3D-bioprinted bone scaffolds incorporating SR1 nanoparticles enhance blood vessel regeneration in rat calvarial defects
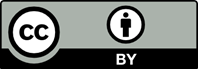
The inherent limitations of bone grafting in the treatment of critical-sized bone defects have led to a growing demand for bone repair implants. Three-dimensional (3D) bioprinting has emerged as a promising manufacturing technique for implants, offering flexibility in their structural design and the use of applicable materials. Although numerous 3D-bioprinted bone scaffolds have been developed to enhance osteogenesis, angiogenesis remains a challenge. Angiogenesis is crucial for successful bone healing because the process forms blood vessels to deliver essential nutrients and oxygen. Endothelial progenitor cells (EPCs) play a pivotal role in the early stages of vascularization. These cells, capable of differentiating into endothelial cells (ECs), are recruited from the bone marrow to the injured area during the healing process. CD34+ cells, a subset of EPCs, have gained attention because of their neovascularization potential and ability to contribute to bone regeneration. The incorporation of CD34+ cell-enhancing factors into 3D-printed bone scaffolds may facilitate successful bone healing in critical defects. StemRegenin-1 (SR1), a molecule that promotes CD34+ cell expansion, has shown promising results in increasing CD34+ hematopoietic stem and progenitor cell populations. This study aimed to investigate the sustained release of SR1 from a collagen-based scaffold integrated with mesoporous silica nanoparticles (MSNs) to promote angiogenesis and enhance bone healing. The sustained release of SR1 from the collagen scaffold is hypothesized to promote angiogenesis, thereby facilitating bone repair. In vitro studies have demonstrated the angiogenic potential of SR1; however, further in vivo investigations are required to establish its clinical efficacy. This study contributes to the development of novel therapies targeting CD34+ cells and demonstrates the potential of SR1 as a promising agent for promoting angiogenesis and enhancing bone healing in critical defects.
- Roberts TT, Rosenbaum AJ. Bone grafts, bone substitutes and orthobiologics: the bridge between basic science and clinical advancements in fracture healing. Organogenesis. 2012;8(4):114-124. doi: 10.4161/org.23306
- Zhang L, Yang G, Johnson BN, Jia X. Three-dimensional (3D) printed scaffold and material selection for bone repair. Acta Biomater. 2019;84:16-33. doi: 10.1016/j.actbio.2018.11.039
- Wang H, Su K, Su L, Liang P, Ji P, Wang C. The effect of 3D-printed Ti(6)Al(4)V scaffolds with various macropore structures on osteointegration and osteogenesis: a biomechanical evaluation. J Mech Behav Biomed Mater. 2018;88:488-496. doi: 10.1016/j.jmbbm.2018.08.049
- Pei X, Ma L, Zhang B, et al. Creating hierarchical porosity hydroxyapatite scaffolds with osteoinduction by three-dimensional printing and microwave sintering. Biofabrication. 2017;9(4):045008. doi: 10.1088/1758-5090/aa90ed
- Dadhich P, Das B, Pal P, et al. A simple approach for an eggshell-based 3D-printed osteoinductive multiphasic calcium phosphate scaffold. ACS Appl Mater Interfaces. 2016;8(19):11910-11924. doi: 10.1021/acsami.5b11981
- Raisian S, Fallahi HR, Khiabani KS, Heidarizadeh M, Azdoo S. Customized titanium mesh based on the 3D printed model vs. manual intraoperative bending of titanium mesh for reconstructing of orbital bone fracture: a randomized clinical trial. Rev Recent Clin Trials. 2017;12(3):154-158. doi: 10.2174/1574887112666170821165206
- Kuttappan S, Mathew D, Jo JI, et al. Dual release of growth factor from nanocomposite fibrous scaffold promotes vascularisation and bone regeneration in rat critical sized calvarial defect. Acta Biomater. 2018;78:36-47. doi: 10.1016/j.actbio.2018.07.050
- Rouwkema J, Rivron NC, van Blitterswijk CA. Vascularization in tissue engineering. Trends Biotechonol. 2008;26(8):434-441. doi: 10.1016/j.tibtech.2008.04.009
- Urbich C, Dimmeler S. Endothelial progenitor cells: characterization and role in vacular biology. Circ Res. 2004;95(4):343-353. doi: 10.1161/01.RES.0000137877.89448.78
- Asahara T, Murohara T, Sullivan A, et al. Isolation of putative progenitor endothelial cells for angiogenesis. Science. 1997;275(5302):964-967. doi: 10.1126/science.275.5302.964
- Hertweck J, Ritz U, Götz H, Schottel PC, Rommens PM, Hofmann A. CD34(+) cells seeded in collagen scaffolds promote bone formation in a mouse calvarial defect model. J Biomed Mater Res B Appl Biomater. 2018;106(4):1505-1516. doi: 10.1002/jbm.b.33956
- Kawamoto A, Iwasaki H, Kusano K, et al. CD34-positive cells exhibit increased potency and safety for therapeutic neovascularization after myocardial infarction compared with total mononuclear cells. Circulation. 2018;114(20): 2163-2169. doi: 10.1161/CIRCULATIONAHA.106.644518
- Musialek P, Tekieli L, Kostkiewicz M, et al. Randomized transcoronary delivery of CD34(+) cells with perfusion versus stop-flow method in patients with recent myocardial infarction: early cardiac retention of (9)(9)(m)Tc-labeled cells activity. J Nucl Cardiol. 2011;18(1):104-116. doi: 10.1007/s12350-010-9326-z
- Pasquet S, Sovalat H, Hénon P, et al. Long-term benefit of intracardiac delivery of autologous granulocyte-colony-stimulating factor-mobilized blood CD34+ cells containing cardiac progenitors on regional heart structure and function after myocardial infarct. Cytotherapy. 2009;11(8): 1002-1015. doi: 10.3109/14653240903164963
- Sivan-Loukianova E, Awad OA, Stepanovic V, Bickenbach J, Schatteman GC. CD34+ blood cells accelerate vascularization and healing of diabetic mouse skin wounds. J Vasc Res. 2003;40(4):368-377. doi: 10.1159/000072701
- O E, Lee BH, Ahn HY, et al. Efficient nanadhesive ex vivo expansion of early endothelial progenitor cells derived from CD34+ human cord blood fraction for effective therapeutic vascularization. FASEB J. 2011;25(1):159-169. doi: 10.1096/fj.10-162040
- Mifune Y, Matsumoto T, Kawamoto A, et al. Local delivery of granulocyte colony stimulating factor-mobilized CD34- positive progenitor cells using bioscaffold for modality of unhealing bone fracture. Stem Cells. 2008;26(6):1395-1405. doi: 10.1634/stemcells.2007-0820
- Matsumoto T, Kawamoto A, Kuroda R, et al. Therapeutic potential of vasculagenesis and osteogenesis promoted by peripheral blood CD34-positive cells for functional bone healing. Am J Pathol. 2006;169(4):1440-1457. doi: 10.2353/ajpath.2006.060064
- Boitano AE, Wang J, Romeo R, et al. Aryl hydrocarbon receptor antagonists promote the expansion of human hematopoietic stem cells. Science. 2010;329(5997):1345-1348. doi: 10.1126/science.1191536
- Wagner JE Jr, Brunstein CG, Boitano AE, et al. Phase I/ II trial of StemRegenin-1 expanded umbilical cord blood hematopoietic stem cells supports testing as a stand-alone graft. Cell Stem Cell. 2016;18(1):144-155. doi: 10.1016/j.stem.2015.10.004
- Smith KJ, Murray IA, Tanos R, et al. Identification of a high-affinity ligand that exhibits complete aryl hydrocarbon receptor antagonism. J Pharmacol Exp Ther. 2011;338(1):318-327. doi: 10.1124/jpet.110.178392
- Cao L, Mooney DJ. Spatiotemporal control over growth factor signaling for therapeutic neovascularization. Adv Drug Deliv Rev. 2007;59(13):1340-1350. doi: 10.1016/j.addr.2007.08.012
- Fahimipour F, Rasoulianboroujeni M, Dashtimoghadam E, et al. 3D printed TCP-based scaffold incorporating VEGF-loaded PLGA microspheres for craniofacial tissue engineering. Dent Mater. 2017;33(11):1205-1216. doi: 10.1016/j.dental.2017.06.016
- Matassi F, Nistri L, Chicon Paez D, Innocenti M. New biomaterials for bone regeneration. Clin Cases Miner Bone Metab. 2011;8(1):21-24.
- Jafari M, Paknejad Z, Rad MR, et al. Polymeric scaffolds in tissue engineering: a literature review. J Biomed Mater Res B Appl Biomater. 2017;105(2):431-459. doi: 10.1002/jbm.b.33547
- Kim SC, Heo SY, Oh GW, Yi M, Jung W-K. A 3D-printed polycaprolactone/marine collagen scaffold reinforced with carbonated hydroxyapatite from fish bones for bone regeneration. Mar Drugs. 2022;20(6):344. doi: 10.3390/md20060344
- Murphy CM, Haugh MG, O’Brien FJ. The effect of mean pore size on cell attachment, proliferation and migration in collagen-glycosaminoglycan scaffolds for bone tissue engineering. Biomaterials. 2010;31(3):461-466. doi: 10.1016/j.biomaterials.2009.09.063
- Momot KI. Hydrated collagen: where physical chemistry, medical imaging, and bioengineering meet. J Phys Chem B. 2022;126(49):10305-10316. doi: 10.1021/acs.jpcb.2c06217
- Lim HJ, Jang WB, Rethineswaran VK, et al. StemRegenin-1 attenuates endothelial progenitor cell senescence by regulating the AhR pathway-mediated CYP1A1 and ROS generation. Cells. 2023;12(15):2005. doi: 10.3390/cells12152005
- Kang D, Lee YB, Yang GH, et al. FeS(2)-incorporated 3D PCL scaffold improves new bone formation and neovascularization in a rat calvarial defect model. Int J Bioprint. 2023;9(1):636. doi: 10.18063/ijb.v9i1.636
- Jang MJ, Bae SK, Jung YS, et al. Enhanced wound healing using a 3D printed VEGF-mimicking peptide incorporated hydrogel patch in a pig model. Biomed Mater. 2021;16(4). doi: 10.1088/1748-605X/abf1a8
- Gurcan MN, Boucheron LE, Can A, Madabhushi A, Rajpoot NM, Yener B. Histopathological image analysis: a review. IEEE Rev Biomed Eng. 2009;2:147-171. doi: 10.1109/RBME.2009.2034865
- Gavet O, Pines J. Progressive activation of CyclinB1- Cdk1 coordinates entry to mitosis. Dev Cell. 2010;18(4): 533-543. doi: 10.1016/j.devcel.2010.02.013
- Bancroft JD, Gamble M. Theory and Practice of Histological Techniques. 6th ed. Churchill Livingstone; 2008:105-134.
- Hankenson KD, Dishowitz M, Gray C, Schenker M. Angiogenesis in bone regeneration. Injury. 2011;42(6): 556-561. doi: 10.1016/j.injury.2011.03.035
- Kim JH, Park M, Shim JH, Yun W-S, Jin S. Multi-scale vascularization strategy for 3D-bioprinted tissue using coaxial core-shell pre-set extrusion bioprinting and biochemical factors. Int J Bioprint. 2023;9(4):726. doi: 10.18063/ijb.726
- Nasehi F, Karshenas M, Nardi S, Barati G, Salim A. Core-shell fibrous scaffold as a vehicle for sustained release of retinal pigmented epithelium-derived factor (PEDF) for photoreceptor differentiation of conjunctiva mesenchymal stem cells. J Biomed Mater Res A. 2017;105(12): 3514-3519. doi: 10.1002/jbm.a.36182
- Milojević M, Vihar B, Banović L, et al. Core/shell printing scaffolds for tissue engineering of tubular structures. J Vix Exp. 2019;(151). doi: 10.3791/59951
- Hassanpour M, Salybekov AA, Kobayashi S, Asahara T. CD34 positive cells as endothelial progenitor cells in biology and medicine. Front Cell Dev Biol. 2023;11:1128134. doi: 10.3389/fcell.2023.1128134
- Singh J, Chen ELY, Xing Y, Stefanski HE, Blazar BR, Zúñiga-Pflücker JC. Generation and function of progenitor T cells from StemRegenin-1-expanded CD34+ human hematopoietic progenitor cells. Blood Adv. 2019;3(20):2934-2948. doi: 10.1182/bloodadvances.2018026575
- Rakkar K, Othman OA, Sprigg N, Bath PM, Bayraktutan U. Evaluation of endothelial progenitor cell characteristics as clinical biomarkers for elderly patients with ischaemic stroke. Stem Cell Rev Rep. 2023;19(6):1856-1869. doi: 10.1007/s12015-023-10544-y
- Kamata S, Miyagawa S, Fukushima S, et al. Improvement of cardiac stem cell sheet therapy for chronic ischemic injury by adding endothelial progenitor cell transplantation: analysis of layer-specific regional cardiac function. Cell Transplant. 2014;23(10):1305-1319. doi: 10.3727/096368913X665602
- Wang X, Cooper S, Broxmeyer HE, Kapur R. Transient regulation of RNA methylation in human hematopoietic stem cells promotes their homing and engraftment. Leukemia. 2023;37(2):453-464. doi: 10.1038/s41375-022-01761-4
- Lewalle JM, Bajou K, Desreux J, et al. Alteration of interendothelial adherens junctions following tumor cell-endothelial cell interaction in vitro. Exp Cell Res. 1997;237(2):347-356. doi: 10.1006/excr.1997.3799
- Newman H, Shih YV, Varghese S. Resolution of inflammation in bone regeneration: from understandings to therapeutic applications. Biomaterials. 2021;277:121114. doi: 10.1016/j.biomaterials.2021.121114
- Marsell R, Einhorn TA. The biology of fracture healing. Injury. 2011;42(6):551-555. doi: 10.1016/j.injury.2011.03.031
- Schmidt-Bleek K, Schell H, Lienau J, et al. Initial immune reaction and angiogenesis in bone healing. J Tissue Eng Regen Med. 2014;8(2):120-130. doi: 10.1002/term.1505
- Usuda K, Iwai S, Funasaki A, et al. Expression and prognostic impact of VEGF, CD31 and alphaSMA in resected primary lung cancers. Anticancer Res. 2018;38(7):4057-4063. doi: 10.21873/anticancers.12695