3D-bioprinted respiratory disease model: Exploring the importance of culture conditions and controlled release in modeling infection
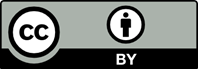
The burden of respiratory illnesses is substantial, significantly impacting healthcare systems worldwide. As researchers work to better understand chronic diseases, as well as newly emerging respiratory viruses, the need for improved respiratory models has become evident. While 3D bioprinting has been illustrated as a feasible method to create complex cellularized constructs or respiratory models, it remains to be determined whether incorporating relevant biomechanical stimuli and/ or relevant growth factors significantly impacts the response of these models to infection. In this study, an alginate/gelatin/collagen solution was synthesized and characterized in terms of rheology, printability, degradation, mechanical properties, and biocompatibility. The bioink, which incorporated primary human pulmonary fibroblasts and THP-1 cells, was bioprinted to form hierarchical 3D constructs and subsequently seeded with primary human bronchial epithelial cells to form the respiratory tissue model. To explore the importance of growth factors and culture conditions in modeling infection, we strategically developed a hepatocyte-growth-factor-loaded nanoparticle system and incorporated them into the bioink for bioprinting the respiratory tissue model, followed by culturing under dynamic conditions in a breath-mimicking bioreactor. The effect of incorporating growth factors and dynamic culture conditions was examined over 28 days, followed by the infection of these constructs with the influenza A virus. It was determined that these constructs support infection, demonstrating a more clinically relevant infection pattern than 2D models. It was further determined that the inclusion of hepatocyte growth factor aids in epithelial cell growth, while the inclusion of biomechanical stimulus increases cellular metabolism and has a moderating effect on response to infection.

- Naghavi M, Antony C, Brauer M, et al. GBD 2019 Chronic Respiratory Diseases Collaborators. Global burden of chronic respiratory diseases and risk factors, 1990–2019: an update from the global burden of disease study 2019. eClinicalMedicine. 2023;59:101936. doi: 10.1016/j.eclinm.2023.101936
- Viegi G, Maoi S, Fasola S, Baldacci S. Global burden of chronic respiratory diseases. J Aerosol Med Pulm Drug Deliv. 2020;33(4):1-38. doi: 10.1089/jamp.2019.1576
- Macias A, McElhaney J, Chaves S, et al. The disease burden of influenza beyond respiratory illness. Vaccine. 2021;39:A6-A14. doi: 10.1016/j.vaccine.2020.09.048
- World Health Organization. Influenza (Seasonal). https://www.who.int/news-room/fact-sheets/detail/influenza- (seasonal). Accessed January 24, 2024
- Yamauchi Y. Chapter one – influenza A virus uncoating. Adv Virus Res. 2020;106:1-38. doi: 10.1016/bs.aivir.2020.01.001
- Suarez D. Influenza A virus. In: Animal Influenza. Ames, Iowa, USA:John Wiley & Sons, Inc.;2017:1-29. doi: 10.1002/9781118924341.ch1
- Julkunen I, Melen K, Nyqvist M, Pirhonen J, Sareneva T, Matikainen S. Inflammatory responses in influenza A virus infection. Vaccine. 2000;19(1):S32-S37. doi: 10.1016/S0264-410X(00)00275-9
- Borau M, Stertz S. Entry of influenza A virus into host cells – recent progress and remaining challenges. Curr Opin Virol. 2021;48:23-29. doi: 10.1016/j.coviro.2021.03.001
- Zhang Y, Xu Z, Cao Y. Host-virus interaction: how host cells defend against influenza A virus infection. Viruses. 2020;12(4):376. doi: 10.3390/v12040376
- Mifsud E, Kuba M, Barr I. Innate immune responses to influenza virus infections in the upper respiratory tract. Viruses. 2021;13(10):2090. doi: 10.3390/v13102090
- Gu Y, Zuo X, Zhang S, et al. The mechanism behind influenza virus cytokine storm. Viruses. 2021;13(7):1362. doi: 10.3390/v13071362
- Julkunen I, Sareneva T, Pirhonen J, Ronni T, Melen K, Matikainen S. Molecular pathogenesis of influenza A virus infection and virus-induced regulation of cytokine gene expression. Cytokine Growth Factor Rev. 2001;12(2-3):171-180. doi: 10.1016/S1359-6101(00)00026-5
- Betakova T, Kostrabova A, Lachova V, Turianova L. Cytokines induced during influenza virus infection. Curr Pharm Des. 2017;23(18):2616-2622. doi: 10.2174/1381612823666170316123736
- Matsushima K, Yang D, Oppenheim J. Interleukin-8: an evolving chemokine. Cytokine. 2022;153:155828. doi: 10.1016/j.cyto.2022.155828
- Can F, Ozkurt Z, Ozturk N, Sezen S. Effect of IL-6, IL-8/CXCL8, IP- 10/CXCL 10 levels on the severity in COVID 19 infection. Int J Clin Pract. 2021;75(12):e14970. doi: 10.1111/ijcp.14970
- Wang J, Oberley-Deegan R, Wang S, et al. Differentiation human alveolar type II cells secrete antiviral IL-29 (IFN-λ1) in response to influenza A infection. J Immunol. 2012;188(5):1924-1932. doi: 10.4049/jimmunol.1101987
- Vastani Z, Ahmadi A, Abounoori M, et al. Interleukin‐29 profiles in COVID‐19 patients: survival is associated with IL‐29 levels. Health Sci Rep. 2022;5(2):e544. doi: 10.1002/hsr2.544
- Niu J, Wu S, Chen M, et al. Hyperactivation of the NLRP3 inflammasome protects mice against influenza A virus infection via IL-1β mediated neutrophil recruitment. Cytokine. 2019;120:115-124. doi: 10.1016/j.cyto.2019.04.019
- Robinson K, Choi S, McHugh K, et al. Influenza A exacerbates staphylococcus aureus pneumonia by attenuating IL-1β production in mice. J Immunol. 2013;191(10):5153-5159. doi: 10.4049/jimmunol.1301237
- Wang W, Yang P, Zhong Y, et al. Monoclonal antibody against CXCL-10/IP-10 ameliorates influenza A (H1N1) virus induced acute lung injury. Cell Res. 2013;23:577-580. doi: 10.1038/cr.2013.25
- Hayney M, Henrique K, Barnet J, et al. Serum IFN-γ-induced protein 10 (IP-10) as a biomarker for severity of acute respiratory infection in healthy adults. J Clin Virol. 2017;90:32-37. doi: 10.1016/j.jcv.2017.03.003
- Zimmerling A, Zhou Y, Chen X. Bioprinted constructs for respiratory tissue engineering. Bioprinting. 2024;24:1-29. doi: 10.1016/j.bprint.2024.100135
- O’Leary C, Gilbert J, O’Dea S, O’Brien F, Cryan S. Respiratory tissue engineering: current status and opportunities for the future. Tissue Eng Part B Rev. 2015;21(4):323-344. doi: 10.1089/ten.TEB.2014.0525
- Shrestha J, Paudel K, Nazari H, et al. Advanced models for respiratory disease and drug studies. Med Res Rev. 2023;43(5):1470-1504. doi: 10.1002/med.21956
- Baroon S, Saez J, Owens R. In vitro models for studying respiratory host–pathogen interactions. Adv Biol. 2021;5(6). doi: 10.1002/adbi.202000624
- Bouvier N, Lowen A. Animal models for influenza virus pathogenesis and transmission. Viruses. 2010;2(8):1530-1563. doi: 10.3390/v20801530
- Nguyen T, Rollon R, Choi Y. Animal models for influenza research: strengths and weaknesses. Viruses. 2021;13(6):1011. doi: 10.3390/v13061011
- Chen X, Anvari-Yazdi A, Duan X, et al. Biomaterials/bioinks and extrusion bioprinting. Bioact Mater. 2023;28:511-536. doi: 10.1016/j.bioactmat.2023.06.006
- Mahfouzi S, Tali S, Amoabediny G. 3D bioprinting for lung and tracheal tissue engineering: criteria, advances, challenges, and future directions. Bioprinting. 2021;21:e00124. doi: 10.1016/j.bprint.2020.e00124
- Carpio M, Dabaghi M, Ungureanu J, Kolb M, Hirota J, Moran- Mirabal J. 3D bioprinting strategies, challenges, and opportunities to model the lung tissue microenvironment and its function. Front Bioeng Biotechnol. 2021;9:773511. doi: 10.3389/fbioe.2021.773511
- Labowska M, Cierluk K, Jankowska A, Kulbacka J, Detyna J, Michalak I. A review on the adaption of alginate-gelatin hydrogels for 3D cultures and bioprinting. Materials. 2021;14(4):85. doi: 10.3390/ma14040858
- Geevarghese R, Somasekharan L, Bhatt A, Kasoju N, Nair R. Development and evaluation of a multicomponent bioink consisting of alginate, gelatin, diethylaminoethyl cellulose and collagen peptide for 3D bioprinting of tissue construct for drug screening application. Int J Biol Macromol. 2022;207:278-288. doi: 10.1016/j.ijbiomac.2022.02.191
- Zimmerling A, Sunil C, Zhou Y, Chen X. Development of a nanoparticle system for controlled release in bioprinted respiratory scaffolds. J Funct Biomater. 2024;15:20. doi: 10.3390/jfb15010020
- Zimmerling A, Boire J, Zhou Y, Chen X. Influence of breath-mimicking ventilated incubation on 3D bioprinted respiratory tissue scaffolds. ASME J Biomech Eng. 2024;146(9):091004. doi: 10.1115/1.4065214
- Butler D, Goldstein S, Guldberg R, et al. The impact of biomechanics in tissue engineering and regenerative medicine. Tissue Eng Part B Rev. 2009;15(4):477-484. doi: 10.1089/ten.teb.2009.0340
- Gresham R, Bahney C, Leach J. Growth factor delivery using extracellular matrix-mimicking substrates for musculoskeletal tissue engineering and repair. Bioact Mater. 2021;6(7):1945-1956. doi: 10.1016/j.bioactmat.2020.12.012
- Nossa R, Costa J, Cacaopardo L, Ahluwalia A. Breathing in vitro: designs and applications of engineered lung models. J Tissue Eng. 2021;12:20417314211008696. doi: 10.1177/20417314211008696
- Huang D, Liu T, Liao J, et al. Reversed-engineered human alveolar lung-on-a-chip model. Proc Natl Acad Sci USA. 2021;118(19):e2016146118. doi: 10.1073/pnas.2016146118
- Kang D, Park J, Kim W, et al. All-inkjet printed 3D alveolar barrier model with physiologically relevant microarchitecture. Adv Sci. 2021;8:2004990. doi: 10.1002/advs.202004990
- Horvath L, Umehara Y, Jud C, Blank F, Petri-Fink A, Rothen- Rutishauser B. Engineering an in vitro air-blood barrier by 3D bioprinting. Sci Rep. 2015;5:7974. doi: 10.1038/srep07974
- Berg J, Hiller T, Kissner M, et al. Optimization of cell-laden bioinks for 3D bioprinting and efficient infection with influenza A virus. Sci Rep. 2018;8:13877. doi: 10.1038/s41598-018-31880-x
- Berg J, Weber Z, Fechler-Bitteti M, et al. Bioprinted multi-cell type lung model for the study of viral inhibitors. Viruses. 2021; 13:1590. doi: 10.3390/v13081590
- Liu G, Park H, Pyo H, Liu Q, Zhou Y. Influenza A virus panhandle structure is directly involved in RIG-I activation and interferon induction. J Virol. 2015;89(11):6067-6079. doi: 10.1128/JVI.00232-15
- Panganiban R, Day R. Hepatocyte growth factor in lung repair and pulmonary fibrosis. Acta Pharmacol Sin. 2010;32:12-20. doi: 10.1038/aps.2010.90
- Zimmerling A, Zhou Y, Chen X. Synthesis of alginate/collagen bioinks for bioprinting respiratory tissue models. J Funct Biomater. 2024;15:90. doi: 10.3390/jfb15040090
- Malekpour A, Chen X. Printability and cell viability in extrusion-based bioprinting from experimental, computational, and machine learning views, J Funct Biomater. 2022;13(2):40. doi: 10.3390/jfb13020040
- Fu ZQ, Naghieh S, Xu CC, Wang CJ, Sun W, Chen XB. Printability in extrusion bioprinting. Biofabrication. 2021; 13(3):033001. doi: 10.1088/1758-5090/abe7ab
- Sousa dos Santos K, Oliveira L, de Lima Fontes M. et al. Alginate-based 3D A549 cell culture model to study paracoccidioides infection. J. Fungi. 2023;9(6):634. doi: 10.3390/jof9060634
- Zhang J, Zhang W, Ren L, et al. Astragaloside IV attenuates IL-1β secretion by enhancing autophagy in H1N1 infection. Microbiol Letters. 2020;367(4):fnaa007. doi: 10.1093/femsle/fnaa007
- Gao J, Gao L, Li R, Lai Z, Zhang Z, Fan X. Integrated analysis of microRNA-nRNA expression in A549 cells infected with influenza A viruses (IAVs) from different host species. Virus Res. 2019;263:34-46. doi: 10.1016/j.virusres.2018.12.016
- Mondrinos M, Lelkes P, Samadikuchaksaraei A, Mantalaris A, Polak J. Lungs. In: Principles of Tissue Engineering (Fourth Edition). San Diego, USA: Academic Press/Elsevier;2014:1560-1577 doi: 10.1016/B978-0-12-398358-9.00074-4
- Kim W, Lee Y, Kang D, Kwak T, Lee H, Jung S. 3D inkjet-bioprinted lung-on-a-chip. ACS Biomater Sci Eng. 2023;9:2806-2815. doi: 10.1021/acsbiomaterials.3c00089