Rapid and efficient in vivo angiogenesis directed by electro-assisted bioprinting of alginate/collagen microspheres with human umbilical vein endothelial cell coating layer
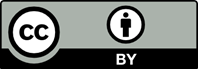
Rapid reconstruction of functional microvasculature is the urgent challenge of regenerative medicine and ischemia therapy development. The purpose of this study was to provide an alternative solution for obtaining functional blood vessel networks in vivo, through assessing whether hydrogel-based microspheres coated by human umbilical vein endothelial cells (HUVECs) can direct rapid and efficient in vivo angiogenesis without the addition of exogenous growth factors or other supporting cells. Uniform alginate microspheres with adjustable diameter were biofabricated by electro-assisted bioprinting technology. Collagen fibrils were evenly coated on the surface of alginate microspheres through simple self-assembly procedure, and collagen concentration is optimized to achieve the highest HUVECs adhesion and proliferation. Immunofluorescence staining and gene analysis confirmed the formation of the prevascularized tubular structure and significantly enhanced endothelial gene expression. HUVECs-coated hydrogel microspheres with different diameters were subcutaneously injected in immunedeficient mice, which demonstrated rapid blood vessel regeneration and functional anastomosis with host blood vessels within 1 week. Besides, microsphere diameter demonstrated influence on blood vessel density with statistical differences but showed no obvious influence on the area occupied by blood vessels. This study provided a powerful tool for rapid and minimalinvasion angiogenesis of bioprinting constructs and a potential method for vascularized tissue regeneration and ischemia treatment with clinically relevant dimensions.
1. Nih LR, Gojgini S, Carmicharel ST, et al., 2018, Dual-function Injectable Angiogenic Biomaterial for the Repair of Brain Tissue Following Stroke. Nat Mater, 17(7):642-51. DOI 10.1038/s41563-018-0083-8.
2. Risau W, 1997, Mechanisms of Angiogenesis. Nature, 386(6626):671-4.
3. Carmeliet P, 2003, Angiogenesis in Health and Disease. Nat Med, 9(6):653-60.
4. Pal A, Vernon BL, Nikkhah M, 2018, Therapeutic Neovascularization Promoted by Injectable Hydrogels. Bioact Mater, 3(4):389-400. DOI 10.1016/j.bioactmat.2018.05.002.
5. De Witte TM, Fratila-Apachitei LE, Zadpoor AA, et al., 2018, Bone Tissue Engineering Via Growth Factor Delivery: From Scaffolds to Complex Matrices. Regen Biomater, 5(4):197- 211. DOI 10.1093/rb/rby013.
6. McGuigan AP, Sefton MV, 2006, Vascularized Organoid Engineered by Modular Assembly Enables Blood Perfusion. Proc Natl Acad Sci U S A, 103(31):11461-6. DOI 10.1073/ pnas.0602740103.
7. McGuigan AP, Leung B, Sefton MV, 2006, Fabrication of Cells Containing Gel Modules to Assemble Modular Tissueengineered Constructs. Nat Protoc, 1(6):2963-9. DOI 10.1038/nprot.2006.443.
8. West MED, Sefton EJB, Sefton MV, 2018, Bone Marrow- Derived Macrophages Enhance Vessel Stability in Modular Engineered Tissues. Tissue Eng Part A. DOI 10.1089/ten. tea.2018.0222.
9. Cooper TP, Sefton MV, 2011, Fibronectin Coating of Collagen Modules Increases in vivo HUVEC Survival and Vessel Formation in SCID Mice. Acta Biomater, 7(3):1072- 83. DOI 10.1016/j.actbio.2010.11.008.
10. McGuigan AP, Sefton MV, 2008, The Thrombogenicity of Human Umbilical Vein Endothelial Cell Seeded Collagen Modules. Biomaterials, 29(16):2453-63. DOI 10.1016/j. biomaterials.2008.02.010.
11. Leong W, Wang DA, 2015, Cell-laden Polymeric Microspheres for Biomedical Applications. Trends Biotechnol, 33(11):653-66. DOI 10.1016/j.tibtech.2015.09.003.
12. Chan JM, Zervantonakis IK, Rimchala T, et al., 2012, Engineering of in vitro 3D Capillary Beds by Self-Directed Angiogenic Sprouting. PLoS One, 7(12):e50582. DOI 10.1371/journal.pone.0050582.
13. Zhang S, Zhou M, Ye Z, et al., 2017, Fabrication of Viable and Functional Pre-vascularized Modular Bone Tissues by Coculturing MSCs and HUVECs on Microcarriers in Spinner Flasks. Biotechnol J, 12(8):1700008. DOI 10.1002/ biot.201700008.
14. Rioja AY, Daley ELH, Habif JC, et al., 2017, Distributed Vasculogenesis from Modular Agarose-hydroxyapatite- Fibrinogen Microbeads. Acta Biomater, 55:144-52. DOI 10.1016/j.actbio.2017.03.050.
15. Ding S, Feng L, Wu J, et al., 2018, Bioprinting of Stem Cells: Interplay of Bioprinting Process, Bioinks, and Stem Cell Properties. ACS Biomaterials Science and Engineering. ACS Biomater Sci Eng, 4(9):3018-124. DOI 10.1021/ acsbiomaterials.8b00399.
16. Roth EA, Xu T, Das M, et al., 2004, Inkjet Printing for High-throughput Cell Patterning. Biomaterials, 25(17):3707-15. DOI 10.1016/j.biomaterials.2003.10.052.
17. An BW, Kim K, Lee H, et al., 2015, High-resolution Printing of 3D Structures Using an Electrohydrodynamic Inkjet with Multiple Functional Inks. Adv. Mater., 27(29):4322-8. DOI 10.1002/adma.201502092.
18. Zhang W, Choi JK, He X, 2017, Engineering Microvascularized 3D Tissue Using Alginate-Chitosan Microcapsules. J Biomater Tissue Eng, 7(2):170-3. DOI 10.1166/jbt.2017.1547.
19. Xie M, Gao Q, Zhao H, et al., 2019, Electro-Assisted Bioprinting of Low-Concentration GelMA Microdroplets. Small, 15(4):e1804216. DOI 10.1002/smll.201804216.
20. Yao R, Zhang R, Luan J, et al., 2012, Alginate and Alginate/ Gelatin Microspheres for Human Adipose-derived Stem Cell Encapsulation and Differentiation. Biofabrication, 4(2):25007. DOI 10.1088/1758-5082/4/2/025007.
21. Wu B, Takeshita N, Wu Y, et al., 2018, Pluronic F127 Blended Polycaprolactone Scaffolds via e-jetting for Esophageal Tissue Engineering. J Mater Sci Mater Med, 29(9):140. DOI 10.1007/s10856-018-6148-z.
22. Kim M, Yun HS, Kim GH, 2017, Electric-field Assisted 3D-fibrous Bioceramic-based Scaffolds for Bone Tissue Regeneration: Fabrication, Characterization, and in vitro Cellular Activities. Sci Rep, 7(1):3166. DOI 10.1038/s41598- 017-03461-x.
23. Wu Y, Han Y, Wong YS, et al., 2018, Fibre-based Scaffolding Techniques for Tendon Tissue Engineering. J Tissue Eng Regen Med, 12(7):1798-821. DOI 10.1002/term.2701.
24. Li XR, Liang H, Sun J, et al., 2015, Electrospun Collagen Fibers with Spatial Patterning of SDF1α for the Guidance of Neural Stem Cells. Adv Healthc Mater, 4(12):1869-76. DOI 10.1002/adhm.201500271.
25. Yao R, Zhang R, Lin F, et al., 2013, Biomimetic Injectable HUVEC-adipocytes/Collagen/Alginate Microsphere co-cultures for Adipose Tissue Engineering. Biotechnol Bioeng, 110(5):1430-43. DOI 10.1002/bit.24784.
26. Yao R, Zhang R, Lin F, et al., 2012, Injectable Cell/Hydrogel Microspheres Induce the Formation of fat Lobule-like Microtissues and Vascularized Adipose Tissue Regeneration. Biofabrication, 4(4):45003. DOI 10.1088/1758- 5082/4/4/045003.
27. Jayasinghe SN, Townsend-Nicholson A, 2006, Stable Electric-field Driven Cone-jetting of Concentrated Biosuspensions. Lab Chip, 6(8):1086-90. DOI 10.1039/b606508m.
28. Xie YB, Castracane J, 2009, High-Voltage, Electric Field-Driven Micro/Nanofabrication for Polymeric Drug Delivery Systems Electrostatic Techniques and Biomedical Application. IEEE Eng Med Biol, 28(1):23-30. DOI 10.1109/ memb.2008.931013.
29. Richardson TP, Peters MC, Ennett AB, et al., 2001, Polymeric System for Dual Growth Factor Delivery. Nat Biotechnol, 19(11):1029-34. DOI 10.1038/nbt1101-1029.
30. Chui CY, Bonilla-Brunner A, Seifert J, et al., 2019, Atomic Force Microscopy-indentation Demonstrates that Alginate Beads are Mechanically Stable Under Cell Culture Conditions. J Mech Behav Biomed Mater, 93:61-9. DOI 10.1016/j.jmbbm.2019.01.019.
31. Augst AD, Kong HJ, Mooney DJ, 2006, Alginate Hydrogels as Biomaterials. Macromol Biosci, 6(8):623-33. DOI 10.1002/mabi.200600069.
32. Uludag H, De Vos P, Tresco PA, 2000, Technology of Mammalian Cell Encapsulation. Adv Drug Deliver Rev, 42(1- 2):29-64. DOI 10.1016/s0169-409x(00)00053-3.
33. Glicklis R, Shapiro L, Agbaria R, et al., 2000, Hepatocyte Behavior within Three-dimensional Porous Alginate Scaffolds. Biotechnol Bioeng, 67(3):344-53. DOI 10.1002/ (sici)1097-0290(20000205)67:3<344::aid-bit11>3.0.co;2-2.
34. Dhoot NO, Tobias CA, Fischer I, et al., 2004, Peptide-modified Alginate Surfaces as a Growth Permissive Substrate for Neurite Outgrowth. J Biomed Mater Res A, 71(2):191- 200. DOI 10.1002/jbm.a.30103.
35. Mosahebi A, Wiberg M, Terenghi G, 2003, Addition of Fibronectin to Alginate Matrix Improves Peripheral Nerve Regeneration in Tissue-engineered Conduits. Tissue Eng, 9(2):209-18. DOI 10.1089/107632703764664684.
36. Prang P, Muller R, Eljaouhari A, et al., 2006, The Promotion of Oriented Axonal Regrowth in the Injured Spinal Cord by Alginate-based Anisotropic Capillary Hydrogels. Biomaterials, 27(19):3560-9. DOI 10.1016/j. biomaterials.2006.01.053.
37. Nerem RM, Seliktar D, 2001, Vascular Tissue Engineering. Annu Rev Biomed Eng, 3:225-43. DOI 10.1146/annurev. bioeng.3.1.225.
38. Weinberg CB, Bell E, 1986, A Blood-vessel Model Constructed from Collagen and Cultured Vascular Cells. Science, 231(4736):397-400. DOI 10.1126/science.2934816.
39. Krock BL, Skuli N, Simon MC, 2011, Hypoxia-induced Angiogenesis: Good and Evil. Genes Cancer, 2(12):1117-33. DOI 10.1177/1947601911423654.
40. Ferrara N, Gerber HP, LeCouter J, 2003, The Biology of VEGF and its Receptors. Nat. Med, 9(6):669-76. DOI 10.1038/nm0603-669.
41. Hauser S, Jung F, Pietzsch J, 2017, Human Endothelial Cell Models in Biomaterial Research. Trends Biotechnol, 35(3):265-77. DOI 10.1016/j.tibtech.2016.09.007.
42. Laschke MW, Harder Y, Amon M, et al., 2006, Angiogenesis in Tissue Engineering: Breathing Life into Constructed Tissue Substitutes. Tissue Eng, 12(8):2093-104. DOI 10.1089/ ten.2006.12.2093.
43. Laschke MW, Menger MD, 2012, Vascularization in Tissue Engineering: Angiogenesis Versus Inosculation. Eur Surg Res, 48(2):85-92. DOI 10.1159/000336876.
44. Ledoux S, Queguiner I, Msika S, et al., 2008, Angiogenesis Associated With Visceral and Subcutaneous Adipose Tissue in Severe Human Obesity. Diabetes, 57(12):3247-57. DOI 10.2337/db07-1812.
45. Koike N, Fukumura D, Gralla O, et al., 2004, Creation of Long-lasting Blood Vessels. Nature, 428(6979):138-9. DOI 10.1038/428138a.