The promising applications of 3D printing technology in neurotrauma
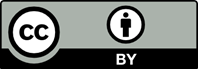
Neurotrauma mainly includes brain injury, spinal cord injury, and peripheral nerve injury, which are characterized by high morbidity and disability rates, and involve costly treatments. Currently, various strategies have been applied for the treatment of neurotrauma, but their efficacy is unsatisfactory. New effective strategies are needed to be developed to promote recovery after neurotrauma. In recent years, three-dimensional (3D) printing technology has been used to manufacture customized and complex constructs in tissue engineering applications, exhibiting great potential in repairing nervous system injuries. In this review, we introduce the principles and advantages of 3D printing and 3D bioprinting technologies that have been applied to repair injured nervous system. In particular, we summarize the current strategies in the aspects of biomaterials, physical stimulation, bioactive substances, cell transplantation, and their combination that have been considered in fabricating 3D-printed devices for neurotrauma treatment. Additionally, the challenges and prospects of 3D printing for neurotrauma treatment were also presented.
- Peppiatt CM, Howarth C, Mobbs P, Attwell D. Bidirectional control of CNS capillary diameter by pericytes. Nature. 2006;443(7112):700-704. doi: 10.1038/nature05193
- Bican O, Minagar A, Pruitt AA. The spinal cord: a review of functional neuroanatomy. Neurol Clin. 2013;31(1):1-18. doi: 10.1016/j.ncl.2012.09.009
- Catala M, Kubis N. Gross anatomy and development of the peripheral nervous system. Handb Clin Neurol. 2013; 115:29-41. doi: 10.1016/b978-0-444-52902-2.00003-5
- Suter T, Jaworski A. Cell migration and axon guidance at the border between central and peripheral nervous system. Science (New York, NY). 2019;365(6456). doi: 10.1126/science.aaw8231
- Lozano JCM, Gomez RM, Sanabria AGZ, Jubiz G, Valderrama S. Genetic aspects of glial cells regarding neurodegenerative diseases. Curr Pharm Des. 2018;24(15):1727-1735. doi: 10.2174/1381612823666170828134055
- Shafiee A, Ahmadi H, Taheri B, et al. Appropriate scaffold selection for CNS tissue engineering. Avicenna J Med Biotechnol. 2020;12(4):203-220.
- Alilain WJ, Horn KP, Hu H, Dick TE, Silver J. Functional regeneration of respiratory pathways after spinal cord injury. Nature. 2011;475(7355):196-200. doi: 10.1038/nature10199
- Fawcett JW, Oohashi T, Pizzorusso T. The roles of perineuronal nets and the perinodal extracellular matrix in neuronal function. Nat Rev Neurosci. 2019;20(8):451-465. doi: 10.1038/s41583-019-0196-3
- Reichardt LF, Tomaselli KJ. Extracellular matrix molecules and their receptors: functions in neural development. Annu Rev Neurosci. 1991;14:531-570. doi: 10.1146/annurev.ne.14.030191.002531
- Menon DK, Schwab K, Wright DW, Maas AI. Position statement: definition of traumatic brain injury. Arch Phys Med Rehabil. 2010;91(11):1637-1640. doi: 10.1016/j.apmr.2010.05.017
- Maas AIR, Menon DK, Adelson PD, et al. Traumatic brain injury: integrated approaches to improve prevention, clinical care, and research. Lancet Neurol. 2017;16(12):987-1048. doi: 10.1016/s1474-4422(17)30371-x
- Wiles MD. Management of traumatic brain injury: a narrative review of current evidence. Anaesthesia. 2022; 77(Suppl 1):102-112. doi: 10.1111/anae.15608
- Zhang J, Liu X, Ma K, et al. Collagen/heparin scaffold combined with vascular endothelial growth factor promotes the repair of neurological function in rats with traumatic brain injury. Biomater Sci. 2021;9(3):745-764. doi: 10.1039/c9bm01446b
- Mukherjee N, Adak A, Ghosh S. Recent trends in the development of peptide and protein-based hydrogel therapeutics for the healing of CNS injury. Soft Matter. 2020;16(44):10046-10064. doi: 10.1039/d0sm00885k
- Mahumane GD, Kumar P, Pillay V, Choonara YE. Repositioning N-acetylcysteine (NAC): NAC-loaded electrospun drug delivery scaffolding for potential neural tissue engineering application. Pharmaceutics. 2020;12(10). doi: 10.3390/pharmaceutics12100934
- Spinal cord injury (SCI) 2016 facts and figures at a glance. J Spinal Cord Med. 2016;39(4):493-494. doi: 10.1080/10790268.2016.1210925
- Zarepour A, Hooshmand S, Gökmen A, Zarrabi A, Mostafavi E. Spinal cord injury management through the combination of stem cells and implantable 3D bioprinted platforms. Cells. 2021;10(11). doi: 10.3390/cells10113189
- Bedir T, Ulag S, Ustundag CB, Gunduz O. 3D bioprinting applications in neural tissue engineering for spinal cord injury repair. Mater Sci Eng C Mater Biol Appl. 2020;110:110741. doi: 10.1016/j.msec.2020.110741
- Fehlings MG, Tetreault LA, Wilson JR, et al. A clinical practice guideline for the management of acute spinal cord injury: introduction, rationale, and scope. Global Spine J. 2017;7(3 Suppl):84s-94s. doi: 10.1177/2192568217703387
- Carvalho CR, Reis RL, Oliveira JM. Fundamentals and current strategies for peripheral nerve repair and regeneration. Adv Exp Med Biol. 2020;1249:173-201. doi: 10.1007/978-981-15-3258-0_12
- Kehoe S, Zhang XF, Boyd D. FDA approved guidance conduits and wraps for peripheral nerve injury: a review of materials and efficacy. Injury. 2012;43(5):553-572. doi: 10.1016/j.injury.2010.12.030
- Petcu EB, Midha R, McColl E, Popa-Wagner A, Chirila TV, Dalton PD. 3D printing strategies for peripheral nerve regeneration. Biofabrication. 2018;10(3):032001. doi: 10.1088/1758-5090/aaaf50
- Barnes DM. What makes nerves regenerate? Science (New York, NY). 1985;230(4729):1024-1025. doi: 10.1126/science.4059920
- Horner PJ, Gage FH. Regenerating the damaged central nervous system. Nature. 2000;407(6807):963-970. doi: 10.1038/35039559
- Scheib J, Höke A. Advances in peripheral nerve regeneration. Nat Rev Neurol. 2013;9(12):668-676. doi: 10.1038/nrneurol.2013.227
- Liu B, Xin W, Tan JR, et al. Myelin sheath structure and regeneration in peripheral nerve injury repair. Proc Natl Acad Sci U S A. 2019;116(44):22347-22352. doi: 10.1073/pnas.1910292116
- Bridging the gap. Science. 2008;320(5878):851. doi: 10.1126/science.320.5878.851b
- Vijayavenkataraman S. Nerve guide conduits for peripheral nerve injury repair: a review on design, materials and fabrication methods. Acta Biomater. 2020;106:54-69. doi: 10.1016/j.actbio.2020.02.003
- Deumens R, Bozkurt A, Meek MF, et al. Repairing injured peripheral nerves: bridging the gap. Prog Neurobiol. 2010;92(3):245-276. doi: 10.1016/j.pneurobio.2010.10.002
- Parker BJ, Rhodes DI, O’Brien CM, Rodda AE, Cameron NR. Nerve guidance conduit development for primary treatment of peripheral nerve transection injuries: a commercial perspective. Acta Biomater. 2021;135:64-86. doi: 10.1016/j.actbio.2021.08.052
- Islamov R, Bashirov F, Fadeev F, et al. Epidural stimulation combined with triple gene therapy for spinal cord injury treatment. Int J Mol Sci. 2020;21(23). doi: 10.3390/ijms21238896
- Ford S, Despeisse M. Additive manufacturing and sustainability: an exploratory study of the advantages and challenges. J Clean Prod. 2016;137:1573-1587. doi: 10.1016/j.jclepro.2016.04.150
- Wang X, Jiang M, Zhou Z, Gou J, Hui D. 3D printing of polymer matrix composites: a review and prospective. Compos B Eng. 2017;110:442-458. doi: 10.1016/j.compositesb.2016.11.034
- Joung D, Lavoie NS, Guo SZ, Park SH, Parr AM, McAlpine MC. 3D printed neural regeneration devices. Adv Funct Mater. 2020;30(1). doi: 10.1002/adfm.201906237
- Haring AP, Sontheimer H, Johnson BN. Microphysiological human brain and neural systems-on-a-chip: potential alternatives to small animal models and emerging platforms for drug discovery and personalized medicine. Stem Cell Rev Rep. 2017;13(3):381-406. doi: 10.1007/s12015-017-9738-0
- Matai I, Kaur G, Seyedsalehi A, McClinton A, Laurencin CT. Progress in 3D bioprinting technology for tissue/organ regenerative engineering. Biomaterials. 2020;226:119536. doi: 10.1016/j.biomaterials.2019.119536
- Li Y, Cheng S, Wen H, et al. Coaxial 3D printing of hierarchical structured hydrogel scaffolds for on-demand repair of spinal cord injury. Acta Biomater. 2023;168:400-415. doi: 10.1016/j.actbio.2023.07.020
- Joung D, Truong V, Neitzke CC, et al. 3D printed stem-cell derived neural progenitors generate spinal cord scaffolds. Adv Funct Mater. 2018;28(39). doi: 10.1002/adfm.201801850
- Johnson BN, Lancaster KZ, Zhen G, et al. 3D printed anatomical nerve regeneration pathways. Adv Funct Mater. 2015;25(39):6205-6217. doi: 10.1002/adfm.201501760
- Oliveira EP, Malysz-Cymborska I, Golubczyk D, et al. Advances in bioinks and in vivo imaging of biomaterials for CNS applications. Acta Biomater. 2019;95:60-72. doi: 10.1016/j.actbio.2019.05.006
- O’Brien CM, Holmes B, Faucett S, Zhang LG. Three-dimensional printing of nanomaterial scaffolds for complex tissue regeneration. Tissue Eng Part B Rev. 2015;21(1):103-114. doi: 10.1089/ten.TEB.2014.0168
- Hsiao D, Hsu SH, Chen RS, Chen MH. Characterization of designed directional polylactic acid 3D scaffolds for neural differentiation of human dental pulp stem cells. J Formos Med Assoc. 2020;119(1 Pt 2):268-275. doi: 10.1016/j.jfma.2019.05.011
- Wang J, Zhang Y, Aghda NH, et al. Emerging 3D printing technologies for drug delivery devices: current status and future perspective. Adv Drug Deliv Rev. 2021;174:294-316. doi: 10.1016/j.addr.2021.04.019
- Wu GH, Hsu SH. Review: polymeric-based 3D printing for tissue engineering. J Med Biol Eng. 2015;35(3):285-292. doi: 10.1007/s40846-015-0038-3
- Chen MY, Skewes J, Woodruff MA, Dasgupta P, Rukin NJ. Multi-colour extrusion fused deposition modelling: a low-cost 3D printing method for anatomical prostate cancer models. Sci Rep. 2020;10(1):10004. doi: 10.1038/s41598-020-67082-7
- Nazemi MM, Khodabandeh A, Hadjizadeh A. Near-field electrospinning: crucial parameters, challenges, and applications. ACS Appl Biomater. 2022;5(2):394-412. doi: 10.1021/acsabm.1c00944
- Sun D, Chang C, Li S, Lin L. Near-field electrospinning. Nano Lett. 2006;6(4):839-842. doi: 10.1021/nl0602701
- He FL, Li DW, He J, et al. A novel layer-structured scaffold with large pore sizes suitable for 3D cell culture prepared by near-field electrospinning. Mater Sci Eng C Mater Biol Appl. 2018;86:18-27. doi: 10.1016/j.msec.2017.12.016
- Chang C, Limkrailassiri K, Lin L. Continuous near-field electrospinning for large area deposition of orderly nanofiber patterns. Appl Phys Lett. 2008;93(12):123111. doi: 10.1063/1.2975834
- Zhao X, Lu X, Li K, et al. Double crosslinked biomimetic composite hydrogels containing topographical cues and WAY-316606 induce neural tissue regeneration and functional recovery after spinal cord injury. Bioact Mater. 2023;24:331-345. doi: 10.1016/j.bioactmat.2022.12.024
- Song S, Zhou J, Wan J, et al. Three-dimensional printing of microfiberreinforced hydrogel loaded with oxymatrine for treating spinal cord injury. Int J Bioprint. 2023;9(3). doi: 10.18063/ijb.692
- Maruo S, Nakamura O, Kawata S. Three-dimensional microfabrication with two-photon-absorbed photopolymerization. Opt Lett. 1997;22(2):132-134. doi: 10.1364/ol.22.000132
- Kawata S, Sun HB, Tanaka T, Takada K. Finer features for functional microdevices. Nature. 2001;412(6848):697-698. doi: 10.1038/35089130
- Lee K-S, Kim RH, Yang D-Y, Park SH. Advances in 3D nano/ microfabrication using two-photon initiated polymerization. Prog Polym Sci. 2008;33(6):631-681. doi: 10.1016/j.progpolymsci.2008.01.001
- Liska R, Schuster M, Inführ R, et al. Photopolymers for rapid prototyping. J Coat Technol Res. 2007;4(4):505-510. doi: 10.1007/s11998-007-9059-3
- Xing J-F, Dong X-Z, Chen W-Q, et al. Improving spatial resolution of two-photon microfabrication by using photoinitiator with high initiating efficiency. Appl Phys Lett. 2007;90(13):131106. doi: 10.1063/1.2717532
- Accardo A, Blatché M-C, Courson R, Loubinoux I, Vieu C, Malaquin L. Two-photon lithography and microscopy of 3D hydrogel scaffolds for neuronal cell growth. Biomed Phys Eng Express. 2018;4(2):027009. doi: 10.1088/2057-1976/aaab93
- Marino A, Ciofani G, Filippeschi C, et al. Two-photon polymerization of sub-micrometric patterned surfaces: investigation of cell-substrate interactions and improved differentiation of neuron-like cells. ACS Appl Mater Interfaces. 2013;5(24):13012-13021. doi: 10.1021/am403895k
- Huang J, Yap N, Walter M, et al. 3D-printed polypyrrole microneedle arrays for electronically controlled transdural drug release. ACS Biomater Sci Eng. 2022;8(4):1544-1553. doi: 10.1021/acsbiomaterials.1c01305
- Huang Y, Wu W, Liu H, et al. 3D printing of functional nerve guide conduits. Burns Trauma. 2021;9:tkab011. doi: 10.1093/burnst/tkab011
- Gao B, Yang Q, Zhao X, Jin G, Ma Y, Xu F. 4D bioprinting for biomedical applications. Trends Biotechnol. 2016;34(9): 746-756. doi: 10.1016/j.tibtech.2016.03.004
- Tse C, Whiteley R, Yu T, et al. Inkjet printing Schwann cells and neuronal analogue NG108-15 cells. Biofabrication. 2016;8(1):015017. doi: 10.1088/1758-5090/8/1/015017
- Gu Q, Tomaskovic-Crook E, Lozano R, et al. Functional 3D neural mini-tissues from printed gel-based bioink and human neural stem cells. Adv Healthc Mater. 2016;5(12):1429-1438. doi: 10.1002/adhm.201600095
- Lorber B, Hsiao WK, Hutchings IM, Martin KR. Adult rat retinal ganglion cells and glia can be printed by piezoelectric inkjet printing. Biofabrication. 2014;6(1):015001. doi: 10.1088/1758-5082/6/1/015001
- Pateman CJ, Harding AJ, Glen A, et al. Nerve guides manufactured from photocurable polymers to aid peripheral nerve repair. Biomaterials. 2015;49:77-89. doi: 10.1016/j.biomaterials.2015.01.055
- Choudary R, Saini N, Chopra DS, Singh D, Singh N. A comprehensive review of 3D bioprinting biomaterials: properties, strategies and wound healing application. J Mater Res. 2023;38(13):3264-3300. doi: 10.1557/s43578-023-01078-7
- Yan X, Tong Y, Wang X, Hou F, Liang J. Extrusion-based 3D-printed supercapacitors: recent progress and challenges. Energy Environ Mater. 2022;5(3):800-822. doi: 10.1002/eem2.12260
- Cui H, Nowicki M, Fisher JP, Zhang LG. 3D bioprinting for organ regeneration. Adv Healthc Mater. 2017;6(1). doi: 10.1002/adhm.201601118
- Wang R, Wang Y, Yao B, et al. Redirecting differentiation of mammary progenitor cells by 3D bioprinted sweat gland microenvironment. Burns Trauma. 2019;7:29. doi: 10.1186/s41038-019-0167-y
- Liu X, Zhang J, Cheng X, et al. Integrated printed BDNF-stimulated HUCMSCs-derived exosomes/collagen/chitosan biological scaffolds with 3D printing technology promoted the remodelling of neural networks after traumatic brain injury. Regen Biomater. 2023;10:rbac085. doi: 10.1093/rb/rbac085
- Jiang JP, Liu XY, Zhao F, et al. Three-dimensional bioprinting collagen/silk fibroin scaffold combined with neural stem cells promotes nerve regeneration after spinal cord injury. Neural Regen Res. 2020;15(5):959-968. doi: 10.4103/1673-5374.268974
- Li Y, Cao X, Deng W, et al. 3D printable sodium alginate-matrigel (SA-MA) hydrogel facilitated ectomesenchymal stem cells (EMSCs) neuron differentiation. J Biomater Appl. 2021;35(6):709-719. doi: 10.1177/0885328220961261
- Bishop ES, Mostafa S, Pakvasa M, et al. 3-D bioprinting technologies in tissue engineering and regenerative medicine: current and future trends. Genes Dis. 2017;4(4):185-195. doi: 10.1016/j.gendis.2017.10.002
- Sanz-Garcia A, Sodupe-Ortega E, Pernía-Espinoza A, Shimizu T, Escobedo-Lucea C. A versatile open-source printhead for low-cost 3D microextrusion-based bioprinting. Polymers. 2020;12(10). doi: 10.3390/polym12102346
- Gauvin R, Chen YC, Lee JW, et al. Microfabrication of complex porous tissue engineering scaffolds using 3D projection stereolithography. Biomaterials. 2012;33(15):3824-3834. doi: 10.1016/j.biomaterials.2012.01.048
- Mandrycky C, Wang Z, Kim K, Kim DH. 3D bioprinting for engineering complex tissues. Biotechnol Adv. 2016;34(4): 422-434. doi: 10.1016/j.biotechadv.2015.12.011
- Wang Z, Abdulla R, Parker B, Samanipour R, Ghosh S, Kim K. A simple and high-resolution stereolithography-based 3D bioprinting system using visible light crosslinkable bioinks. Biofabrication. 2015;7(4):045009. doi: 10.1088/1758-5090/7/4/045009
- Tao J, Zhang J, Du T, et al. Rapid 3D printing of functional nanoparticle-enhanced conduits for effective nerve repair. Acta Biomater. 2019;90:49-59. doi: 10.1016/j.actbio.2019.03.047
- Derakhshanfar S, Mbeleck R, Xu K, Zhang X, Zhong W, Xing M. 3D bioprinting for biomedical devices and tissue engineering: a review of recent trends and advances. Bioact Mater. 2018;3(2):144-156. doi: 10.1016/j.bioactmat.2017.11.008
- Kim SH, Yeon YK, Lee JM, et al. Precisely printable and biocompatible silk fibroin bioink for digital light processing 3D printing. Nat Commun. 2018;9(1):1620. doi: 10.1038/s41467-018-03759-y
- Liu X, Tao J, Liu J, et al. 3D Printing enabled customization of functional microgels. ACS Appl Mater Interfaces. 2019;11(13):12209-12215. doi: 10.1021/acsami.8b18701
- Raspa A, Pugliese R, Maleki M, Gelain F. Recent therapeutic approaches for spinal cord injury. Biotechnol Bioeng. 2016;113(2):253-259. doi: 10.1002/bit.25689
- Slotkin JR, Pritchard CD, Luque B, et al. Biodegradable scaffolds promote tissue remodeling and functional improvement in non-human primates with acute spinal cord injury. Biomaterials. 2017;123:63-76. doi: 10.1016/j.biomaterials.2017.01.024
- Li J, Wu C, Chu PK, Gelinsky M. 3D printing of hydrogels: rational design strategies and emerging biomedical applications. Mater Sci Eng R Rep. 2020;140:100543. doi: 10.1016/j.mser.2020.100543
- Kuo KC, Lin RZ, Tien HW, et al. Bioengineering vascularized tissue constructs using an injectable cell-laden enzymatically crosslinked collagen hydrogel derived from dermal extracellular matrix. Acta Biomater. 2015;27:151-166. doi: 10.1016/j.actbio.2015.09.002
- Chen C, Zhao ML, Zhang RK, et al. Collagen/heparin sulfate scaffolds fabricated by a 3D bioprinter improved mechanical properties and neurological function after spinal cord injury in rats. J Biomed Mater Res Part A. 2017;105(5):1324-1332. doi: 10.1002/jbm.a.36011
- Jiang J, Liu X, Chen H, et al. 3D printing collagen/heparin sulfate scaffolds boost neural network reconstruction and motor function recovery after traumatic brain injury in canine. Biomater Sci. 2020;8(22):6362-6374. doi: 10.1039/d0bm01116a
- Wang Y, Zhang L, Guo K, Wang H. 3D printing of collagen nerve scaffold with multichannel characteristics. J Phys Conf Ser. 2023;2557(1):012058. doi: 10.1088/1742-6596/2557/1/012058
- Rockwood DN, Preda RC, Yücel T, Wang X, Lovett ML, Kaplan DL. Materials fabrication from Bombyx mori silk fibroin. Nat Protoc. 2011;6(10):1612-1631. doi: 10.1038/nprot.2011.379
- Qian KY, Song Y, Yan X, et al. Injectable ferrimagnetic silk fibroin hydrogel for magnetic hyperthermia ablation of deep tumor. Biomaterials. 2020;259:120299. doi: 10.1016/j.biomaterials.2020.120299
- Li XH, Zhu X, Liu XY, et al. The corticospinal tract structure of collagen/silk fibroin scaffold implants using 3D printing promotes functional recovery after complete spinal cord transection in rats. J Mater Sci Mater Med. 2021;32(4):31. doi: 10.1007/s10856-021-06500-2
- Kim SH, Yeon YK, Lee JM, et al. Precisely printable and biocompatible silk fibroin bioink for digital light processing 3D printing. Nat Commun. 2018;9(1):1620. doi: 10.1038/s41467-018-03759-y
- Wu W, Dong Y, Liu H, et al. 3D printed elastic hydrogel conduits with 7,8-dihydroxyflavone release for peripheral nerve repair. Mater Today Bio. 2023;20:100652. doi: 10.1016/j.mtbio.2023.100652
- Zhang X, Wu W, Huang Y, Yang X, Gou M. Antheraea pernyi silk fibroin bioinks for digital light processing 3D printing. Int J Bioprint. 2023;9(5):760. doi: 10.18063/ijb.760
- Stenberg L, Kodama A, Lindwall-Blom C, Dahlin LB. Nerve regeneration in chitosan conduits and in autologous nerve grafts in healthy and in type 2 diabetic Goto-Kakizaki rats. Eur J Neurosci. 2016;43(3):463-473. doi: 10.1111/ejn.13068
- Neubrech F, Sauerbier M, Moll W, et al. Enhancing the outcome of traumatic sensory nerve lesions of the hand by additional use of a chitosan nerve tube in primary nerve repair: a randomized controlled bicentric trial. Plast Reconstr Surg. Aug 2018;142(2):415-424. doi: 10.1097/prs.0000000000004574
- Zhao Y, Wang Y, Gong J, et al. Chitosan degradation products facilitate peripheral nerve regeneration by improving macrophage-constructed microenvironments. Biomaterials. 2017;134:64-77. doi: 10.1016/j.biomaterials.2017.02.026
- Sun Y, Yang C, Zhu X, et al. 3D printing collagen/chitosan scaffold ameliorated axon regeneration and neurological recovery after spinal cord injury. J Biomed Mater Res Part A. 2019;107(9):1898-1908. doi: 10.1002/jbm.a.36675
- Lindberg D, Kristoffersen KA, Wubshet SG, et al. Exploring effects of protease choice and protease combinations in enzymatic protein hydrolysis of poultry by-products. Molecules (Basel, Switzerland). 2021;26(17). doi: 10.3390/molecules26175280
- Duan B, Hockaday LA, Kang KH, Butcher JT. 3D bioprinting of heterogeneous aortic valve conduits with alginate/gelatin hydrogels. J Biomed Mater Res Part A. 2013;101(5):1255-1264. doi: 10.1002/jbm.a.34420
- Tran KA, DeOre BJ, Ikejiani D, et al. Matching mechanical heterogeneity of the native spinal cord augments axon infiltration in 3D-printed scaffolds. Biomaterials. 2023;295:122061. doi: 10.1016/j.biomaterials.2023.122061
- Murphy SV, Atala A. 3D bioprinting of tissues and organs. Nat Biotechnol. 2014;32(8):773-785. doi: 10.1038/nbt.2958
- Bae M, Hwang DW, Ko MK, et al. Neural stem cell delivery using brain-derived tissue-specific bioink for recovering from traumatic brain injury. Biofabrication. 2021;13(4). doi: 10.1088/1758-5090/ac293f
- Kong JS, Huang X, Choi YJ, et al. Promoting long-term cultivation of motor neurons for 3D neuromuscular junction formation of 3D in vitro using central-nervous-tissue-derived bioink. Adv Healthc Mater. 2021;10(18):e2100581. doi: 10.1002/adhm.202100581
- Gyles DA, Castro LD, Silva JOC, Ribeiro-Costa RM. A review of the designs and prominent biomedical advances of natural and synthetic hydrogel formulations. Eur Polym J. 2017;88:373-392. doi: 10.1016/j.eurpolymj.2017.01.027
- Assunção-Silva RC, Gomes ED, Sousa N, Silva NA, Salgado AJ. Hydrogels and cell based therapies in spinal cord injury regeneration. Stem Cells Int. 2015;2015:948040. doi: 10.1155/2015/948040
- Haggerty AE, Oudega M. Biomaterials for spinal cord repair. Neurosci Bull. 2013;29(4):445-459. doi: 10.1007/s12264-013-1362-7
- Kaplan B, Merdler U, Szklanny AA, et al. Rapid prototyping fabrication of soft and oriented polyester scaffolds for axonal guidance. Biomaterials. 2020;251:120062. doi: 10.1016/j.biomaterials.2020.120062
- Qian Y, Song J, Zhao X, et al. 3D fabrication with integration molding of a graphene oxide/polycaprolactone nanoscaffold for neurite regeneration and angiogenesis. Adv Sci. 2018;5(4):1700499. doi: 10.1002/advs.201700499
- Zhang C, Zhang N, Wen X. Synthesis and characterization of biocompatible, degradable, light-curable, polyurethane-based elastic hydrogels. J Biomed Mater Res Part A. 2007;82(3):637-650. doi: 10.1002/jbm.a.30992
- Yeganegi M, Kandel RA, Santerre JP. Characterization of a biodegradable electrospun polyurethane nanofiber scaffold: mechanical properties and cytotoxicity. Acta Biomater. 2010;6(10):3847-3855. doi: 10.1016/j.actbio.2010.05.003
- Huang CT, Kumar Shrestha L, Ariga K, Hsu SH. A graphene-polyurethane composite hydrogel as a potential bioink for 3D bioprinting and differentiation of neural stem cells. J Mater Chem B. 2017;5(44):8854-8864. doi: 10.1039/c7tb01594a
- Hsieh FY, Lin HH, Hsu SH. 3D bioprinting of neural stem cell-laden thermoresponsive biodegradable polyurethane hydrogel and potential in central nervous system repair. Biomaterials. 2015;71:48-57. doi: 10.1016/j.biomaterials.2015.08.028
- Lendlein A, Trask R. Multifunctional materials: concepts, function- structure relationships, knowledge-based design, translational materials research. Multifunct Mater. 2018;1. doi: 10.1088/2399-7532/aada7b
- Buwalda S. Bio-based composite hydrogels for biomedical applications. Multifunct Mater. 2020;3. doi: 10.1088/2399-7532/ab80d6
- Wei Z, Harris BT, Zhang LG. Gelatin methacrylamide hydrogel with graphene nanoplatelets for neural cell-laden 3D bioprinting. Annu Int Conf IEEE Eng Med Biol Soc. 2016;2016:4185-4188. doi: 10.1109/embc.2016.7591649
- Wu W, Dong Y, Liu H, et al. Modification of plasma protein for bioprinting via photopolymerization. Chin Chem Lett. 2023;109260. doi: 10.1016/j.cclet.2023.109260
- AlGhamdi KM, Kumar A, Moussa NA. Low-level laser therapy: a useful technique for enhancing the proliferation of various cultured cells. Lasers Med Sci. 2012;27(1): 237-249. doi: 10.1007/s10103-011-0885-2
- Chung H, Dai T, Sharma SK, Huang YY, Carroll JD, Hamblin MR. The nuts and bolts of low-level laser (light) therapy. Ann Biomed Eng. 2012;40(2):516-533. doi: 10.1007/s10439-011-0454-7
- Pjrek E, Friedrich ME, Cambioli L, et al. The efficacy of light therapy in the treatment of seasonal affective disorder: a meta-analysis of randomized controlled trials. Psychother Psychosom. 2020;89(1):17-24. doi: 10.1159/000502891
- Hashmi JT, Huang YY, Osmani BZ, Sharma SK, Naeser MA, Hamblin MR. Role of low-level laser therapy in neurorehabilitation. PM & R. 2010;2(12 Suppl 2):S292-S305. doi: 10.1016/j.pmrj.2010.10.013
- Zhu W, George JK, Sorger VJ, Grace Zhang L. 3D printing scaffold coupled with low level light therapy for neural tissue regeneration. Biofabrication. 2017;9(2):025002. doi: 10.1088/1758-5090/aa6999
- Sordini L, Garrudo FFF, Rodrigues CAV, et al. Effect of electrical stimulation conditions on neural stem cells differentiation on cross-linked PEDOT:PSS films. Front Bioeng Biotechnol. 2021;9:591838. doi: 10.3389/fbioe.2021.591838
- Fu C, Pan S, Ma Y, Kong W, Qi Z, Yang X. Effect of electrical stimulation combined with graphene-oxide-based membranes on neural stem cell proliferation and differentiation. Artif Cells Nanomed Biotechnol. 2019;47(1):1867-1876. doi: 10.1080/21691401.2019.1613422
- Yang K, Yu SJ, Lee JS, et al. Electroconductive nanoscale topography for enhanced neuronal differentiation and electrophysiological maturation of human neural stem cells. Nanoscale. 2017;9(47):18737-18752. doi: 10.1039/c7nr05446g
- Heo DN, Acquah N, Kim J, Lee SJ, Castro NJ, Zhang LG. Directly induced neural differentiation of human adipose-derived stem cells using three-dimensional culture system of conductive microwell with electrical stimulation. Tissue Eng Part A. 2018;24(7-8):537-545. doi: 10.1089/ten.TEA.2017.0150
- Rahmani A, Nadri S, Kazemi HS, Mortazavi Y, Sojoodi M. Conductive electrospun scaffolds with electrical stimulation for neural differentiation of conjunctiva mesenchymal stem cells. Artif Organs. 2019;43(8):780-790. doi: 10.1111/aor.13425
- Heo DN, Lee SJ, Timsina R, Qiu X, Castro NJ, Zhang LG. Development of 3D printable conductive hydrogel with crystallized PEDOT:PSS for neural tissue engineering. Mater Sci Eng C Mater Biol Appl. 2019;99:582-590. doi: 10.1016/j.msec.2019.02.008
- Song S, Li Y, Huang J, Cheng S, Zhang Z. Inhibited astrocytic differentiation in neural stem cell-laden 3D bioprinted conductive composite hydrogel scaffolds for repair of spinal cord injury. Biomater Adv. 2023;148:213385. doi: 10.1016/j.bioadv.2023.213385
- Vijayavenkataraman S, Zhang S, Thaharah S, Sriram G, Lu WF, Fuh JYH. Electrohydrodynamic jet 3D printed nerve guide conduits (NGCs) for peripheral nerve injury repair. Polymers. 2018;10(7). doi: 10.3390/polym10070753
- Vijayavenkataraman S, Kannan S, Cao T, Fuh JYH, Sriram G, Lu WF. 3D-printed PCL/PPy conductive scaffolds as three-dimensional porous nerve guide conduits (NGCs) for peripheral nerve injury repair. Front Bioeng Biotechnol. 2019;7:266. doi: 10.3389/fbioe.2019.00266
- Vijayavenkataraman S, Thaharah S, Zhang S, Lu WF, Fuh JYH. 3D-printed PCL/rGO conductive scaffolds for peripheral nerve injury repair. Artif Organs. 2019;43(5):515-523. doi: 10.1111/aor.13360
- Vijayavenkataraman S, Thaharah S, Zhang S, Lu WF, Fuh JYH. Electrohydrodynamic jet 3D-printed PCL/PAA conductive scaffolds with tunable biodegradability as nerve guide conduits (NGCs) for peripheral nerve injury repair. Mater Des. 2019;162:171-184. doi: 10.1016/j.matdes.2018.11.044
- Qian Y, Zhao X, Han Q, Chen W, Li H, Yuan W. An integrated multi-layer 3D-fabrication of PDA/RGD coated graphene loaded PCL nanoscaffold for peripheral nerve restoration. Nat Commun. 2018;9(1):323. doi: 10.1038/s41467-017-02598-7
- Zhu W, Tringale KR, Woller SA, et al. Rapid continuous 3D printing of customizable peripheral nerve guidance conduits. Mater Today. 2018;21(9):951-959. doi: 10.1016/j.mattod.2018.04.001
- Liu K, Yan L, Li R, et al. 3D printed personalized nerve guide conduits for precision repair of peripheral nerve defects. Adv Sci. 2022;9(12):e2103875. doi: 10.1002/advs.202103875
- Song S, Zhou J, Wan J, et al. Three-dimensional printing of microfiber- reinforced hydrogel loaded with oxymatrine for treating spinal cord injury. Int J Bioprint. 2023;9(3):692. doi: 10.18063/ijb.692
- Jin DK, Shido K, Kopp HG, et al. Cytokine-mediated deployment of SDF-1 induces revascularization through recruitment of CXCR4+ hemangiocytes. Nat Med. 2006;12(5):557-567. doi: 10.1038/nm1400
- Cheng X, Wang H, Zhang X, et al. The role of SDF-1/CXCR4/ CXCR7 in neuronal regeneration after cerebral ischemia. Front Neurosci. 2017;11:590. doi: 10.3389/fnins.2017.00590
- Li C, Kuss M, Kong Y, et al. 3D printed hydrogels with aligned microchannels to guide neural stem cell migration. ACS Biomater Sci Eng. 2021;7(2):690-700. doi: 10.1021/acsbiomaterials.0c01619
- Kim TH, Yoon SJ, Lee SM. Genipin attenuates sepsis by inhibiting Toll-like receptor signaling. Mol Med. 2012;18(1):455-465. doi: 10.2119/molmed.2011.00308
- Da Silva K, Kumar P, van Vuuren SF, Pillay V, Choonara YE. Three-dimensional printability of an ECM-based gelatin methacryloyl (GelMA) biomaterial for potential neuroregeneration. ACS Omega. 2021;6(33):21368-21383. doi: 10.1021/acsomega.1c01903
- Chang HM, Liu CH, Hsu WM, et al. Proliferative effects of melatonin on Schwann cells: implication for nerve regeneration following peripheral nerve injury. J Pineal Res. 2014;56(3):322-332. doi: 10.1111/jpi.12125
- Qian Y, Han Q, Zhao X, et al. 3D melatonin nerve scaffold reduces oxidative stress and inflammation and increases autophagy in peripheral nerve regeneration. J Pineal Res. 2018;65(4):e12516. doi: 10.1111/jpi.12516
- Xu X, Tao J, Wang S, et al. 3D printing of nerve conduits with nanoparticle-encapsulated RGFP966. Appl Mater Today. 2019;16:247-256. doi: 10.1016/j.apmt.2019.05.014
- Zhang J, Chen Y, Huang Y, et al. A 3D-printed self-adhesive bandage with drug release for peripheral nerve repair. Adv Sci. 2020;7(23):2002601. doi: 10.1002/advs.202002601
- Holtzman DM, Sheldon RA, Jaffe W, Cheng Y, Ferriero DM. Nerve growth factor protects the neonatal brain against hypoxic-ischemic injury. Ann Neurol. 1996;39(1): 114-122. doi: 10.1002/ana.410390117
- Keefe KM, Sheikh IS, Smith GM. Targeting neurotrophins to specific populations of neurons: NGF, BDNF, and NT-3 and their relevance for treatment of spinal cord injury. Int J Mol Sci. 2017;18(3). doi: 10.3390/ijms18030548
- Liu XY, Chen C, Xu HH, et al. Integrated printed BDNF/ collagen/chitosan scaffolds with low temperature extrusion 3D printer accelerated neural regeneration after spinal cord injury. Regen Biomater. 2021;8(6):rbab047. doi: 10.1093/rb/rbab047
- Lee YB, Polio S, Lee W, et al. Bio-printing of collagen and VEGF-releasing fibrin gel scaffolds for neural stem cell culture. Exp Neurol. 2010;223(2):645-652. doi: 10.1016/j.expneurol.2010.02.014
- Tao J, Liu H, Wu W, et al. 3D-printed nerve conduits with live platelets for effective peripheral nerve repair. Adv Funct Mater. 2020;30(42):2004272. doi: 10.1002/adfm.202004272
- Kalluri R, LeBleu VS. The biology, function, and biomedical applications of exosomes. Science. 2020;367(6478). doi: 10.1126/science.aau6977
- Wen SW, Lima LG, Lobb RJ, et al. Breast cancer-derived exosomes reflect the cell-of-origin phenotype. Proteomics. 2019;19(8):e1800180. doi: 10.1002/pmic.201800180
- Liu XY, Feng YH, Feng QB, et al. Low-temperature 3D-printed collagen/chitosan scaffolds loaded with exosomes derived from neural stem cells pretreated with insulin growth factor-1 enhance neural regeneration after traumatic brain injury. Neural Regen Res. 2023;18(9):1990-1998. doi: 10.4103/1673-5374.366497
- Liu X, Wang J, Wang P, et al. Hypoxia-pretreated mesenchymal stem cell-derived exosomes-loaded low-temperature extrusion 3D-printed implants for neural regeneration after traumatic brain injury in canines. Front Bioeng Biotechnol. 2022;10:1025138. doi: 10.3389/fbioe.2022.1025138
- Shinozaki M, Nagoshi N, Nakamura M, Okano H. Mechanisms of stem cell therapy in spinal cord injuries. Cells. 2021;10(10). doi: 10.3390/cells10102676
- Yousefifard M, Nasseri Maleki S, Askarian-Amiri S, et al. A combination of mesenchymal stem cells and scaffolds promotes motor functional recovery in spinal cord injury: a systematic review and meta-analysis. J Neurosurg Spine. 2019;32(2):269-284. doi: 10.3171/2019.8.Spine19201
- Fan L, Liu C, Chen X, et al. Directing induced pluripotent stem cell derived neural stem cell fate with a three-dimensional biomimetic hydrogel for spinal cord injury repair. ACS Appl Mater Interfaces. 2018;10(21):17742-17755. doi: 10.1021/acsami.8b05293
- Pereira IM, Marote A, Salgado AJ, Silva NA. Filling the gap: neural stem cells as a promising therapy for spinal cord injury. Pharmaceuticals. 2019;12(2). doi: 10.3390/ph12020065
- Younsi A, Zheng G, Riemann L, et al. Long-term effects of neural precursor cell transplantation on secondary injury processes and functional recovery after severe cervical contusion-compression spinal cord injury. Int J Mol Sci. 2021;22(23). doi: 10.3390/ijms222313106
- Giraldo E, Nebot VJ, Đorđević S, et al. A rationally designed self-immolative linker enhances the synergism between a polymer-rock inhibitor conjugate and neural progenitor cells in the treatment of spinal cord injury. Biomaterials. 2021;276:121052. doi: 10.1016/j.biomaterials.2021.121052
- Mothe AJ, Tam RY, Zahir T, Tator CH, Shoichet MS. Repair of the injured spinal cord by transplantation of neural stem cells in a hyaluronan-based hydrogel. Biomaterials. 2013;34(15):3775-3783. doi: 10.1016/j.biomaterials.2013.02.002
- Koffler J, Zhu W, Qu X, et al. Biomimetic 3D-printed scaffolds for spinal cord injury repair. Nat Med. 2019;25(2):263-269. doi: 10.1038/s41591-018-0296-z
- Liu X, Hao M, Chen Z, et al. 3D bioprinted neural tissue constructs for spinal cord injury repair. Biomaterials. 2021;272:120771. doi: 10.1016/j.biomaterials.2021.120771
- Yang J, Yang K, Man W, et al. 3D bio-printed living nerve-like fibers refine the ecological niche for long-distance spinal cord injury regeneration. Bioact Mater. 2023;25:160-175. doi: 10.1016/j.bioactmat.2023.01.023
- Li Y, Lv S, Yuan H, et al. Peripheral nerve regeneration with 3D printed bionic scaffolds loading neural crest stem cell derived schwann cell progenitors. Adv Funct Mater. 2021;31(16):2010215. doi: 10.1002/adfm.202010215
- Cho SR, Kim YR, Kang HS, et al. Functional recovery after the transplantation of neurally differentiated mesenchymal stem cells derived from bone marrow in a rat model of spinal cord injury. Cell Transplant. 2009;18(12): 1359-1368. doi: 10.3727/096368909x475329
- Luo H, Xu C, Liu Z, et al. Neural differentiation of bone marrow mesenchymal stem cells with human brain-derived neurotrophic factor gene-modified in functionalized self-assembling peptide hydrogel in vitro. J Cell Biochem. 2019;120(3):2828-2835. doi: 10.1002/jcb.26408
- GBD 2016 Traumatic Brain Injury and Spinal Cord Injury Collaborators. Global, regional, and national burden of traumatic brain injury and spinal cord injury, 1990-2016: a systematic analysis for the Global Burden of Disease Study 2016. Lancet Neurol. 2019;18(1):56-87. doi: 10.1016/s1474-4422(18)30415-0
- Ocansey DKW, Pei B, Yan Y, et al. Improved therapeutics of modified mesenchymal stem cells: an update. J Transl Med. 2020;18(1):42. doi: 10.1186/s12967-020-02234-x
- Chen C, Xu HH, Liu XY, et al. 3D printed collagen/ silk fibroin scaffolds carrying the secretome of human umbilical mesenchymal stem cells ameliorated neurological dysfunction after spinal cord injury in rats. Regen Biomater. 2022;9:rbac014. doi: 10.1093/rb/rbac014
- Qian Y, Gong J, Lu K, et al. DLP printed hDPSC-loaded GelMA microsphere regenerates dental pulp and repairs spinal cord. Biomaterials. 2023;299:122137. doi: 10.1016/j.biomaterials.2023.122137
- Qiu C, Sun Y, Li J, et al. Therapeutic effect of biomimetic scaffold loaded with human amniotic epithelial cell-derived neural-like cells for spinal cord injury. Bioengineering. 2022;9(10). doi: 10.3390/bioengineering9100535
- Hu Y, Wu Y, Gou Z, et al. 3D-engineering of cellularized conduits for peripheral nerve regeneration. Sci Rep. 2016;6:32184. doi: 10.1038/srep32184
- Li Y, Shen PP, Wang B. Induced pluripotent stem cell technology for spinal cord injury: a promising alternative therapy. Neural Regen Res. 2021;16(8):1500-1509. doi: 10.4103/1673-5374.303013
- Moradi S, Mahdizadeh H, Šarić T, et al. Research and therapy with induced pluripotent stem cells (iPSCs): social, legal, and ethical considerations. Stem Cell Res Ther. 2019;10(1):341. doi: 10.1186/s13287-019-1455-y
- Gaudet AD, Fonken LK. Glial cells shape pathology and repair after spinal cord injury. Neurotherapeutics. 2018;15(3):554-577. doi: 10.1007/s13311-018-0630-7
- Sun F, Shi T, Zhou T, et al. 3D poly(lactic-co-glycolic acid) scaffolds for treating spinal cord injury. J Biomed Nanotechnol. 2017;13(3):290-302. doi: 10.1166/jbn.2017.2348
- Wu Z, Li Q, Xie S, Shan X, Cai Z. In vitro and in vivo biocompatibility evaluation of a 3D bioprinted gelatin-sodium alginate/rat Schwann-cell scaffold. Mater Sci Eng C. 2020;109:110530. doi: 10.1016/j.msec.2019.110530
- Silva NA, Salgado AJ, Sousa RA, et al. Development and characterization of a novel hybrid tissue engineering-based scaffold for spinal cord injury repair. Tissue Eng Part A. 2010;16(1):45-54. doi: 10.1089/ten.TEA.2008.0559
- Liu S, Yang H, Chen D, et al. Three-dimensional bioprinting sodium alginate/gelatin scaffold combined with neural stem cells and oligodendrocytes markedly promoting nerve regeneration after spinal cord injury. Regen Biomater. 2022;9:rbac038. doi: 10.1093/rb/rbac038
- Wang J, Kong X, Li Q, et al. The spatial arrangement of cells in a 3D-printed biomimetic spinal cord promotes directional differentiation and repairs the motor function after spinal cord injury. Biofabrication. 2021;13(4). doi: 10.1088/1758-5090/ac0c5f
- Qian Y, Song J, Zheng W, et al. 3D manufacture of gold nanocomposite channels facilitates neural differentiation and regeneration. Adv Funct Mater. 2018;28(14):1707077. doi: 10.1002/adfm.201707077
- Liu X, Song S, Chen Z, et al. Release of O-GlcNAc transferase inhibitor promotes neuronal differentiation of neural stem cells in 3D bioprinted supramolecular hydrogel scaffold for spinal cord injury repair. Acta Biomater. 2022;151:148-162. doi: 10.1016/j.actbio.2022.08.031