Experimental and numerical approaches for optimizing conjunction area design to enhance switching efficiency in single-nozzle multi-ink bioprinting systems
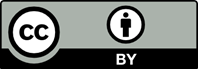
Three-dimensional (3D) bioprinting has emerged as a promising technology in the field of tissue engineering. Notably, the advancement of multi-ink printing technology is crucial for further progress in 3D bioprinting. In this study, we developed a single-nozzle system with multiple inlets for multi-ink bioprinting that achieves high switching efficiency through a combination of numerical and experimental approaches. This single-nozzle system demonstrates the potential for higher-resolution printing and quicker ink switching compared with multi-nozzle printing systems. In general, inks used in bioprinting have low viscosity (<10 Pa·s); however, their behaviors inside a single nozzle have not been thoroughly investigated. Initially, we conducted numerical simulations to analyze fluid behavior within single nozzles, focusing on the junction of multiple ink inlets, to propose an advanced nozzle design. We proposed a novel index called switching efficiency (Se) for evaluating the switching behavior of the bioink inside the single nozzle. Numerical simulation results showed that the nozzle design and combinations of inks affected Se. In addition, subsequent experimental analysis confirmed the consistency of the simulation results. The proposed design, developed using simulations, featured a single nozzle with enhanced switching efficiency, demonstrating a smaller transition length compared with that of conventional single nozzles or T-junction nozzles in printing line structures of different viscous inks. This is the first study to employ numerical simulation in designing a single nozzle with multiple inlets to switch ink in multi-ink bioprinting. This methodology will broaden the potential of single nozzles for high-resolution printing in bioprinting applications.
- Oropallo W, Piegl LA. Ten challenges in 3D printing. Eng Comput. 2016;32(1):135-148. doi: 10.1007/s00366-015-0407-0.
- Sun J, Zhou W, Huang D, Fuh JYH, Hong GS. An overview of 3D printing technologies for food fabrication. Food Bioproc Tech. 2015;8(8):1605-1615. doi: 10.1007/s11947-015-1528-6.
- Duan B. State-of-the-art review of 3D bioprinting for cardiovascular tissue engineering. Ann Biomed Eng. 2017;45(1): 195-209. doi: 10.1007/s10439-016-1607-5
- Ng WL, Chua CK, Shen YF. Print me an organ! Why we are not there yet. Prog Polym Sci. 2019;97:101145. doi: 10.1016/j.progpolymsci.2019.101145
- Singh S, Choudhury D, Yu F, Mironov V, Naing MW. In situ bioprinting – bioprinting from benchside to bedside? Acta Biomater. 2020;101:14-25. doi: 10.1016/j.actbio.2019.08.045
- Vithani K, Goyanes A, Jannin V, Basit AW, Gaisford S, Boyd BJ. An overview of 3D printing technologies for soft materials and potential opportunities for lipid-based drug delivery systems. Pharm Res. 2019;36(1):4. doi: 10.1007/s11095-018-2531-1
- Ramesh S, Harrysson OLA, Rao PK, et al. Extrusion bioprinting: recent progress, challenges, and future opportunities. Bioprinting. 2021;21:e00116. doi: 10.1016/j.bprint.2020.e00116
- Heinrich MA, Liu W, Jimenez A, et al. 3D bioprinting: from benches to translational applications. Small. 2019; 15(23):1–47. doi: 10.1002/smll.201805510
- Derakhshanfar S, Mbeleck R, Xu K, Zhang X, Zhong W, Xing M. 3D bioprinting for biomedical devices and tissue engineering: a review of recent trends and advances. Bioact Mater. 2018;3(2):144-156. doi: 10.1016/j.bioactmat.2017.11.008
- Ng WL, Lee JM, Zhou M, et al. Vat polymerization-based bioprinting - process, materials, applications and regulatory challenges. Biofabrication. 2020;12(2):022001. doi: 10.1088/1758-5090/ab6034
- Miri AK, Mirzaee I, Hassan S, et al. Effective bioprinting resolution in tissue model fabrication. Lab Chip. 2019;19(11):2019-2037. doi: 10.1039/c8lc01037d
- Hernandez-Quintanar L, Rodriguez-Salvador M. Discovering new 3D bioprinting applications: analyzing the case of optical tissue phantoms. Int J Bioprint. 2019; 5(1):1-11. doi: 10.18063/IJB.v5i1.178
- Hinton TJ, Lee A, Feinberg AW. 3D bioprinting from the micrometer to millimeter length scales: size does matter. Curr Opin Biomed Eng. 2017;1:31-37. doi: 10.1016/j.cobme.2017.02.004
- Murphy SV, De Coppi P, Atala A. Opportunities and challenges of translational 3D bioprinting. Nat Biomed Eng. 2020;4(4):370-380. doi: 10.1038/s41551-019-0471-7
- Santoni S, Gugliandolo SG, Sponchioni M, Moscatelli D, Colosimo BM. 3D bioprinting: current status and trends—a guide to the literature and industrial practice. Biodes Manuf. 2021;5:14-42. doi: 10.1007/s42242-021-00165-0
- Gu Z, Fu J, Lin H, He Y. Development of 3D bioprinting: from printing methods to biomedical applications. Asian J Pharm Sci. 2020;15(5):529-557. doi: 10.1016/j.ajps.2019.11.003
- Liu Z, Zhang M, Bhandari B, Wang Y. 3D printing: printing precision and application in food sector. Trends Food Sci Technol. 2017;69:83-94. doi: 10.1016/j.tifs.2017.08.018
- Brunel LG, Hull SM, Heilshorn SC. Engineered assistive materials for 3D bioprinting: support bath and sacrificial inks. Biofabrication. 2022;14:032001. doi: 10.1088/1758-5900/ac6bbe
- Sodupe-Ortega E, Sanz-Garcia A, Pernia-Espinoza A, Escobedo-Lucea C. Accurate calibration in multi-material 3D bioprinting for tissue engineering. Materials. 2018;11(8):1-19. doi: 10.3390/ma11081402
- Hardin JO, Ober TJ, Valentine AD, Lewis JA. Microfluidic printheads for multimaterial 3D printing of viscoelastic inks. Adv Mater. 2015;27(21):3279-3284. doi: 10.1002/adma.201500222
- Skylar-Scott MA, Mueller J, Visser CW, Lewis JA. Voxelated soft matter via multimaterial multinozzle 3D printing. Nature. 2019;575(7782):330-335. doi: 10.1038/s41586-019-1736-8
- Cameron T, Naseri E, Maccallum B, Ahmadi A. Development of a disposable single-nozzle printhead for 3D bioprinting of continuous multi-material constructs. Micromachines (Basel). 2020;11(5):459. doi: 10.3390/mi11050459
- Zhou LY, Gao Q, Fu JZ, et al. Multimaterial 3D printing of highly stretchable silicone elastomers. ACS Appl Mater Interfaces. 2019;11(26):23573-23583. doi: 10.1021/acsami.9b04873
- Tai C, Bouissil S, Gantumur E, et al. Use of anionic polysaccharides in the development of 3D bioprinting technology. Appl Sci. 2019;9(13):2596. doi: 10.3390/app9132596
- Kolesky DB, Homan KA, Skylar-Scott MA, Lewis JA. Three-dimensional bioprinting of thick vascularized tissues. Proc Natl Acad Sci U S A. 2016;113(12):3179-3184. doi: 10.1073/pnas.1521342113
- Jessop ZM, Al-Sabah A, Gao N, et al. Printability of pulp derived crystal, fibril and blend nanocellulose-alginate bioinks for extrusion 3D bioprinting. Biofabrication. 2019;11(4):045006. doi: 10.1088/1758-5090/ab0631
- Nair K, Gandhi M, Khalil S, et al. Characterization of cell viability during bioprinting processes. Biotechnol J. 2009;4(8):1168-1177. doi: 10.1002/biot.200900004
- Nadernezhad A, Khani N, Skvortsov GA, et al. Multifunctional 3D printing of heterogeneous hydrogel structures. Sci Rep. 2016;6:33178. doi: 10.1038/srep33178
- Yang L, Li S, Liu J, Cheng J. Fluid mixing in droplet-based microfluidics with T junction and convergent–divergent sinusoidal microchannels. Electrophoresis. 2018;39(3): 512-520. doi: 10.1002/elps.201700374
- Juraeva M, Kang DJ. Mixing performance of a cross-channel split-and-recombine micro-mixer combined with mixing cell. Micromachines (Basel). 2020;11(7):685. doi: 10.3390/mi11070685
- Shamloo A, Mirzakhanloo M, Dabirzadeh MR. Numerical simulation for efficient mixing of Newtonian and non- Newtonian fluids in an electro-osmotic micro-mixer. Chem Eng Process: Process Intensif. 2016;107:11-20. doi: 10.1016/j.cep.2016.06.003
- Xu K, Ganapathy K, Andl T, et al. 3D porous chitosan-alginate scaffold stiffness promotes differential responses in prostate cancer cell lines. Biomaterials. 2019;217:119311. doi: 10.1016/j.biomaterials.2019.119311
- de Melo BAG, Jodat YA, Mehrotra S, et al. 3D printed cartilage-like tissue constructs with spatially controlled mechanical properties. Adv Funct Mater. 2019;29(51):1-13. doi: 10.1002/adfm.201906330
- Trucco D, Sharma A, Manferdini C, et al. Modeling and fabrication of silk fibroin-gelatin-based constructs using extrusion-based three-dimensional bioprinting. ACS Biomater Sci Eng. 2021;7(7):3306-3320. doi: 10.1021/acsbiomaterials.1c00410
- Köpf M, Nasehi R, Kreimendahl F, Jockenhoevel S, Fischer H. Bioprinting-associated shear stress and hydrostatic pressure affect the angiogenic potential of human umbilical vein endothelial cells. Int J Bioprint. 2022;8(4):96-107. doi: 10.18063/ijb.v8i4.606
- Zaeri A, Zgeib R, Cao K, Zhang F, Chang RC. Numerical analysis on the effects of microfluidic-based bioprinting parameters on the microfiber geometrical outcomes. Sci Rep. 2022;12(1):3364. doi: 10.1038/s41598-022-07392-0
- Sakai S, Mochizuki K, Qu Y, Mail M, Nakahata M, Taya M. Peroxidase-catalyzed microextrusion bioprinting of cell-laden hydrogel constructs in vaporized ppm-level hydrogen peroxide. Biofabrication. 2018;10(4):045007. doi: 10.1088/1758-5090/aadc9e
- Sakai S, Ohi H, Taya M. Gelatin/hyaluronic acid content in hydrogels obtained through blue light-induced gelation affects hydrogel properties and adipose stem cell behaviors. Biomolecules. 2019;9(8):342. doi: 10.3390/biom9080342
- Pössl A, Hartzke D, Schmidts TM, Runkel FE, Schlupp P. A targeted rheological bioink development guideline and its systematic correlation with printing behavior. Biofabrication. 2021;13(3):035021. doi: 10.1088/1758-5090/abde1e
- Zheng J, Zeng R, Zhang F, Kan J. Effects of sodium carboxymethyl cellulose on rheological properties and gelation behaviors of sodium alginate induced by calcium ions. LWT. 2019;103:131-138. doi: 10.1016/j.lwt.2018.12.081
- Hidaka M, Kojima M, Nakahata M, Sakai S. Visible light-curable chitosan ink for extrusion-based and vat polymerization-based 3D bioprintings. Polymers (Basel). 2021;13(9):1382. doi: 10.3390/polym13091382
- Jiong Z, Ruiqi Z, Fusheng Z, Jianquan K. Effects of sodium carboxymethyl cellulose on rheological properties and gelation behaviors of sodium alginate induced by calcium ions. LWT. 2019;103:131-138. doi: 10.1016/j.lwt.2018.12.081
- Minerva AHG, Javier SF, Maribel CM, et al. Protective effect of alginate microcapsules with different rheological behavior on Lactiplantibacillus plantarum 299v. Gels. 2023; 9(9):682. doi: 10.3390/gels9090682
- Emebu S, Ogunleye RO, Achbergerová E, Vítková L, Ponížil P, Martinez CM. Review and proposition for model-based multivariable-multiobjective optimisation of extrusion-based bioprinting. Appl Mater Today. 2023;34:101914. doi: 10.1016/j.apmt.2023.101914
- Elie M, Eric C. Precise method to estimate the Hershel- Bulkley parameters from pipe rheometer measurements. Fluids. 2021;6(4):157. doi: 10.3390/fluids6040157
- Huber T, Pierre G. Extension of the natural element method to surface tension and wettability for the simulation of polymer flows at the micro and nano scales. J Nonnewton Fluid Mech. 2013;300:9-16. doi: 10.1016/j.jnnfm.2012.10.003
- Jalal J, Leong TSH. Microstreaming and its role in applications: a mini-review. Fluids. 2018;3(4):93. doi: 10.3390/fluids3040093
- Wang L, Xu ME, Luo L, Zhou Y, Si P. Iterative feedback bio-printing-derived cell-laden hydrogel scaffolds with optimal geometrical fidelity and cellular controllability. Sci Rep. 2018;8(1):2802. doi: 10.1038/s41598-018-21274-4