Biomechanical analysis of partial mandibular implants with various lattice designs of different material properties: In vitro study and finite element analysis
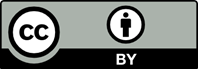
For patients with mandibular bone defects, although reconstruction plates can be used for repair, achieving both occlusal function and facial aesthetics is challenging. In the present study, in vitro experiments and finite element analysis (FEA) were conducted to determine the biomechanical characteristics of multiple porous lattice structures of varying shapes and diameters that were used for mandibular implants. Additionally, an abutment designed to carry occlusal forces was added to the top of the implants. The stress distribution of four lattice designs (tetrahedron, quad-diametral-cross, hex-star, and hex-vase) of three sizes (2.5, 3.0, and 3.5 mm) in cubic porous models were analyzed by FEA. Subsequently, two optimal designs for 3D-printed titanium alloy were selected. These designs, featuring different lattice diameters (0.5, 0.7, and 0.9 mm), were tested to determine their elastic modulus, which was used in another FEA of a mandibular implant designed for a patient with a malignant tumor in the right mandible. This model, which included an abutment design, was subjected to a vertical force of 100 N and muscle forces generated by biting. This analysis was conducted to determine the elastic modulus of the implant and the values of stress and strain on the implant and surrounding bone. The lattice designs of quad-diametral-cross and hex-vase exhibited smaller high-stress regions than those of tetrahedron and hex-star. In vitro tests revealed that the elastic modulus of the lattices increased with the rod diameter. When these values were applied to mandibular implants, Young’s modulus decreased, which in turn increased the frictional stress observed at the interface between the abutment and the implant. However, the implant’s maximum stress remained below its yield strength (910 MPa), and the strain on the surrounding bone varied between 1500 and 3000 μstrain. As indicated by Frost’s theory, these implants are unlikely to damage the surrounding bone tissue and are likely to support bone growth.

- Seikaly H, Chau J, Li F, et al. Bone that best matches the properties of the mandible. J Otolaryngol. 2003;32(4):262-265. doi: 10.2310/7070.2003.41646
- Bak M, Jacobson AS, Buchbinder D, Urken ML. Contemporary reconstruction of the mandible. Oral Oncol. 2010;46(2):71-76. doi: 10.1016/j.oraloncology.2009.11.006
- Schlueter B, Kim KB, Oliver D, Sortiropoulos G. Cone beam computed tomography 3D reconstruction of the mandibular condyle. Angle Orthod. 2008;78(5):880-888. doi: 10.2319/072007-339.1
- van Baar GJC, Forouzanfar T, Liberton NPTJ, Winters HAH, Leusink FKJ. Accuracy of computer-assisted surgery in mandibular reconstruction: a systematic review. Oral Oncol. 2018;84:52-60. doi: 10.1016/j.oraloncology.2018.07.004
- Baltatu MS, Tugui CA, Perju MC, et al. Biocompatible titanium alloys used in medical applications. Rev Chim. 2019;70(4):1302-1306. doi: 10.37358/RC.19.4.7114
- Ran Q, Yang W, Hu Y, et al. Osteogenesis of 3D printed porous Ti6Al4V implants with different pore sizes. J Mech Behav Biomed Mater. 2018;84:1-11. doi: 10.1016/j.jmbbm.2018.04.010
- Taniguchi N, Fujibayashi S, Takemoto M, et al. Effect of pore size on bone ingrowth into porous titanium implants fabricated by additive manufacturing: An in vivo experiment. Mater Sci Eng C Mater Biol Appl. 2016;59:690-701. doi: 10.1016/j.msec.2015.10.069
- Eshkalak SK, Ghomi ER, Dai Y, Choudhury D, Ramakrishna S. The role of three-dimensional printing in healthcare and medicine. Mater Des. 2020;194:108940. doi: 10.1016/j.matdes.2020.108940
- Gao H, Li X, Wang C, Ji P, Wang C. Mechanobiologically optimization of a 3D titanium-mesh implant for mandibular large defect: a simulated study. Mater Sci Eng C Mater Biol Appl. 2019;104:109934. doi: 10.1016/j.msec.2019.109934
- Hosseini S, Hudak R, Penhaker M, Majernik J. Fatigue of Ti- 6Al-4V. In: Biomedical Engineering-Technical Applications in Medicine. London, UK: IntechOpen; 2012:75-92. doi: 10.5772/45753
- Davoodi E, Montazerian H, Esmaeilizadeh R, et al. Additively manufactured gradient porous Ti-6Al-4V hip replacement implants embedded with cell-laden gelatin methacryloyl hydrogels. ACS Appl Mater Interfaces. 2021;13(19):22110-22123. doi: 10.1021/acsami.0c20751
- Farajpour H, Bastami F, Bohlouli M, Khojasteh A. Reconstruction of bilateral ramus-condyle unit defect using custom titanium prosthesis with preservation of both condyles. J Mech Behav Biomed Mater. 2021;124:104765. doi: 10.1016/j.jmbbm.2021.104765
- Luo D, Rong Q, Chen Q. Finite-element design and optimization of a three-dimensional tetrahedral porous titanium scaffold for the reconstruction of mandibular defects. Med Eng Phys. 2017;47:176-183. doi: 10.1016/j.medengphy.2017.06.015
- Liu R, Su Y, Yang W, et al. Novel design and optimization of porous titanium structure for mandibular reconstruction. Appl Bionics Biomech. 2022;2022:8686670. doi: 10.1155/2022/8686670
- Touré G, Gouet E. Use of a 3-dimensional custom-made porous titanium prosthesis for mandibular body reconstruction with prosthetic dental rehabilitation and lipofilling. J Oral Maxillofac Surg. 2019;77(6):1305-1313. doi: 10.1016/j.joms.2018.12.026
- Cheng KJ, Liu YF, Wang R, et al. Topological optimization of 3D printed bone analog with PEKK for surgical mandibular reconstruction. J Mech Behav Biomed Mater. 2020;107:103758. doi: 10.1016/j.jmbbm.2020.103758
- Qassemyar Q, Assouly N, Temam S, Kolb F. Use of a three-dimensional custom-made porous titanium prosthesis for mandibular body reconstruction. Int J Oral Maxillofac Surg. 2017;46(10):1248-1251. doi: 10.1016/j.ijom.2017.06.001
- Ardila CM, Hernández-Arenas Y, Álvarez-Martínez E. Mandibular body reconstruction utilizing a three-dimensional custom-made porous titanium plate: a four-year follow-up clinical report. Case Rep Dent. 2022; 2022:5702066. doi: 10.1155/2022/5702066
- Park JH, Odkhuu M, Cho S, Li J, Park BY, Kim JW. 3D-printed titanium implant with pre-mounted dental implants for mandible reconstruction: a case report. Maxillofac Plast Reconstr Surg. 2020;42(1):28. doi: 10.1186/s40902-020-00272-5
- Raz K, Chval Z, Sedlacek F. Compressive strength prediction of quad-diametral lattice structures. Key Eng Mater. 2020;847:69-74. doi: 10.4028/www.scientific.net/KEM.847.69
- Kim JW, Oh CW, Kim BS, Jeong SL, Jung GH, Lee DH. Structure-mechanical analysis of various fixation constructs for basicervical fractures of the proximal femur and clinical implications; finite element analysis. Injury. 2023;54(2):370-378. doi: 10.1016/j.injury.2022.12.004
- Huang HL, Lin TW, Tsai HL, Wu YL, Wu AYJ. Biomechanical effects of bone atrophy, implant design, and vertical or tilted of posterior implant on all-on-four concept implantation: finite element analysis. J Med Biol Eng. 2022;42(4):488-497. doi: 10.1007/s40846-022-00725-4
- Grant JA, Bishop NE, Götzen N, Sprecher C, Honl M, Morlock MM. Artificial composite bone as a model of human trabecular bone: the implant-bone interface. J Biomech. 2007;40(5):1158-1164. doi: 10.1016/j.jbiomech.2006.04.007
- Bozkaya D, Müftü S. Mechanics of the taper integrated screwed-in (TIS) abutments used in dental implants. J Biomech. 2005;38(1):87-97. doi: 10.1016/j.jbiomech.2004.03.006
- Huang HL, Su KC, Fuh LJ, et al. Biomechanical analysis of a temporomandibular joint condylar prosthesis during various clenching tasks. J Craniomaxillofac Surg. 2015;43(7):1194-1201. doi: 10.1016/j.jcms.2015.04.016
- Korioth TW, Hannam AG. Mandibular forces during simulated tooth clenching. J Orofac Pain. 1994;8(2):178-189.
- Moiduddin K, Anwar S, Ahmed N, Ashfaq M, Al-Ahmari A. Computer assisted design and analysis of customized porous plate for mandibular reconstruction. Irbm. 2017;38(2):78-89. doi: 10.1016/j.irbm.2017.01.003
- Peng WM, Cheng KJ, Liu YF, et al. Biomechanical and mechanostat analysis of a titanium layered porous implant for mandibular reconstruction: the effect of the topology optimization design. Mater Sci Eng C Mater Biol Appl. 2021;124:112056. doi: 10.1016/j.msec.2021.112056
- Liu Y, Wang H, Li S, et al. Compressive and fatigue behavior of beta-type titanium porous structures fabricated by electron beam melting. Acta Mater. 2017;126:58-66. doi: 10.1016/j.actamat.2016.12.052
- Torres-Sanchez C, Al Mushref FRA, Norrito M, Yendall K, Liu Y, Conway PP. The effect of pore size and porosity on mechanical properties and biological response of porous titanium scaffolds. Mater Sci Eng C Mater Biol Appl. 2017;77:219-228. doi: 10.1016/j.msec.2017.03.249
- Arabnejad S, Johnston B, Tanzer M, Pasini D. Fully porous 3D printed titanium femoral stem to reduce stress-shielding following total hip arthroplasty. J Orthop Res. 2017;35(8):1774-1783. doi: 10.1002/jor.23445
- Knoll WD, Gaida A, Maurer P. Analysis of mechanical stress in reconstruction plates for bridging mandibular angle defects. J Craniomaxillofac Surg. 2006;34(4):201-209. doi: 10.1016/j.jcms.2006.01.004
- Afazov S, Denmark WA, Toralles BL, Holloway A, Yaghi A. Distortion prediction and compensation in selective laser melting. Addit Manuf. 2017;17:15-22. doi: 10.1016/j.addma.2017.07.005
- Frost HM. A 2003 update of bone physiology and Wolff ’s Law for clinicians. Angle Orthod. 2004;74(1):3-15. doi: 10.1043/0003-3219(2004)074<0003:AUOBPA>2.0.CO;2
- Biewener AA. Safety factors in bone strength. Calcif Tissue Int. 1993;53(Suppl 1):S68-S74. doi: 10.1007/BF01673406