Photoacoustic imaging for three-dimensional bioprinted constructs
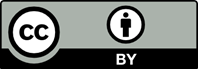
Bioimaging is used to inspect the successful growth and functional differentiation of cells in printed biomaterials, which are ultimately finalized into functional artificial tissues capable of replacing native tissues. While optical bioimaging techniques are commonly utilized, the current trend in three-dimensional (3D) bioprinting towards replicating complex 3D microarchitectures poses a challenge for conventional optical imaging techniques in providing clear cross-sectional images due to the opaque nature of tissue. Consequently, these limitations necessitate lengthy and destructive preparation processes, which are associated with sacrificing cell viability and damaging the bioprinted material. Photoacoustic imaging (PAI) is a versatile imaging technique that extends the advantages of the optical bioimaging technique to undiscovered depths enabled by its acoustic hybridity, making itself a promising tool for non-destructive imaging of 3D bioprinted constructs. In this review, we introduce the flexible spectral contrasts provided by PAI, which are potentially applicable to 3D-bioprinted constructs, and summarize bioprinting studies that functionally implement PAI for in vitro and in vivo assessments. Finally, we provide an outlook on practical considerations for the more complete integration of these two fields, anticipating more fruitful discoveries as bioprinting advances towards more complex hierarchies.
- Ashammakhi N, Ahadian S, Xu C, et al. Bioinks and bioprinting technologies to make heterogeneous and biomimetic tissue constructs. Mater Today Bio. 2019;1:100008. doi: 10.1016/j.mtbio.2019.100008
- Jo Y, Hwang DG, Kim M, Yong U, Jang J. Bioprinting-assisted tissue assembly to generate organ substitutes at scale. Trends Biotechnol. 2023;41(1):93-105. doi: 10.1016/j.tibtech.2022.07.001
- Cho S, Jang J. Recent trends in biofabrication technologies for studying skeletal muscle tissue-related diseases. Front Bioeng Biotechnol. 2021;9:782333. doi: 10.3389/fbioe.2021.782333
- Kim D, Kim M, Lee J, Jang J. Review on multicomponent hydrogel bioinks based on natural biomaterials for bioprinting 3D liver tissues. Front Bioeng Biotechnol. 2022;10:764682. doi: 10.3389/fbioe.2022.764682
- Gao G, Kim BS, Jang J, Cho D-W. Recent strategies in extrusion-based three-dimensional cell printing toward organ biofabrication. ACS Biomater Sci Eng. 2019;5(3): 1150-1169. doi: 10.1021/acsbiomaterials.8b00691
- Das S, Nam H, Jang J. 3D bioprinting of stem cell-laden cardiac patch: a promising alternative for myocardial repair. APL Bioeng. 2021;5(3):031508. doi: 10.1063/5.0030353
- Jang J, Park JY, Gao G, Cho D-W. Biomaterials-based 3D cell printing for next-generation therapeutics and diagnostics. Biomaterials. 2018;156:88-106. doi: 10.1016/j.biomaterials.2017.11.030
- Jang J, Yi H-G, Cho D-W. 3D printed tissue models: present and future. ACS Biomater Sci Eng. 2016;2(10):1722-1731. doi: 10.1021/acsbiomaterials.6b00129
- Kim D, Kang D, Kim D, Jang J. Volumetric bioprinting strategies for creating large-scale tissues and organs. MRS Bull. 2023;48(6):657-667. doi: 10.1557/s43577-023-00541-4
- Yong U, Kim D, Kim H, et al. Biohybrid 3D printing of a tissue‐sensor platform for wireless, real‐time, and continuous monitoring of drug‐induced cardiotoxicity. Adv Mater. 2023;35(11):e2208983. doi: 10.1002/adma.202208983
- Yoon J, Singh NK, Jang J, Cho D-W. 3D bioprinted in vitro secondary hyperoxaluria model by mimicking intestinal-oxalate-malabsorption-related kidney stone disease. Appl Phys Rev. 2022;9(4):041408. doi: 10.1063/5.0087345
- Hwang DG, Jo Y, Kim M, et al. A 3D bioprinted hybrid encapsulation system for delivery of human pluripotent stem cell-derived pancreatic islet-like aggregates. Biofabrication. 2021;14(1):014101. doi: 10.1088/1758-5090/ac23ac
- Kim BS, Ahn M, Cho W-W, Gao G, Jang J, Cho D-W. Engineering of diseased human skin equivalent using 3D cell printing for representing pathophysiological hallmarks of type 2 diabetes in vitro. Biomaterials. 2021;272:120776. doi: 10.1016/j.biomaterials.2021.120776
- Kim M, Jang J. Construction of 3D hierarchical tissue platforms for modeling diabetes. APL Bioeng. 2021;5(4):041506. doi: 10.1063/5.0055128
- Jang J, Park H-J, Kim S-W, et al. 3D printed complex tissue construct using stem cell-laden decellularized extracellular matrix bioinks for cardiac repair. Biomaterials. 2017;112: 264-274. doi: 10.1016/j.biomaterials.2016.10.026
- Choi Y-m, Lee H, Ann M, Song M, Rheey J, Jang J. 3D bioprinted vascularized lung cancer organoid models with underlying disease capable of more precise drug evaluation. Biofabrication. 2023;15(3):034104. doi: 10.1088/1758-5090/acd95f
- Kim JJ, Park JY, Nguyen VVT, et al. Pathophysiological reconstruction of a tissue‐specific multiple‐organ on‐a‐chip for Type 2 diabetes emulation using 3D cell printing. Adv Funct Mater. 2023;33(22):2213649. doi: 10.1002/adfm.202213649
- Choi S, Lee KY, Kim SL, et al. Fibre-infused gel scaffolds guide cardiomyocyte alignment in 3D-printed ventricles. Nat Mater. 2023;22(8):1039-1046. doi: 10.1038/s41563-023-01611-3
- Hwang DG, Choi H, Yong U, et al. Bioprinting‐assisted tissue assembly for structural and functional modulation of engineered heart tissue mimicking left ventricular myocardial fiber orientation. Adv Mater. 2024:e2400364. doi: 10.1002/adma.202400364
- An J, Zhang S, Wu J, et al. Assessing bioartificial organ function: the 3P model framework and its validation. Lab Chip. 2024;24(10). doi: 10.1039/d3lc01020a
- Mirdamadi E, Tashman JW, Shiwarski DJ, Palchesko RN, Feinberg AW. FRESH 3D bioprinting a full-size model of the human heart. ACS Biomater Sci Eng. 2020;6(11):6453-6459. doi: 10.1021/acsbiomaterials.0c01133
- Kolesky DB, Truby RL, Gladman AS, Busbee TA, Homan KA, Lewis JA. 3D bioprinting of vascularized, heterogeneous cell‐laden tissue constructs. Adv Mater. 2014;26(19): 3124-3130. doi: 10.1002/adma.201305506
- Colosi C, Shin SR, Manoharan V, et al. Microfluidic bioprinting of heterogeneous 3D tissue constructs using low‐viscosity bioink. Adv Mater. 2016;28(4):677-684. doi: 10.1002/adma.201503310
- Glaser AK, Reder NP, Chen Y, et al. Light-sheet microscopy for slide-free non-destructive pathology of large clinical specimens. Nat Biomed Eng. 2017;1(7):0084. doi: 10.1038/s41551-017-0084
- De Santis MM, Alsafadi HN, Tas S, et al. Extracellular‐matrix‐reinforced bioinks for 3D bioprinting human tissue. Adv Mater. 2021;33(3):e2005476. doi: 10.1002/adma.202005476
- Hafa L, Breideband L, Ramirez Posada L, et al. Light sheet‐based laser patterning bioprinting produces long‐term viable full‐thickness skin constructs. Adv Mater. 2024;36(8):e2306258. doi: 10.1002/adma.202306258
- Ouyang L, Armstrong JP, Chen Q, Lin Y, Stevens MM. Void‐free 3D bioprinting for in situ endothelialization and microfluidic perfusion. Adv Funct Mater. 2019;30(1):1908349. doi: 10.1002/adfm.201908349
- Chen C-W, Betz MW, Fisher JP, Paek A, Chen Y. Macroporous hydrogel scaffolds and their characterization by optical coherence tomography. Tissue Eng Part C Methods. 2011;17(1):101-112. doi: 10.1089/ten.tec.2010.0072
- Tashman JW, Shiwarski DJ, Coffin B, et al. In situ volumetric imaging and analysis of FRESH 3D bioprinted constructs using optical coherence tomography. Biofabrication. 2022;15(1):014102. doi: 10.1088/1758-5090/ac975e
- Attia ABE, Balasundaram G, Moothanchery M, et al. A review of clinical photoacoustic imaging: current and future trends. Photoacoustics. 2019;16:100144. doi: 10.1016/j.pacs.2019.100144
- Karlas A, Fasoula N-A, Paul-Yuan K, et al. Cardiovascular optoacoustics: from mice to men–a review. Photoacoustics. 2019;14:19-30. doi: 10.1016/j.pacs.2019.03.001
- Manohar S, Dantuma M. Current and future trends in photoacoustic breast imaging. Photoacoustics. 2019;16:100134. doi: 10.1016/j.pacs.2019.04.004
- Jeon S, Kim J, Lee D, Baik JW, Kim C. Review on practical photoacoustic microscopy. Photoacoustics. 2019;15:100141. doi: 10.1016/j.pacs.2019.100141
- Choi W, Oh D, Kim C. Practical photoacoustic tomography: realistic limitations and technical solutions. J Appl Phys. 2020;127(23):230903. doi: 10.1063/5.0008401
- Park B, Oh D, Kim J, Kim C. Functional photoacoustic imaging: from nano-and micro-to macro-scale. Nano Converg. 2023;10(1):29. doi: 10.1186/s40580-023-00377-3
- Yao J, Wang LV. Sensitivity of photoacoustic microscopy. Photoacoustics. 2014;2(2):87-101. doi: 10.1016/j.pacs.2014.04.002
- Park J, Park B, Kim TY, et al. Quadruple ultrasound, photoacoustic, optical coherence, and fluorescence fusion imaging with a transparent ultrasound transducer. Proc Natl Acad Sci U S A. 2021;118(11):e1920879118. doi: 10.1073/pnas.1920879118
- Choi S, Yang J, Lee SY, et al. Deep learning enhances multiparametric dynamic volumetric photoacoustic computed tomography in vivo (DL‐PACT). Adv Sci (Weinh). 2022;10(1):e2202089. doi: 10.1002/advs.202202089
- Park B, Park S, Kim J, Kim C. Listening to drug delivery and responses via photoacoustic imaging. Adv Drug Deliv Rev. 2022;184:114235. doi: 10.1016/j.addr.2022.114235
- Choi W, Park B, Choi S, Oh D, Kim J, Kim C. Recent advances in contrast-enhanced photoacoustic imaging: overcoming the physical and practical challenges. Chem Rev. 2023;123(11):7379-7419. doi: 10.1021/acs.chemrev.2c00627
- Cho S, Baik J, Managuli R, Kim C. 3D PHOVIS: 3D photoacoustic visualization studio. Photoacoustics. 2020;18:100168. doi: 10.1016/j.pacs.2020.100168
- Choi S, Park S, Kim J, et al. X-ray free-electron laser induced acoustic microscopy (XFELAM). Photoacoustics. 2024;35(4):100587. doi: 10.1016/j.pacs.2024.100587
- Oh D, Lee D, Heo J, et al. Contrast agent‐free 3D Renal ultrafast doppler imaging reveals vascular dysfunction in acute and diabetic kidney diseases. Adv Sci (Weinh). 2023;10(36):e2303966. doi: 10.1002/advs.202303966
- Oh D, Kim H, Sung M, Kim C. Video-rate endocavity photoacoustic/harmonic ultrasound imaging with miniaturized light delivery. J Biomed Opt. 2024;29(S1):S11528. doi: 10.1117/1.jbo.29.s1.s11528
- Yoo J, Oh D, Kim C, Kim HH, Um J-Y. Switchable preamplifier for dual modal photoacoustic and ultrasound imaging. Biomed Opt Express. 2023;14(1):89-105. doi: 10.1364/boe.476453
- Kim H, Cho S, Park E, et al. Nonlinear beamforming for intracardiac echocardiography: a comparative study. Biomed Eng Lett. 2024;14(3):571-582. doi: 10.1007/s13534-024-00352-9
- Baik JW, Kim JY, Cho S, Choi S, Kim J, Kim C. Super wide-field photoacoustic microscopy of animals and humans in vivo. IEEE Trans Med Imaging. 2020;39(4):975-984. doi: 10.1109/tmi.2019.2938518
- Ahn J, Baik JW, Kim D, et al. In vivo photoacoustic monitoring of vasoconstriction induced by acute hyperglycemia. Photoacoustics. 2023;30:100485. doi: 10.1016/j.pacs.2023.100485
- Kim D, Ahn J, Park E, Kim JY, Kim C. In vivo quantitative photoacoustic monitoring of corticosteroid-induced vasoconstriction. J Biomed Opt. 2023;28(8):082805. doi: 10.1117/1.JBO.28.8.082805
- Lee H, Park SM, Park J, et al. Transportable multispectral optical-resolution photoacoustic microscopy using stimulated raman scattering spectrum. IEEE Trans Instrum Measure. 2024. doi: 10.1109/tim.2024.3351259
- Park B, Lee KM, Park S, et al. Deep tissue photoacoustic imaging of nickel (II) dithiolene-containing polymeric nanoparticles in the second near-infrared window. Theranostics. 2020;10(6):2509-2521. doi: 10.7150/thno.39403
- Li X, Park EY, Kang Y, et al. Supramolecular phthalocyanine assemblies for improved photoacoustic imaging and photothermal therapy. Angew Chemie. 2020;132(22): 8708-8712. doi: 10.1002/ange.201916147
- Park E-Y, Oh D, Park S, Kim W, Kim C. New contrast agents for photoacoustic imaging and theranostics: recent 5-year overview on phthalocyanine/naphthalocyanine-based nanoparticles. APL Bioeng. 2021;5(3):031510. doi: 10.1063/5.0047660
- Ding Y, Park B, Ye J, et al. Surfactant‐stripped semiconducting polymer micelles for tumor theranostics and deep tissue imaging in the NIR‐II window. Small. 2022;18(6):e2104132. doi: 10.1002/smll.202104132
- Park J, Park B, Yong U, et al. Bi-modal near-infrared fluorescence and ultrasound imaging via a transparent ultrasound transducer for sentinel lymph node localization. Opt Lett. 2022;47(2):393-396. doi: 10.1364/ol.446041
- Maji D, Oh D, Sharmah Gautam K, et al. Copper‐catalyzed covalent dimerization of near‐infrared fluorescent cyanine dyes: synergistic enhancement of photoacoustic signals for molecular imaging of tumors. Anal Sens. 2022;2(1):e202100045. doi: 10.1002/anse.202100045
- Noh I, Kim M, Kim J, et al. Structure-inherent near-infrared bilayer nanovesicles for use as photoacoustic image-guided chemo-thermotherapy. J Control Release. 2020;320:283-292. doi: 10.1016/j.jconrel.2020.01.032
- Fasoula N-A, Karlas A, Prokopchuk O, et al. Non-invasive multispectral optoacoustic tomography resolves intrahepatic lipids in patients with hepatic steatosis. Photoacoustics. 2023;29:100454. doi: 10.1016/j.pacs.2023.100454
- Choi W, Park E-Y, Jeon S, et al. Three-dimensional multistructural quantitative photoacoustic and US imaging of human feet in vivo. Radiology. 2022;303(2):467-473. doi: 10.1148/radiol.211029
- Kim J, Park B, Ha J, et al. Multiparametric photoacoustic analysis of human thyroid cancers in vivo. Cancer Res. 2021;81(18):4849-4860. doi: 10.1158/0008-5472.can-20-3334
- Yao D-K, Maslov K, Shung KK, Zhou Q, Wang LV. In vivo label-free photoacoustic microscopy of cell nuclei by excitation of DNA and RNA. Opt Lett. 2010;35(24): 4139-4141. doi: 10.1364/OL.35.004139
- Wong TT, Zhang R, Zhang C, et al. Label-free automated three-dimensional imaging of whole organs by microtomy-assisted photoacoustic microscopy. Nat Commun. 2017;8(1):1386. doi: 10.1038/s41467-017-01649-3
- Wong TT, Zhang R, Hai P, et al. Fast label-free multilayered histology-like imaging of human breast cancer by photoacoustic microscopy. Sci Adv. 2017;3(5):e1602168. doi: 10.1126/sciadv.1602168
- Baik JW, Kim H, Son M, et al. Intraoperative label‐free photoacoustic histopathology of clinical specimens. Laser Photonics Rev. 2021;15(10):2100124. doi: 10.1002/lpor.202170052
- Martell MT, Haven NJ, Cikaluk BD, et al. Deep learning-enabled realistic virtual histology with ultraviolet photoacoustic remote sensing microscopy. Nat Commun. 2023;14(1):5967. doi: 10.1038/s41467-023-41574-2
- Cao R, Nelson SD, Davis S, et al. Label-free intraoperative histology of bone tissue via deep-learning-assisted ultraviolet photoacoustic microscopy. Nat Biomed Eng. 2023;7(2):124-134. doi: 10.1038/s41551-022-00940-z
- Kang L, Li X, Zhang Y, Wong TT. Deep learning enables ultraviolet photoacoustic microscopy based histological imaging with near real-time virtual staining. Photoacoustics. 2021;25:100308. doi: 10.1016/j.pacs.2021.100308
- Cao R, Zhao J, Li L, et al. Optical-resolution photoacoustic microscopy with a needle-shaped beam. Nat Photon. 2023;17(1):89-95. doi: 10.1038/s41566-022-01112-w
- Kim D, Park E, Park J, et al. An ultraviolet‐transparent ultrasound transducer enables high‐resolution label‐free photoacoustic histopathology. Laser Photon Rev. 2024;18(2):2300652. doi: 10.1002/lpor.202470012
- Zhu X, Huang Q, DiSpirito A, et al. Real-time whole-brain imaging of hemodynamics and oxygenation at micro-vessel resolution with ultrafast wide-field photoacoustic microscopy. Light Sci Appl. 2022;11(1):138. doi: 10.1038/s41377-022-00836-2
- Zhu X, Huang Q, Jiang L, et al. Longitudinal intravital imaging of mouse placenta. Sci Adv. 2024;10(12):eadk1278. doi: 10.1126/sciadv.adk1278
- Gatford KL, Andraweera PH, Roberts CT, Care AS. Animal models of preeclampsia: causes, consequences, and interventions. Hypertension. 2020;75(6):1363-1381. doi: 10.1161/hypertensionaha.119.14598
- Hemberger M, Hanna CW, Dean W. Mechanisms of early placental development in mouse and humans. Nat Rev Genet. 2020;21(1):27-43. doi: 10.1038/s41576-019-0169-4
- Dekan S, Linduska N, Kasprian G, Prayer D. MRI of the placenta–a short review. Wien Med Wochenschr. 2012;162(9):225-228. doi: 10.1007/s10354-012-0073-4
- Rebling J, Ben‐Yehuda Greenwald M, Wietecha M, Werner S, Razansky D. Long‐term imaging of wound angiogenesis with large scale optoacoustic microscopy. Adv Sci. 2021;8(13):2004226. doi: 10.1002/advs.202004226
- Liu C, Liang Y, Wang L. Single-shot photoacoustic microscopy of hemoglobin concentration, oxygen saturation, and blood flow in sub-microseconds. Photoacoustics. 2020;17:100156. doi: 10.1016/j.pacs.2019.100156
- Pleitez MA, Khan AA, Soldà A, et al. Label-free metabolic imaging by mid-infrared optoacoustic microscopy in living cells. Nat Biotechnol. 2020;38(3):293-296. doi: 10.1038/s41587-019-0359-9
- Shi J, Wong TT, He Y, et al. High-resolution, high-contrast mid-infrared imaging of fresh biological samples with ultraviolet-localized photoacoustic microscopy. Nat Photonics. 2019;13(9):609-615. doi: 10.1038/s41566-019-0441-3
- Park E, Lee Y-J, Kim C, Eom TJ. Azimuth mapping of fibrous tissue in linear dichroism-sensitive photoacoustic microscopy. Photoacoustics. 2023;31:100510. doi: 10.1016/j.pacs.2023.100510
- Zhao W, Yu H, Ge Z, et al. Characterization of interconnectivity of gelatin methacrylate hydrogels using photoacoustic imaging. Lab Chip. 2022;22(4):727-732. doi: 10.1039/d1lc00967b
- Ma C, Li W, Li D, et al. Photoacoustic imaging of 3D-printed vascular networks. Biofabrication. 2022;14(2):025001. doi: 10.1088/1758-5090/ac49d5
- Yim W, Zhou J, Sasi L, et al. 3D‐bioprinted phantom with human skin phototypes for biomedical optics. Adv Mater. 2023;35(3):e2206385. doi: 10.1002/adma.202305227
- Luo Y, Wei X, Wan Y, Lin X, Wang Z, Huang P. 3D printing of hydrogel scaffolds for future application in photothermal therapy of breast cancer and tissue repair. Acta Biomater. 2019;92:37-47. doi: 10.1016/j.actbio.2019.05.039
- Zhu W, Zhou Z, Huang Y, et al. A versatile 3D-printable hydrogel for antichondrosarcoma, antibacterial, and tissue repair. J Mater Sci Technol. 2023;136:200-211. doi: 10.1016/j.jmst.2022.07.010
- Wei X, Liu C, Wang Z, Luo Y. 3D printed core-shell hydrogel fiber scaffolds with NIR-triggered drug release for localized therapy of breast cancer. Int J Pharm. 2020;580:119219. doi: 10.1016/j.ijpharm.2020.119219
- Yang C, Gao X, Younis MR, et al. Non-invasive monitoring of in vivo bone regeneration based on alkaline phosphatase-responsive scaffolds. Chem Eng J. 2021;408:127959. doi: 10.1016/j.cej.2020.127959
- Zhang Y, Cai X, Choi S-W, Kim C, Wang LV, Xia Y. Chronic label-free volumetric photoacoustic microscopy of melanoma cells in three-dimensional porous scaffolds. Biomaterials. 2010;31(33):8651-8658. doi: 10.1016/j.biomaterials.2010.07.089
- Zhang YS, Cai X, Yao J, Xing W, Wang LV, Xia Y. Non‐invasive and in situ characterization of the degradation of biomaterial scaffolds by volumetric photoacoustic microscopy. Angew Chemie Int Ed. 2014;53(1):184-188. doi: 10.1002/anie.201306282
- Cai X, Zhang Y, Li L, et al. Investigation of neovascularization in three-dimensional porous scaffolds in vivo by a combination of multiscale photoacoustic microscopy and optical coherence tomography. Tissue Eng Part C Methods. 2013;19(3):196-204. doi: 10.1089/ten.tec.2012.0326
- Cai X, Paratala BS, Hu S, Sitharaman B, Wang LV. Multiscale photoacoustic microscopy of single-walled carbon nanotube-incorporated tissue engineering scaffolds. Tissue Eng Part C Methods. 2012;18(4):310-317. doi: 10.1089/ten.tec.2011.0519
- Zheng N, Fitzpatrick V, Cheng R, Shi L, Kaplan DL, Yang C. Photoacoustic carbon nanotubes embedded silk scaffolds for neural stimulation and regeneration. ACS Nano. 2022;16(2):2292-2305. doi: 10.1021/acsnano.1c08491
- Ogunlade O, Ho JO, Kalber TL, et al. Monitoring neovascularization and integration of decellularized human scaffolds using photoacoustic imaging. Photoacoustics. 2019;13:76-84. doi: 10.1016/j.pacs.2019.01.001
- Hwang SH, Kim J, Heo C, et al. 3D printed multi-growth factor delivery patches fabricated using dual-crosslinked decellularized extracellular matrix-based hybrid inks to promote cerebral angiogenesis. Acta Biomater. 2023;157:137-148. doi: 10.1016/j.actbio.2022.11.050
- Li C, Ma Z, Li W, et al. 3D-printed scaffolds promote angiogenesis by recruiting antigen-specific T cells. Engineering. 2022;17:183-195. doi: 10.1016/j.eng.2021.05.018
- Tosoratti E, Fisch P, Taylor S, Laurent‐Applegate LA, Zenobi‐Wong M. 3D‐printed reinforcement scaffolds with targeted biodegradation properties for the tissue engineering of articular cartilage. Adv Healthc Mater. 2021;10(23):e2101094. doi: 10.1002/adhm.202101094
- Hajireza P, Shi W, Bell K, Paproski RJ, Zemp RJ. Non-interferometric photoacoustic remote sensing microscopy. Light Sci Appl. 2017;6(6):e16278. doi: 10.1038/lsa.2016.278
- Sun W, Starly B, Daly AC, et al. The bioprinting roadmap. Biofabrication. 2020;12(2):022002. doi: 10.1088/1758-5090/ab5158
- Gao G, Lee JH, Jang J, et al. Tissue engineered bio‐blood‐vessels constructed using a tissue‐specific bioink and 3D coaxial cell printing technique: a novel therapy for ischemic disease. Adv Funct Mater. 2017;27(33):1700798. doi: 10.1002/adfm.201770192