3D Printing of Tricalcium Phosphate/Poly Lactic-coglycolic Acid Scaffolds Loaded with Carfilzomib for Treating Critical-sized Rabbit Radial Bone Defects
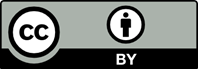
The rapid development of scaffold-based bone tissue engineering strongly relies on the fabrication of advanced scaffolds and the use of newly discovered functional drugs. As the creation of new drugs and their clinical approval often cost a long time and billions of U.S. dollars, producing scaffolds loaded with repositioned conventional drugs whose biosafety has been verified clinically to treat critical-sized bone defect has gained increasing attention. Carfilzomib (CFZ), an approved clinical proteasome inhibitor with a much fewer side effects, is used to replace bortezomib to treat multiple myeloma. It is also reported that CFZ could enhance the activity of alkaline phosphatase and increase the expression of osteogenic transcription factors. With the above consideration, in this study, a porous CFZ/β-tricalcium phosphate/poly lactic-co-glycolic acid scaffold (designated as “cytidine triphosphate [CTP]”) was produced through cryogenic three-dimensional (3D) printing. The hierarchically porous CTP scaffolds were mechanically similar to human cancellous bone and can provide a sustained CFZ release. The implantation of CTP scaffolds into critical-sized rabbit radius bone defects improved the growth of new blood vessels and significantly promoted new bone formation. To the best of our knowledge, this is the first work that shows that CFZ-loaded scaffolds could treat nonunion of bone defect by promoting osteogenesis and angiogenesis while inhibiting osteoclastogenesis, through the activation of the Wnt/β-catenin signaling. Our results suggest that the loading of repositioned drugs with effective osteogenesis capability in advanced bone tissue engineering scaffold is a promising way to treat criticalsized defects of a long bone.
1. Sharif F, Ur Rehman I, Muhammad N, et al., 2016, Dental Materials for Cleft Palate Repair. Mater Sci Eng C Mater Biol Appl, 61:1018–28. https://doi.org/10.1016/j.msec.2015.12.019
2. Marx RE, 2007, Bone and Bone Graft Healing. Oral Maxillofac Surg Clin North Am, 19:455–66.
3. Agarwal R, García AJ, 2015, Biomaterial Strategies for Engineering Implants for Enhanced Osseointegration and Bone Repair. Adv Drug Deliv Rev, 94:53–62. https://doi.org/10.1016/j.addr.2015.03.013
4. Pilliar RM, Filiaggi MJ, Wells JD, et al., 2001, Porous Calcium Polyphosphate Scaffolds for Bone Substitute Applications-In Vitro Characterization. Biomaterials, 22:963–72. https://doi.org/10.1016/s0142-9612(00)00261-1
5. Lai Y, Li Y, Cao H, et al., 2019, Osteogenic Magnesium Incorporated into PLGA/TCP Porous Scaffold by 3D Printing for Repairing Challenging Bone Defect. Biomaterials, 197:207–19. https://doi.org/1 .1016/j.biomaterials.2019.01.013
6. Lee JY, Son SJ, Son JS, et al., 2016, Bone-Healing Capacity of PCL/PLGA/Duck Beak Scaffold in Critical Bone Defects in a Rabbit Model. Biomed Res Int, 2016:2136215. https://doi.org/10.1155/2016/2136215
7. Walsh WR, Vizesi F, Michael D, et al., 2008, Bruceβ-TCP Bone Graft Substitutes in a Bilateral Rabbit Tibial Defect Model. Biomaterials, 29:266–71. https://doi.org/10.1016/j.biomaterials.2007.09.035
8. Huang J, Liu W, Liang Y, et al., 2018, Preparation and Biocompatibility of Diphasic Magnetic Nanocomposite Scaffold. Mater Sci Eng C Mater Biol Appl, 87:70–7.
9. Ji C, Annabi N, Khademhosseini A, et al., 2011, Fabrication of Porous Chitosan Scaffolds for Soft Tissue Engineering using Dense Gas Co2. Acta Biomater, 7:1653–64. https://doi.org/10.1016/j.actbio.2010.11.043
10. Hartgerink JD, Beniash E, Stupp SI, 2002, Peptide-Amphiphile Nanofibers A Versatile Scaffold for the Preparation of Self- Assembling Materials. Proc Natl Acad Sci USA, 99:5133–8. https://doi.org/10.1073/pnas.072699999
11. Green JD, Tollemar V, Dougherty M, et al., 2015, Multifaceted Signaling Regulators of Chondrogenesis Implications in Cartilage Regeneration and Tissue engineering. Genes Dis, 2:307–27.
12. Gaasbeek RD, Toonen HG, van Heerwaarden RJ, et al., 2005, Mechanism of Bone Incorporation of β-TCP Bone Substitute in Open Wedge Tibial Osteotomy in Patients. Biomaterials, 26:6713–9. https://doi.org/10.1016/j.biomaterials.2005.04.056
13. Michalicka M, Boisjoli G, Jahan S, et al., 2017, Human Bone Marrow Mesenchymal Stromal Cell-Derived Osteoblasts Promote the Expansion of Hematopoietic Progenitors Through Beta-Catenin and Notch Signaling Pathways. Stem Cells Dev, 26:1735–48. https://doi.org/10.1089/scd.2017.0133
14. Zhang C, Hu B, Chen Y, et al., 2013, Characterization of the Molecular Mechanism of the Bone-Anabolic Activity of Carfilzomib in Multiple Myeloma. PLoS One, 8:e74191. https://doi.org/10.1371/journal.pone.0074191
15. Hurchla MA, Garcia-Gomez A, Hornick MC, et al., 2012, The Epoxyketone-Based Proteasome Inhibitors Carfilzomib and Orally Bioavailable Oprozomib Have Anti-Resorptive and Bone-Anabolic Activity in Addition to Anti-Myeloma Effects. Leukemia, 27:430–40. https://doi.org/10.1038/leu.2012.183
16. Yang Y, Blair HC, Shapiro IM, et al., 2015, The Proteasome Inhibitor Carfilzomib Suppresses Parathyroid Hormone induced Osteoclastogenesis through a RANKL-Mediated Signaling Pathway. J Biol Chem, 290:16918–28. https://doi.org/10.1074/jbc.m115.663963
17. Maeda K, Kobayashi Y, Koide M, et al., 2019, The Regulation of Bone Metabolism and Disorders by WNT Signaling. Int J Mol Sci, 20:5525.
18. Wei W, Zeve D, Suh JM, et al., 2011, Biphasic and Dosage-Dependent Regulation of Osteoclastogenesis by β-Catenin. Mol Cell Biol, 31:4706–19. https://doi.org/10.1128/mcb.05980-11
19. Coffin JD, Homer-Bouthiette C, Hurley MM, et al., 2018, Fibroblast Growth Factor 2 and Its Receptors in Bone Biology and Disease. J Endocr Soc, 2:657–71. https://doi.org/10.1210/js.2018-00105
20. Zhou H, Mak W, Zheng Y, et al., 2008, Osteoblasts Directly Control Lineage Commitment of Mesenchymal Progenitor Cells through Wnt Signaling. J Biol Chem, 283:1936–45. https://doi.org/10.1074/jbc.m702687200
21. Barbosa MA, Yao D, Xie XH, et al., 2012, Icaritin, an Exogenous Phytomolecule, Enhances Osteogenesis but Not Angiogenesis-An In Vitro Efficacy Study. PLoS One, 7:e41264. https://doi.org/10.1371/journal.pone.0041264
22. Guzzo CM, Nychka JA, 2020, Bone ‘Spackling’ Paste: Mechanical Properties and In Vitro Response of a Porous Ceramic Composite Bone Tissue Scaffold. J Mech Behav Biomed Mater, 112:103958. https://doi.org/10.1016/j.jmbbm.2020.103958
23. Roddy E, DeBaun MR, Daoud-Gray A, et al., 2017, Treatment of Critical-Sized Bone Defectsclinical and Tissue Engineering Perspectives. Eur J Orthop Surg Traumatol, 28:351–62. https://doi.org/10.1007/s00590-017-2063-0
24. Ho-Shui-Ling A, Bolander J, Rustom LE, et al., 2018, Bone Regeneration Strategies: Engineered Scaffolds, Bioactive Molecules and Stem Cells Current Stage and Future Perspectives. Biomaterials, 180:143–62. https://doi.org/10.1016/j.biomaterials.2018.07.017
25. Polo-Corrales L, Latorre-Esteves M, Ramirez-Vick JE, 2014, Scaffold Design for Bone Regeneration. J Nanosci Nanotechnol, 14:15–56. https://doi.org/10.1166/jnn.2014.9127
26. McBride A, Klaus JO, Stockerl-Goldstein K, 2015, Carfilzomib: A Second-Generation Proteasome Inhibitor for the Treatment of Multiple Myeloma. Am J Health Syst Pharm, 72:353–60. https://doi.org/10.2146/ajhp130281
27. Glass DA, Bialek P, Ahn JD, et al., 2005, Canonical WNT Signaling in Differentiated Osteoblasts Controls Osteoclast Differentiation. Dev Cell, 8:751–64. https://doi.org/10.1016/j.devcel.2005.02.017
28. Wang Y, Li M, Li P, et al., 2019, Progress and Applications of Polyphosphate in Bone and Cartilage Regeneration. Biomed Res Int, 2019:5141204.
29. Hadjidakis DJ, Androulakis II, 2006, Bone Remodeling. Ann N Y Acad Sci, 1092:385–96.
30. Grosso A, Burger MG, Lunger A, et al., 2017, It Takes Two to Tango: Coupling of Angiogenesis and Osteogenesis for Bone Regeneration. Front Bioeng Biotechnol, 5:68. https://doi.org/10.3389/fbioe.2017.00068