et al., 2007, Engineering functional two- and three-dimensional liver systems in vivo using hepatic tissue sheets. Nat Med, 13:880–885. https://doi.org/10.1038/nm1576
17. Mazza G, Al-Akkad W, Rombouts K, et al., 2018 Liver tissue engineering: From implantable tissue to whole organ engineering, Hepatol Commun, 2:131–141. https://doi.org/10.1002/hep4.1136
18. Hollister SJ, 2009, Scaffold design and manufacturing: From concept to clinic. Adv Mater, 21:3330–3342. https://doi.org/10.1002/adma.200802977
19. Huang D, Gibeley SB, Xu C, et al., 2020, Engineering liver microtissues for disease modeling and regenerative medicine. Adv Funct Mater, 30:1909553. https://doi.org/10.1002/adfm.201909553
20. Cho S, Discher DE, Leong KW, et al., 2022, Challenges and opportunities for the next generation of cardiovascular tissue engineering. Nat Methods, 19:1064–1071. https://doi.org/10.1038/s41592-022-01591-3
21. Mir TA, Nakamura M, 2017, Three-dimensional bioprinting: Toward the era of manufacturing human organs as spare parts for healthcare and medicine, Tissue Eng Part B Rev, 23:245–256. https://doi.org/10.1089/ten.TEB.2016.0398
22. Moroni L, Burdick JU, Highley C, et al., 2018, Biofabrication strategies for 3D in vitro models and regenerative medicine. Nat Rev Mater, 3:21–37. https://doi.org/10.1038/s41578-018-0006-y
23. Mir TA, Iwanaga S, Kurooka T, et al., 2019, Biofabrication offers future hope for tackling various obstacles and challenges in tissue engineering and regenerative medicine: A perspective. Int J Bioprint, 5:153. https://doi.org/10.18063/ijb.v5i1.153
24. Arai K, Yoshida T, Okabe M, et al., 2017, Fabrication of 3D-culture platform with sandwich architecture for preserving liver-specific functions of hepatocytes using 3D bioprinter. J Biomed Mater Res A, 105:1583–1592. https://doi.org/10.1002/jbm.a.35905
25. Bertassoni LE, 2022, Bioprinting of complex multicellular organs with advanced functionality-recent progress and challenges ahead. Adv Mater, 34:e2101321. https://doi.org/10.1002/adma.202101321
26. Chae S, Yong U, Park W, et al., 2023, 3D cell-printing of gradient multi-tissue interfaces for rotator cuff regeneration. Bioact Mater, 19:611–625. https://doi.org/10.1016/j.bioactmat.2022.05.004
27. Gantumur E, Nakahata M, Kojima M, et al., 2020, Extrusion-based bioprinting through glucose-mediated enzymatic hydrogelation. Int J Bioprint, 6:250. https://doi.org/10.18063/ijb.v6i1.250
28. Groll J, Boland T, Blunk T, et al., 2016, Biofabrication: reappraising the definition of an evolving field. Biofabrication, 8(1):013001. https://doi.org/10.1088/1758-5090/8/1/013001
29. Mironov V, Boland T, Trusk T, et al., 2003, Organ printing: Computer-aided jet-based 3D tissue engineering. Trends Biotechnol, 21:157-161. https://doi.org/10.1016/S0167-7799(03)00033-7
30. Kang KH, Hockaday LA, Duan B, et al., 2013, 3D Bioprinting of heterogeneous aortic valve conduits with alginate/gelatin hydrogels. J Biomed Mat Res A, 101(5):1255–1264. https://doi.org/10.1002/jbm.a.34420
31. Sears NA, Seshadri DR, Dhavalikar PS, et al., 2015, A review of three-dimensional printing in tissue engineering. Tissue Eng Part B Rev, 298–310. https://doi.org/10.1089/ten.teb.2015.0464
32. Elomaa L, Yang YP, 2017, Additive manufacturing of vascular grafts and vascularized tissue constructs. Tissue Eng Part B Rev, 5:436–450. https://doi.org/10.1089/ten.teb.2016.0348
33. Matai I, Kaur G, Seyedsalehi A, et al., 2020, Progress in 3D bioprinting technology for tissue/organ regenerative engineering. Biomaterials, 226:119536. https://doi.org/10.1016/j.biomaterials.2019.119536
34. Moroni L, Boland T, Burdick JA, et al., 2018, Biofabrication: A guide to technology and terminology. Trends Biotechnol, 36:384–402. https://doi.org/10.1016/j.tibtech.2017.10.015
35. Pereira RF, Bártolo PJ, 2015, 3D bioprinting of photocrosslinkable hydrogel constructs. J Appl Polym Sci, 132:42458. https://doi.org/10.1002/app.42458
36. Mandrycky C, Wang Z, Kim K, et al., 2016, 3D bioprinting for engineering complex tissues. Biotechnol Adv, 34: 422–434. https://doi.org/10.1016/j.biotechadv.2015.12.011
37. Saunders RE, Derby B, 2014, Inkjet printing biomaterials for tissue engineering: Bioprinting. Int Mater Rev, 59:430–448. https://doi.org/10.1179/1743280414Y.0000000040
38. Ozbolat IT, Hospodiuk M, 2016, Current advances and future perspectives in extrusion-based bioprinting. Biomaterials, 76:321–343. https://doi.org/10.1016/j.biomaterials.2015.10.076
39. Gudapati H, Dey M, Ozbolat I, 2016, A comprehensive review on droplet-based bioprinting: Past, present and future. Biomaterials, 102:20–42. https://doi.org/10.1016/j.biomaterials.2016.06.012
40. Guillemot F, Souquet A, Catros S, et al., 2010, High-throughput laser printing of cells and biomaterials for tissue engineering. Acta Biomater, 6:2494–2500. https://doi.org/10.1016/j.actbio.2009.09.029
41. Ozbolat IT, Moncal KK, Gudapati H, 2017, Evaluation of bioprinter technologies. Addit Manuf, 13:179–200. https://doi.org/10.1016/j.addma.2016.10.003
42. Ouyang L, Highley CB, Sun W, et al., 2017, A generalizable strategy for the 3D bioprinting of hydrogels from nonviscous photo-crosslinkable inks. Adv Mater, 29:1604983. https://doi.org/10.1002/adma.201604983
43. Duan B, Hockaday LA, Kang KH, et al., 2013, 3D bioprinting of heterogeneous aortic valve conduits with alginate/gelatin hydrogels. J Biomed Mater Res A, 101:1255–1264. https://doi.org/10.1002/jbm.a.34420
44. Zhu W, Ma X, Gou M, et al., 2016, 3D printing of functional biomaterials for tissue engineering. Curr Opin Biotechnol, 40:103–112. https://doi.org/10.1016/j.copbio.2016.03.014
45. Sarig-Nadir O, Livnat N, Zajdman R, et al., 2009, Laser photoablation of guidance microchannels into hydrogels directs cell growth in three dimensions. Biophys J, 96: 4743–4752. https://doi.org/10.1016/j.bpj.2009.03.019
46. Park S, Choi G, Kim JM, et al., 2022, 3D printing model of abdominal cavity of liver transplantation recipient to prevent large-forsize syndrome. Int J Bioprint, 8(4):609. https://doi.org/10.18063/ijb.v8i4.609
47. Xie F, Sun L, Pang Y, et al., 2021, Three-dimensional bio-printing of primary human hepatocellular carcinoma for personalized medicine. Biomaterials, 265:120416. https://doi.org/10.1016/j.biomaterials.2020.120416
48. Bouwmeester MC, Bernal PN, Oosterhoff LA, et al., 2021, Bioprinting of human liver-derived epithelial organoids for toxicity studies. Macromol Biosci, 21(12):e2100327. https://doi.org/10.1002/mabi.202100327
49. Taymour R, Kilian D, Ahlfeld T, et al., 2021, 3D bioprinting of hepatocytes: Core–shell structured co-cultures with fibroblasts for enhanced functionality. Sci Rep, 11:5130. https://doi.org/10.1038/s41598-021-84384-6
50. Nakamura M, Mir TA, Arai K, et al., 2015, Bioprinting with pre-cultured cellular constructs towards tissue engineering of hierarchical tissues. Int J Bioprint, 1(1):39–48. https://dx.doi.org/10.18063/IJB.2015.01.007
51. Ma L, Wu Y, Li Y, et al., 2020, Current advances on 3D-bioprinted liver tissue models. Adv Healthc Mater, 9(24):e2001517. https://doi.org/10.1002/adhm.202001517
52. Xu K, Han Y, Huang Y, et al., 2022, The application of 3D bioprinting in urological diseases. Mat Today Bio, 2(16):100388. https://doi.org/10.1016/j.mtbio.2022.100388
53. Murphy SV, De Coppi P, Atala A, 2019, Opportunities and challenges of translational 3D bioprinting. Nat Biomed Eng, 4:370–380. https://doi.org/10.1038/s41551-019-0471-7
54. Weekes A, Bartnikowski N, Pinto N, et al., 2022, Biofabrication of small diameter tissue-engineered vascular grafts. Acta Biomater, 138:92–111. https://doi.org/10.1016/j.actbio.2021.11.012
55. Taormina G, Sciancalepore C, Messori M, et al., 2018, 3D printing processes for photocurable polymeric materials: Technologies, materials, and future trends. J Appl Biomater Funct Mater, 16:151–160. https://doi.org/10.1177/2280800018764770
56. Krkobabić M, Medarević D, Pešić N, et al., 2020, Digital light processing (DLP) 3D printing of atomoxetine hydrochloride tablets using photoreactive suspensions. Pharmaceutics, 12:E833. https://doi.org/10.3390/pharmaceutics12090833
57. Ozbolat IT, 2015, Bioprinting scale-up tissue and organ constructs for transplantation, Trends Biotechnol, 33:395–400. https://doi.org/10.1016/j.tibtech.2015.04.005
58. Rengier F, Mehndiratta A, Tengg-Kobligk HV, et al., 2010, 3D printing based on imaging data: Review of medical applications. Int J Comput Assist Radiol Surg, 5:335–341. https://doi.org/10.1007/s11548-010-0476-x
59. Stampfl J, Baudis S, Heller C, et al., 2008, Photopolymers with tunable mechanical properties processed by laser-based high-resolution stereolithography. J Micromech Microeng, 18:125014. https://doi.org/10.1088/0960-1317/18/12/125014
60. Tumbleston JR, Shirvanyants D, Ermoshkin N, et al., 2015, Additive manufacturing. Continuous liquid interface production of 3D objects. Science, 347:1349–1352. https://doi.org/10.1126/science.aaa2397
61. Murphy SV, Atala A, 2014, 3D bioprinting of tissues and organs. Nat Biotechnol, 32:773–785. https://doi.org/10.1038/nbt.2958
62. Guillotin B, Guillemot F, 2011, Cell patterning technologies for organotypic tissue fabrication. Trends Biotechnol, 29:183–190. https://doi.org/10.1016/j.tibtech.2010.12.008
63. Arai K, Iwanaga S, Toda H, et al., 2011, Three-dimensional inkjet biofabrication based on designed images. Biofabrication, 3:034113. https://iopscience.iop.org/article/10.1088/1758-5082/3/3/034113
64. Choudhury D, Anand S, Naing MW, 2018, The arrival of commercial bioprinters—Towards 3D bioprinting revolution. Int J Bioprint, 4:139. https://doi.org/10.18063/IJB.v4i2.139
65. Li X, Liu B, Pei B, et al., 2020, Inkjet bioprinting of biomaterials. Chem Rev, 120:10793–10833. https://doi.org/10.1021/acs.chemrev.0c00008
66. Gu Z, Fu J, Lin H, et al., 2020, Development of 3D bioprinting: From printing methods to biomedical applications. Asian J Pharm Sci, 15:529–557. https://doi.org/10.1016/j.ajps.2019.11.003
67. Levato R, Jungst T, Scheuring RG, et al., 2020, From shape to function: The next step in bioprinting. Adv Mater, 1906423. https://doi. org/10.1002/adma.201906423
68. Carlos Mota C, Camarero-Espinosa S, Baker MB, et al., 2020, Bioprinting: From tissue and organ development to in vitro models. Chem Rev, 120(19):10547–10607. https://doi.org/10.1021/acs.chemrev.9b00789
69. Jorgensen AM, Yoo JJ, Anthony A, 2020, Solid organ bioprinting: Strategies to achieve organ function. Chem Rev, 120(19):11093–11127. https://doi.org/10.1021/acs.chemrev.0c00145
70. Wang Y, Li J, Li Y, et al., 2021, Biomimetic bioinks of nanofibrillar polymeric hydrogels for 3D bioprinting. Nano Today, 39:101180. https://doi.org/10.1016/j.nantod.2021.101180
71. Lee J, Lee S, Ahmad T, et al., 2020, Human adipose-derived stem cell spheroids incorporating platelet-derived growth factor (PDGF) and bio-minerals for vascularized bone tissue engineering. Biomaterials, 255:120192. https://doi.org/10.1016/j.biomaterials.2020.120192
72. Kim EM, Lee YB, Kim SJ, et al., 2019, Fabrication of core-shell spheroids as building blocks for engineering 3D complex vascularized tissue. Acta Biomater, 100:158–172. https://doi.org/10.1016/j.actbio.2019.09.028
73. Nakamura M, Iwanaga S, Henmi C, et al., 2010, Biomatrices and biomaterials for future developments of bioprinting and biofabrication. Biofabrication, 2(1):014110. https://iopscience.iop.org/article/10.1088/1758-5082/2/1/014110
74. George J, Hsu CC, Ba LT, et al., 2020, Neural tissue engineering with structured hydrogels in CNS models and therapies. Biotechnol Adv, 42:107370. https://doi.org/10.1016/j.biotechadv.2019.03.009
75. Pereira RF, Bártolo PJ, 2015, 3D photo-fabrication for tissue engineering and drug delivery. Engineering, 1:90–112. https://doi.org/10.15302/J-ENG-2015015
76. Unagolla JM, Jayasuriya AC, 2020, Hydrogel-based 3D bioprinting: A comprehensive review on cell-laden hydrogels, bioink formulations, and future perspectives. Appl Mater Today, 18:100479. https://doi.org/10.1016/j.apmt.2019.100479
77. Sharma S, Tiwari S, 2020, A review on biomacromolecular hydrogel classification and its applications. Int J Biol Macromol, 162:737–747. https://doi.org/10.1016/j.ijbiomac.2020.06.110
78. Ebhodaghe SO, 2020, Hydrogel based biopolymers for regenerative medicine applications: A critical review. Intl J Polym Mater Polym Biomater, 71:155–172. https://doi.org/10.1080/00914037.2020.1809409
79. Catoira MC, Fusaro L, Francesco DD, et al., 2019, Overview of natural hydrogels for regenerative medicine applications. J Mater Sci Mater Med, 30:115. https://doi.org/10.1007/s10856-019-6318-7
80. Zieliński PS, Gudet PKR, Rikmanspoel T, et al., 2022, 3D printing of bio-instructive materials: Toward directing the cell. Bioact Mater, 19:292–327. https://doi.org/10.1016/j.bioactmat.2022.04.008
81. Malda J, Visser J, Melchels FP, et al., 2013, 25th anniversary article: Engineering hydrogels for biofabrication. Adv Mater, 25:5011–5028. https://doi.org/10.1002/adma.201302042
82. Kim BS, Das S, Jang J, et al., 2020, Decellularized extracellular matrix-based bioinks for engineering tissue- and organ-specific microenvironments. Chem Rev, 120:10608–10661. https://doi.org/10.1021/acs.chemrev.9b00808
83. Lee SC, Gillispie G, Prim P, et al., 2020, Physical and chemical factors influencing the printability of hydrogel-based extrusion bioinks. Chem Rev, 120:10834–10886. https://doi.org/10.1021/acs.chemrev.0c00015
84. Zhou K, Sun Y, Yang J, et al., 2022, Hydrogels for 3D embedded bioprinting: A focused review on bioinks and support baths. J Mater Chem B, 10(12):1897–1907. https://doi.org/10.1039/D1TB02554F
85. Arai K, Tsukamoto Y, Yoshida H, et al., 2016, The development of cell-adhesive hydrogel for 3D printing. Int J Bioprint, 2(2):153–162. https://dx.doi.org/10.18063/IJB.2016.02.002
86. Soman SS, Govindraj M, Al Hashimi N, et al., 2022, Bioprinting of human neural tissues using a sustainable marine tunicate derived bioink for translational medicine applications. Int J Bioprint, 8(4):604. https://doi.org/10.18063/ijb.v8i4.604
87. Wang M, Li W, Hao J, et al., 2022, Molecularly cleavable bioinks facilitate high-performance digital light processing-based bioprinting of functional volumetric soft tissues. Nat Commun, 13:3317. https://doi.org/10.1038/s41467-022-31002-2
88. Wang Y, Yuan X, Yao B, et al., 2022, Tailoring bioinks of extrusion-based bioprinting for cutaneous wound healing. Bioact Mater, 17:178–194. https://doi.org/10.1016/j.bioactmat.2022.01.024
89. Khoeini R, Nosrati H, Akbarzadeh A, et al., 2021, Natural and synthetic bioinks for 3D bioprinting. Adv NanoBiomed Res,1:2000097. https://doi.org/10.1002/anbr.202000097
90. Pati F, Jang J, Ha DH, et al., 2014, Printing three-dimensional tissue analogues with decellularized extracellular matrix bioink. Nat Commun, 5:3935. https://doi.org/10.1038/ncomms4935
91. Cui X, Li J, Hartanto Y, et al., 2020, Advances in extrusion 3D bioprinting: A focus on multicomponent hydrogel-based bioinks. Adv Healthc Mater, 9:e1901648. https://doi.org/10.1002/adhm.201901648
92. Sakai S, Nakahata M, 2017, Horseradish peroxidase catalyzed hydrogelation for biomedical, biopharmaceutical, and biofabrication applications. Chem Asian J, 12:3098–3109. https://doi.org/10.1002/asia.201701364
93. Gu Y, Forget A, Shastri VP, 2022, Biobridge: An outlook on translational bioinks for 3D bioprinting. Adv Sci (Weinh), 9:e2103469. https://doi.org/10.1002/advs.202103469
94. Frantz C, Stewart KM, Weaver VM, 2010, The extracellular matrix at a glance. J Cell Sci, 123:4195–4200. https://doi.org/10.1242/jcs.023820
95. Zhang X, Chen X, Hong H, et al., 2022, Decellularized extracellular matrix scaffolds: Recent trends and emerging strategies in tissue engineering. Bioact Mater, 10:15–31. https://doi.org/10.1016/j.bioactmat.2021.09.014
96. Nicolas J, Magli S, Rabbachin L, et al., 2020, 3D extracellular matrix mimics: Fundamental concepts and role of materials chemistry to influence stem cell fate. Biomacromolecules, 21:1968–1994. https://doi.org/10.1021/acs.biomac.0c00045
97. Kuraitis D, Giordano C, Ruel M, et al., 2012, Exploiting extracellular matrix-stem cell interactions: A review of natural materials for therapeutic muscle regeneration. Biomaterials, 33(2):428–443. https://doi.org/10.1016/j.biomaterials.2011.09.078
98. Jain P, Rauer SB, Möller M, et al., 2022, Mimicking the natural basement membrane for advanced tissue engineering. Biomacromolecules, 23:3081–3103. https://doi.org/10.1021/acs.biomac.2c00402
99. Keane TJ, Swinehart IT, Badylak SF, 2015, Methods of tissue decellularization used for preparation of biologic scaffolds and in vivo relevance. Methods, 84:25–34. https://doi.org/10.1016/j.ymeth.2015.03.005
100. Willemse J, Tienderen G, Hengel E, et al., 2022, Hydrogels derived from decellularized liver tissue support the growth and differentiation of cholangiocyte organoids. Biomaterials, 284:121473.
101. Liao J, Xu B, Zhang R, et al., 2020, Applications of decellularized materials in tissue engineering: Advantages, drawbacks and current improvements, and future perspectives. J Mater Chem B, 8:10023–10049. https://doi.org/10.1039/d0tb01534b
102. Lu P, Weaver VM, Werb Z, 2012, The extracellular matrix: A dynamic niche in cancer progression. J Cell Biol, 196:395–406. https://doi.org/10.1083/jcb.201102147
103. Meng F, Almohanna F, Altuhami A, et al., 2018, Vasculature reconstruction of decellularized liver scaffolds via gelatin-based re-endothelialization. J Biomed Res Part A, 4:107. https://doi.org/10.1002/jbm.a.36551
104. Ponticos M, Smith BD, 2014, Extracellular matrix synthesis in vascular disease: Hypertension, and atherosclerosis. J Biomed Res, 28:25–39. https://doi.org/10.7555/JBR.27.20130064
105. Lorenzo P, Bayliss MT, Heinegård D, 2004, Altered patterns and synthesis of extracellular matrix macromolecules in early osteoarthritis. Matrix Biol, 23:381–391. https://doi.org/10.1016/j.matbio.2004.07.007
106. Meng F, Assiri AM, Dhar D, et al., 2017, Whole liver engineering: A promising approach to develop functional liver surrogates. Liver Int, 37:1759–1772. https://doi.org/10.1111/liv.13444
107. Hussey GS, Dziki JL, Badylak SF, 2018, Extracellular matrix-based materials for regenerative medicine. Nat Rev Mater, 3:159–173. https://doi.org/10.1038/s41578-018-0023-x
108. Zhang X, Liu Y, Zuo Q, et al., 2021, 3D bioprinting of biomimetic bilayered scaffold consisting of decellularized extracellular matrix and silk fibroin for osteochondral repair. Int J Bioprint, 74:401. https://doi.org/10.18063/ijb.v7i4.401
109. Chen FM, Liu X, 2016 Advancing biomaterials of human origin for tissue engineering. Prog Polym Sci, 53:86–168. https://doi.org/10.1016/j.progpolymsci.2015.02.004
110. Kim MK, Jeong WW, Lee SM, et al., 2020, Decellularized extracellular matrix-based bio-ink with enhanced 3D printability and mechanical properties. Biofabrication, 12:025003. https://doi.10.1088/1758-5090/ab5d80
111. Rueda-Gensini L, Serna JA, Cifuentes J, et al., 2021, Graphene oxide-embedded extracellular matrix-derived hydrogel as a multiresponsive platform for 3D bioprinting applications. Int J Bioprint, 7(3):353. https://doi.org/10.18063/ijb.v7i3.353
112. Yang R, Xu J, Huang C, 2023, Effect of Ionic crosslinking on morphology and thermostability of biomimetic supercritical fluids-decellularized dermalbased composite bioscaffolds for bioprinting applications. Int J Bioprint, 9(1):625. https://doi.org/10.18063/ijb.v9i1.625
113. Zheng J, Liu Y, Hou C, et al., 2022, Ovary-derived decellularized extracellular matrix-based bioink for fabricating 3D primary ovarian cells-laden structures for mouse ovarian failure correction. Int J Bioprint, 8(3):597. https://doi.org/10.18063/ijb.v8i3.597
114. Choudhury D, Tun HW, Wang T, et al., 2018, Organ-derived decellularized extracellular matrix: A game changer for bioink manufacturing? Trends Biotechnol, 36(8):787–805. https://doi.org/10.1016/j.tibtech.2018.03.003
115. Lee H, Han W, et al., 2017, Development of liver decellularized extracellular matrix bioink for three-dimensional cell printing-based liver tissue engineering. Biomacromolecules, 18:1229–1237. https://doi.org/10.1021/acs.biomac.6b01908
116. Ali M, Kumar A, Yoo JJ, et al., 2019, A photo-crosslinkable kidney ECM-derived bioink accelerates renal tissue formation. Adv Healthc Mater, 8(7):e1800992. https://doi.org/10.1002/adhm.201800992
117. Willemse J, Verstegen MMA, Vermeulen A, et al., 2020, Fast, robust and effective decellularization of whole human livers using mild detergents and pressure controlled perfusion. Mater Sci Eng C Mater Biol Appl, 108:110200. https://doi.org/10.1016/j.msec.2019.110200
118. Felgendreff P, Schindler C, Mussbach F, et al., 2021, Identification of tissue sections from decellularized liver scaffolds for repopulation experiments. Heliyon, 7:e06129. https://doi.org/10.1016/j.heliyon.2021.e06129
119. Shimoda H, Yagi H, Higashi H, et al., 2019, Decellularized liver scaffolds promote liver regeneration after partial hepatectomy. Sci Rep, 9:12543. https://doi.org/10.1038/s41598-019-48948-x
120. Tajima K, Yagi H, Kitagawa Y, 2018, Human-scale liver harvest and decellularization for preclinical research. Methods Mol Biol, 1577:327–335. https://doi.org/10.1007/7651_2018_195
121. Zhang Z, Xu M, Mazza G, et al., 2019, Decellularized human liver scaffold-based three-dimensional culture system facilitate hepatitis B virus infection. J Biomed Mater Res A, 107(8):1744–1753. https://doi.org/10.1002/jbm.a.36690
122. Mazza G, Rombouts K, et al., 2015, Decellularized human liver as a natural 3D-scaffold for liver bioengineering and transplantation. Sci Rep, 5:13079. https://doi.org/10.1038/srep13079
123. Mazza G, Al-Akkad W, Telese A, et al., 2017, Rapid production of human liver scaffolds for functional tissue engineering by high shear stress oscillation-decellularization. Sci Rep, 7:5534. https://doi.org/10.1038/s41598-017-05134-1
124. Skardal A, Devarasetty M, Kang H-W, et al., 2015, A hydrogel bioink toolkit for mimicking native tissue biochemical and mechanical properties in bioprinted tissue constructs. Acta Biomater, 25:24–34. https://doi.org/10.1016/j.actbio.2015.07.030
125. Lee H, Han W, Kim H, et al., 2017, Development of liver decellularized extracellular matrix bioink for three-dimensional cell printing-based liver tissue engineering. Biomacromolecules, 18:1229–1237. https://doi.org/10.1021/acs.biomac.6b01908
126. Yu C, Ma X, Zhu W, et al., 2019, Scanningless and continuous 3D bioprinting of human tissues with decellularized extracellular matrix. Biomaterials, 194:1–13. https://doi.org/10.1016/j.biomaterials.2018.12.009
127. Kim MK, Jeong W, Lee SM, et al., 2020, Decellularized extracellular matrix-based bio-ink with enhanced 3D printability and mechanical properties. Biofabrication, 12:025003. https://doi.org/10.1088/1758-5090/ab5d80
128. Lewis PL, Yan M, Su J, et al., 2019, Directing the growth and alignment of biliary epithelium within extracellular matrix hydrogels. Acta Biomater, 85:84–93. https://doi.org/10.1016/j.actbio.2018.12.039
129. Lee H, Chae S, Kim JY, et al., 2019, Cell-printed 3D liver-on-a-chip possessing a liver microenvironment and biliary system. Biofabrication, 11:025001. https://doi.org/10.1088/1758-5090/aaf9fa
130. Mao Q, Wang Y, Li Y, et al., 2020, Fabrication of liver microtissue with liver decellularized extracellular matrix (dECM) bioink by digital light processing (DLP) bioprinting. Mater Sci Eng C Mater Biol Appl, 109:110625. https://doi.org/10.1016/j.msec.2020.110625
131. Jeong W, Kim MK, Kang HW, 2021, Effect of detergent type on the performance of liver decellularized extracellular matrix-based bio-inks. J Tissue Eng, 12:2041731421997091. https://doi.org/10.1177/2041731421997091
132. Khati V, Ramachandraiah H, Pati F, et al., 2022, 3D bioprinting of multi-material decellularized liver matrix hydrogel at physiological temperatures. Biosensors (Basel), 12:521. https://doi.org/10.3390/bios12070521
133. Zhou G, Jiang H, Yin Z, et al., 2018, In vitro regeneration of patient-specific ear-shaped cartilage and its first clinical application for auricular reconstruction. EBioMedicine, 28:287–302. https://doi.org/10.1016/j.ebiom.2018.01.011
134. Raya-Rivera AM, Esquiliano D, Fierro-Pastrana R, et al., 2014, Tissue-engineered autologous vaginal organs in patients: A pilot cohort study. Lancet, 384: 329–336. https://doi.org/10.1016/S0140-6736(14)60542-0
135. Zopf DA, Hollister SJ, Nelson ME, et al., 2013, Bioresorbable airway splint created with a three-dimensional printer. N Engl J Med, 368:2043–2045. https://doi.org/10.1056/NEJMc1206319
136. Poel WE, 1948, Preparation of acellular homogenates from muscle samples. Science, 108:390–391. https://doi.org/10.1126/science.108.2806.390-a
137. Hjelle JT, Carlson EC, Brendel K, et al., 1979, Biosynthesis of basement membrane matrix by isolated rat renal glomeruli. Kidney Int, 15:20–32. https://doi.org/10.1038/ki.1979.3
138. Badylak SF, Tullius R, Kokini K, et al., 1995, The use of xenogeneic small intestinal submucosa as a biomaterial for Achilles tendon repair in a dog model. J Biomed Mater Res, 29:977–985. https://doi.org/10.1002/jbm.820290809
139. Ott HC, Matthiesen TS, Goh SK, et al., 2008, Perfusion-decellularized matrix: Using nature’s platform to engineer a bioartificial heart. Nat Med, 14:213–221. https://doi.org/10.1038/nm1684
140. Ott HC, Clippinger B, Conrad C, et al., 2010, Regeneration and orthotopic transplantation of a bioartificial lung. Nat Med, 16:927–933. https://doi.org/10.1038/nm.2193
141. Petersen TH, Calle EA, Zhao L, et al., 2010, Tissue-engineered lungs for in vivo implantation. Science, 329: 538–541. https://doi.org/10.1126/science.1189345
142. Uygun BE, Soto-Gutierrez AS, Yagi H, et al., 2010, Organ reengineering through development of a transplantable recellularized liver graft using decellularized liver matrix. Nat Med, 16:814–820. https://doi.org/10.1038/nm.2170
143. Song JJ, Guyette JP, Gilpin SE, et al., 2013, Regeneration and experimental orthotopic transplantation of a bioengineered kidney. Nat Med, 19:646–651. https://doi.org/10.1038/nm.3154
144. Saldin LT, Cramer MC, Velankar SS, et al., 2017, Extracellular matrix hydrogels from decellularized tissues: Structure and function. Acta Biomater, 49:1–15. https://doi.org/10.1016/j.actbio.2016.11.068
145. Garreta E, Oria R, Tarantino C, et al., 2017, Tissue engineering by decellularization and 3D bioprinting. Mater Today, 20:166–178. https://doi.org/10.1016/j.mattod.2016.12.005
146. Chimene D, Kaunas R, Gaharwar AK, 2020, Hydrogel bioink reinforcement for additive manufacturing: A focused review of emerging strategies. Adv Mater, 32:e1902026. https://doi.org/10.1002/adma.201902026
147. Jang J, Park HJ, Kim SW, et al., 2017, 3D printed complex tissue construct using stem cell-laden decellularized extracellular matrix bioinks for cardiac repair, Biomaterials, 112:264–274. https://doi.org/10.1016/j.biomaterials.2016.10.026
148. Abaci A, Guvendiren M, 2020, Designing decellularized extracellular matrix-based bioinks for 3D bioprinting. Adv Healthc Mater, 9(24):e2000734. https://doi.org/10.1002/adhm.202000734
149. Fu RH, Wang YC, Liu SH, et al., 2014, Decellularization and recellularization technologies in tissue engineering. Cell Transplant, 23:621–630. https://doi.org/10.3727/096368914X678382
150. Hussey GS, Dziki JL, Badylak SF, 2018, Extracellular matrix-based materials for regenerative medicine. Nat Rev Mater, 3:159–173. https://doi.org/10.1038/s41578-018-0023-x