Polydopamine chelating strontium on graphene oxide enhances the mechanical and osteogenic induction properties of PLLA/PGA bone scaffold
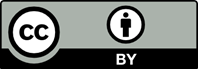
Poly (lactic acid)/poly (glycolic acid) (LG) bone scaffold exhibits good biocompatibility for bone defect regeneration but lacks satisfactory mechanical and osteogenic induction properties. Here, graphene oxide (GO) was encapsulated by polydopamine (PDA) via self-polymerization of dopamine, and strontium (Sr) was loaded onto GO by the chelation of PDA. The modified GO was added to the LG scaffold prepared via selective laser sintering as a reinforcing phase to improve the mechanical properties and osteogenic induction properties. The results indicated that the tensile and compressive strengths of the scaffold with 1.5 wt% modified GO were 9.49 MPa and 19.22 MPa, respectively, representing 67.08% and 95.33% improvement compared to the LG scaffold. The enhancement mechanisms of the modified GO in the scaffold included crack branching, crack deflection, crack pinning, crack bridging, and pulling out. More importantly, the scaffold with modified GO exhibited superior bioactivity and osteogenic induction properties compared to the LG scaffold, because PDA could chelate calcium ions derived from the surrounding physiological environment, and the calcium ions attracted phosphate ions through electrostatic interactions to promote the apatite layer deposition. Additionally, the presence of Sr in the scaffold promoted the proliferation and differentiation of osteoblasts, thereby improving osteogenic induction properties.
- Sun H, Ling L, Ren Z, Memon SA, Xing F. Effect of graphene oxide/graphene hybrid on mechanical properties of cement mortar and mechanism investigation. Nanomaterials (Basel). 2020;10(1):113. doi: 10.3390/nano10010113
- Chathuranga H, Wasalathilake KC, Marriam I, et al. Preparation of bioinspired graphene oxide/PMMA nanocomposite with improved mechanical properties. Compos Sci Technol. 2021;216:109046. doi: 10.1016/j.compscitech.2021.109046
- Qu H, Huang L, Han Z, et al. A review of graphene-oxide/metal– organic framework composites materials: characteristics, preparation and applications. J Porous Mat. 2021; 28:1837-1865. doi: 10.1007/s10934-021-01125-w
- Yan L, Wang L, Wu J, et al. Multi-biofunctional graphene oxide-enhanced poly-L-lactic acid composite nanofiber scaffolds for ovarian function recovery of transplanted-tissue. NPJ Regen Med. 2022;7(1):52. doi: 10.1038/s41536-022-00236-5
- Ju J, Peng X, Huang K, et al. High-performance porous PLLA-based scaffolds for bone tissue engineering: preparation, characterization, and in vitro and in vivo evaluation. Polymer. 2019;180:121707. doi: 10.1016/j.polymer.2019.121707
- Murakami T, Otsuki S, Nakagawa K, et al. Establishment of novel meniscal scaffold structures using polyglycolic and poly-l-lactic acids. J Biomater Appl. 2017;32:150-161. doi: 10.1177/0885328217713631
- Cantón I, Mckean R, Charnley M, et al. Development of an Ibuprofen‐releasing biodegradable PLA/PGA electrospun scaffold for tissue regeneration. Biotechnol Bioeng. 2010;105:396-408. doi: 10.1002/bit.22530
- Hua W, Shi W, Mitchell K, et al. 3D printing of biodegradable polymer vascular stents: a review. Chin J Mech Eng Addit Manuf Front. 2022;1(2):100020. doi: 10.1016/j.cjmeam.2022.100020
- Shuai C, Shi X, Yang F, Tian H, Feng P. Oxygen vacancy boosting Fenton reaction in bone scaffold towards fighting bacterial infection. Int J Extreme Manuf. 2023;6: 015101. doi: 10.1088/2631-7990/ad01fd
- Storck JL, Ehrmann G, Uthoff J, Diestelhorst E, Blachowicz T, Ehrmann A. Investigating inexpensive polymeric 3D printed materials under extreme thermal conditions. Mater Futures. 2022;1:015001. doi: 10.1088/2752-5724/ac4beb
- Najafinezhad A, Bakhsheshi-Rad HR, Saberi A, et al. Graphene oxide encapsulated forsterite scaffolds to improve mechanical properties and antibacterial behavior. Biomed Mater. 2022;17:035011. doi: 10.1088/1748-605X/ac62e8
- Abzan N, Kharaziha M, Labbaf S. Development of three-dimensional piezoelectric polyvinylidene fluoride-graphene oxide scaffold by non-solvent induced phase separation method for nerve tissue engineering. Mater Design. 2019;167:107636. doi: 10.1016/j.matdes.2019.107636
- Wang W, Wei J, Lei D, et al. 3D printing of lithium osteogenic bioactive composite scaffold for enhanced bone regeneration. Compos Part B Eng. 2023;256:110641. doi: 10.1016/j.compositesb.2023.110641
- Zhang X, He J, Qiao L, et al. 3D printed PCLA scaffold with nano‐hydroxyapatite coating doped green tea EGCG promotes bone growth and inhibits multidrug‐resistant bacteria colonization. Cell Prolif. 2022;55(10):e13289. doi: 10.1111/cpr.13289
- Chen A, Wang W, Mao Z, et al. Multi‐material 3D and 4D bioprinting of heterogeneous constructs for tissue engineering. Adv Mater. 2023:2307686. doi: 10.1002/adma.202307686
- Askari E, Rasouli M, Darghiasi SF, Naghib SM, Zare Y, Rhee KY. Reduced graphene oxide-grafted bovine serum albumin/bredigite nanocomposites with high mechanical properties and excellent osteogenic bioactivity for bone tissue engineering. Bio-Des Manuf. 2021;4:243-257. doi: 10.1007/s42242-020-00113-4
- Soraya Z, Ghollasi M, Halabian R, Eftekhari E, Tabasi A, Salimi A. Donepezil hydrochloride as a novel inducer for osteogenic differentiation of mesenchymal stem cells on PLLA scaffolds in vitro. Biotechnol J. 2021;16:2100112. doi: 10.1002/biot.202100112
- Toosi S, Naderi-Meshkin H, Kalalinia F, et al. Bone defect healing is induced by collagen sponge/polyglycolic acid. J Mater Sci Mater Med. 2019;30:1-10. doi: 10.1007/s10856-019-6235-9
- Bai H, Zhao Y, Wang C, et al. Enhanced osseointegration of three-dimensional supramolecular bioactive interface through osteoporotic microenvironment regulation. Theranostics. 2020;10:4779. doi: 10.7150/thno.43736
- Wang J, Wang H, Wang Y, et al. Endothelialized microvessels fabricated by microfluidics facilitate osteogenic differentiation and promote bone repair. Acta Biomater. 2022;142:85-98. doi: 10.1016/j.actbio.2022.01.055
- Jarrar H, Altındal DÇ, Gümüşderelioğlu M. Scaffold-based osteogenic dual delivery system with melatonin and BMP-2 releasing PLGA microparticles. Int J Pharmaceut. 2021;600:120489. doi: 10.1016/j.ijpharm.2021.120489
- Wu X, Tang Z, Wu K, et al. Strontium-calcium phosphate hybrid cement with enhanced osteogenic and angiogenic properties for vascularised bone regeneration. J Mater Chem B. 2021;9(2021):5982-5997. doi: 10.1039/D1TB00439E
- Zhang W, Tian Y, He H, et al. Strontium attenuates rhBMP- 2-induced osteogenic differentiation via formation of Sr-rhBMP-2 complex and suppression of Smad-dependent signaling pathway. Acta Biomater. 2016;33:290-300. doi: 10.1016/j.actbio.2016.01.042
- Li S, Cui Y, Liu H, et al. Application of bioactive metal ions in the treatment of bone defects. J Mater Chem B. 2022;10(45):9369-9388. doi: 10.1039/D2TB01684B
- Luo Y, Liu H, Zhang Y, et al. Metal ions: the unfading stars of bone regeneration-from bone metabolism regulation to biomaterial applications. Biomater Sci. 2023;11(22): 7268-7295. doi: 10.1039/D3BM01146A
- Yuan Z, Wei P, Huang Y, et al. Injectable PLGA microspheres with tunable magnesium ion release for promoting bone regeneration. Acta Biomater. 2019;85:294-309. doi: 10.1016/j.actbio.2018.12.017
- Yu Y, Liu K, Wen Z, Liu W, Zhang L, Su J. Double-edged effects and mechanisms of Zn2+ microenvironments on osteogenic activity of BMSCs: osteogenic differentiation or apoptosis. RSC Adv. 2020;10(25):14915-14927. doi: 10.1039/D0RA01465F
- Xue Y, Zhang L, Liu F, et al. Alkaline “nanoswords” coordinate ferroptosis-like bacterial death for antibiosis and osseointegration. ACS Nano. 2023;17(3):2711-2724. doi: 10.1021/acsnano.2c10960
- Kołodziejska B, StępieńN, Kolmas J. The influence of strontium on bone tissue metabolism and its application in osteoporosis treatment. Int J Mol Sci. 2021;22:6564. doi: 10.3390/ijms22126564
- Zhang C, Xu G, Han L, Hu X, Zhao Y, Li Z. Bone induction and defect repair by true bone ceramics incorporated with rhBMP-2 and Sr. J Mater Sci Mater Med. 2021;32:107. doi: 10.1007/s10856-021-06587-7
- Lakhkar NJ, Lee IH, Kim HW, Salih V, Wall IB, Knowles JC. Bone formation controlled by biologically relevant inorganic ions: role and controlled delivery from phosphate-based glasses. Adv Drug Deliver Rev. 2013;65(4):405-420. doi: 10.1016/j.addr.2012.05.015
- Schatkoski VM, Larissa do Amaral Montanheiro T, Canuto de Menezes, et al. Current advances concerning the most cited metal ions doped bioceramics and silicate-based bioactive glasses for bone tissue engineering. Ceram Int. 2021;47(3):2999-3012. doi: 10.1016/j.ceramint.2020.09.213
- Zhao R, Chen S, Zhao W, et al. A bioceramic scaffold composed of strontium-doped three-dimensional hydroxyapatite whiskers for enhanced bone regeneration in osteoporotic defects. Theranostics. 2020;10(4):1572. doi: 10.7150/thno.40103
- Szewczyk J, Aguilar-Ferrer D, Coy E. Polydopamine films: electrochemical growth and sensing applications. Eur Polym J. 2022;174:111346. doi: 10.1016/j.eurpolymj.2022.111346
- Wang LS, Xu S, Gopal S, et al. Facile fabrication of antibacterial and antiviral perhydrolase-polydopamine composite coatings. Sci Rep. 2021;11:12410. doi: 10.1038/s41598-021-91925-6
- Xie Y, Yue L, Zheng Y, et al. The antibacterial stability of poly (dopamine) in-situ reduction and chelation nano-Ag based on bacterial cellulose network template. Appl Surf Sci. 2019;491:383-394. doi: 10.1016/j.apsusc.2019.06.096
- Yang Z, Fan WH, Ding YJ, Xiao Z-L. Preparation and thermal performance of nitrocellulose coated by polydopamine. J Appl Polym Sci. 2022;139:51809. doi: 10.1002/app.51809
- Zhou S, Yan J, Chen J, et al. Polydopamine/polyethyleneimine co-crosslinked graphene oxide for the enhanced tribological performance of epoxy resin coatings. J Mater Sci Technol. 2023;136:13-20. doi: 10.1016/j.jmst.2022.07.019
- Gao T, Zhang J, Zhang N, Wang Y, He J, Wu F. Dopamine assisted incorporation of Sr ions in porous titanium alloy and its in-vitro bioactivity and cellular responses. Mater Lett. 2021;287:129308. doi: 10.1016/j.matlet.2021.129308
- Zhou X, Cheng X, Xing D, et al. Ca ions chelation, collagen I incorporation and 3D bionic PLGA/PCL electrospun architecture to enhance osteogenic differentiation. Mater Design. 2021;198:109300. doi: 10.1016/j.matdes.2020.109300
- Qi F, Liao R, Wu P, et al. An electrical microenvironment constructed based on electromagnetic induction stimulates neural differentiation. Mater Chem Front. 2023;7: 1671-1683. doi: 10.1039/D2QM01193J
- Gao C, Yao X, Deng Y, Pan H, Shuai C. Laser-beam powder bed fusion followed by annealing with stress: a promising route for magnetostrictive improvement of polycrystalline Fe81Ga19 alloys. Addit Manuf. 2023;68:103516. doi: 10.1016/j.addma.2023.103516
- Ling C, Li Q, Zhang Z, et al. Influence of heat treatment on microstructure, mechanical and corrosion behavior of WE43 alloy fabricated by laser-beam powder bed fusion. Int J Extreme Manuf. 2024;6:015001.doi: 10.1088/2631-7990/acfad5
- Han W, Kong L, Xu M. Advances in selective laser sintering of polymers. Int J Extreme Manuf. 2022;4:042002. doi: 10.1088/2631-7990/ac9096
- Feng J, Fu J, Yao X, He Y. Triply periodic minimal surface (TPMS) porous structures: from multi-scale design, precise additive manufacturing to multidisciplinary applications. Int J Extreme Manuf. 2022;4:022001. doi: 10.1088/2631-7990/ac5be6
- Hou Y, Wang W, Bartolo P. Application of additively manufactured 3D scaffolds for bone cancer treatment: a review. Bio Des Manuf. 2022;5:556-579. doi: 10.1007/s42242-022-00182-7
- Cui ZW, Jing YR, Liu SS, Liu GY. Systematic investigation on the effect of crosslinking agent type and dosage on the performance of TPU/MVQ based thermoplastic vulcanizates. J Appl Polym Sci. 2021;138:50630. doi: 10.1002/app.50630
- Liu Y, Qian L, Xia C, Zou J, Lian Z, Yi X. Failure analysis and structural optimization of rubber core and support rib of full-size spherical blowout preventer. Eng Fail Anal. 2023;143:106865. doi: 10.1016/j.engfailanal.2022.106865
- Goel P, Mandal P, Bhuvanesh E, Shahi VK, Chattopadhyay S. Temperature resistant cross-linked brominated poly phenylene oxide-functionalized graphene oxide nanocomposite anion exchange membrane for desalination. Sep Purif Technol. 2021;255:117730. doi: 10.1016/j.seppur.2020.117730
- Qiblawey H. Development of novel composite membranes in water/wastewater treatment. Membranes (Basel). 2022;12:260. doi: 10.3390/membranes12030260
- Kou Y, Zhou W, Xu L, et al. Surface modification of GO by PDA for dielectric material with well-suppressed dielectric loss. High Perform Polym. 2019;31:1183-1194. doi: 10.1177/0954008319837744
- Alkhouzaam A, Qiblawey H, Khraisheh M. Polydopamine functionalized graphene oxide as membrane nanofiller: spectral and structural studies. Membranes (Basel). 2021;11:86. doi: 10.3390/membranes11020086
- Song H, Wang Z, Yang J, Jia X, Zhang Z. Facile synthesis of copper/polydopamine functionalized graphene oxide nanocomposites with enhanced tribological performance. Chem Eng J. 2017;324:51-62. doi: 10.1016/j.cej.2017.05.016
- Ren Y, Peng D, Wu H, et al. Enhanced carbon dioxide flux by catechol-Zn2+ synergistic manipulation of graphene oxide membranes. Chem Eng J. 2019;195:230-238. doi: 10.1016/j.ces.2018.11.055
- Zhao Z, Guo L, Feng L, et al. Polydopamine functionalized graphene oxide nanocomposites reinforced the corrosion protection and adhesion properties of waterborne polyurethane coatings. Eur Polym J. 2019;120:109249. doi: 10.1016/j.eurpolymj.2019.109249
- Ye W, Chen Y, Zhou Y, et al. Enhancing the catalytic activity of flowerike Pt nanocrystals using polydopamine functionalized graphene supports for methanol electrooxidation. Electrochim Acta. 2014;142:18-24. doi: 10.1016/j.electacta.2014.06.161
- Wang S, Zhu J, Rao Y, et al. Polydopamine modified graphene oxide-TiO2 nanofiller for reinforcing physical properties and anticorrosion performance of waterborne epoxy coatings. Appl Sci. 2019;9:3760. doi: 10.3390/app9183760
- Wang R, Song F, Li S, et al. Polydopamine-assisted Sr immobilization to improve the osteogenesis of a three-dimensional reduced graphene oxide/polypyrrole composite scaffold. Mater Lett. 2019;246:182-185. doi: 10.1016/j.matlet.2019.03.065
- Jia Z, Xu X, Zhu D, Zheng Y. Design, printing, and engineering of regenerative biomaterials for personalized bone healthcare. Prog Mater Sci. 2023;134:101072. doi: 10.1016/j.pmatsci.2023.101072
- Tan X, Tan Y, Chow C, Tor SB, Yeong WY. Metallic powder-bed based 3D printing of cellular scaffolds for orthopaedic implants: a state-of-the-art review on manufacturing, topological design, mechanical properties and biocompatibility. Mat Sci Eng C Mater. 2017;76:1328-1343. doi: 10.1016/j.msec.2017.02.094
- Zhu H, Li M, Huang X, et al. 3D printed tricalcium phosphate-bioglass scaffold with gyroid structure enhance bone ingrowth in challenging bone defect treatment. Appl Mater Today. 2021;25:101166. doi: 10.1016/j.apmt.2021.101166
- Ibrahim A, Klopocinska A, Horvat K, Hamid ZA. Graphene-based nanocomposites: synthesis, mechanical properties, and characterizations. Polymers (Basel). 2021;13:2869. doi: 10.3390/polym13172869
- Wang M, Ma L, Shi L, et al. Chemical grafting of nano-SiO2 onto graphene oxide via thiol-ene click chemistry and its effect on the interfacial and mechanical properties of GO/ epoxy composites. Compos Sci Technol. 2019;182:107751. doi: 10.1016/j.compscitech.2019.107751
- Hu Y, Xie Y, Xin H, et al. Nano/microstructures and mechanical properties of Al2O3-TiC ceramic composites reinforced with Al2O3@RGO nanohybrids. Ceram Int. 2022;48:27536-27549. doi: 10.1016/j.ceramint.2022.06.047
- Li B, Ying P, Gao Y, et al. Heterogeneous diamond-cBN composites with superb toughness and hardness. Nano Lett. 2022;22:4979-4984.doi: 10.1021/acs.nanolett.2c01716
- Peng J, Huang C, Cao C, et al. Inverse nacre-like epoxy-graphene layered nanocomposites with integration of high toughness and self-monitoring. Matter. 2020;2:220-232. doi: 10.1016/j.matt.2019.08.013
- Gholinezhad F, Golhosseini R, Moini Jazani O. Synthesis, characterization, and properties of silicone grafted epoxy/acrylonitrile butadiene styrene/graphene oxide nanocomposite with high adhesion strength and thermal stability. Polym Composite. 2022;43:1665-1684. doi: 10.1002/pc.26487
- Mehwish N, Xu M, Zaeem M, Lee BH. Mussel-inspired surface functionalization of porous albumin cryogels supporting synergistic antibacterial/antioxidant activity and bone-like apatite formation. Gels. 2022;8:679. doi: 10.3390/gels8100679
- Deng Y, Yang WZ, Shi D, et al. Bioinspired and osteopromotive polydopamine nanoparticle-incorporated fibrous membranes for robust bone regeneration. NPG Asia Mater. 2019;11:39. doi: 10.1038/s41427-019-0139-5
- Ghorbani F, Zamanian A, Behnamghader A, Joupari MD. A facile method to synthesize mussel-inspired polydopamine nanospheres as an active template for in situ formation of biomimetic hydroxyapatite. Mat Sci Eng C Mater. 2019;94:729-739. doi: 10.1016/j.msec.2018.10.010
- Arepalli SK, Tripathi H, Hira SK, Manna PP, Pyare R, Singh SP. Enhanced bioactivity, biocompatibility and mechanical behavior of strontium substituted bioactive glasses. Mat Sci Eng C Mater Biol Appl. 2016;69:108-116. doi: 10.1016/j.msec.2016.06.070
- Ge J, Wang F, Xu Z, et al. Influences of niobium pentoxide on roughness, hydrophilicity, surface energy and protein absorption, and cellular responses to PEEK based composites for orthopedic applications. J Mater Chem B. 2020;8:2618-2626. doi: 10.1039/C9TB02456E
- Mi X, Su Z, Fu Y, Li S, Mo A. 3D printing of Ti3C2-MXene-incorporated composite scaffolds for accelerated bone regeneration. Biomed Mater. 2022;17:035002. doi: 10.1088/1748-605X/ac5ffe
- Qi F, Wang Z, Yang L, et al. A collaborative CeO2@metal-organic framework nanosystem to endow scaffolds with photodynamic antibacterial effect. Mater Today Chem. 2023;27:101336. doi: 10.1016/j.mtchem.2022.101336
- Guo W, Wang X, Yang C, Huang R, Wang H, Zhao Y. Microfluidic 3D printing polyhydroxyalkanoates-based bionic skin for wound healing. Mater Futures. 2022;1:015401. doi: 10.1088/2752-5724/ac446b
- Ng JY, Obuobi S, Chua ML, et al. Biomimicry of microbial polysaccharide hydrogels for tissue engineering and regenerative medicine: a review. Carbohyd Polym. 2020;241:116345. doi: 10.1016/j.carbpol.2020.116345
- Abdollahiyan P, Baradaran B, de la Guardia M, Oroojalian F, Mokhtarzadeh A. Cutting-edge progress and challenges in stimuli responsive hydrogel microenvironment for success in tissue engineering today. J Control Release. 2020; 328:514-531. doi: 10.1016/j.jconrel.2020.09.030
- Dong P, Zhu D, Deng X, et al. Effect of hydroxyapatite nanoparticles and wedelolactone on osteoblastogenesis from bone marrow mesenchymal stem cells. J Biomed Mater Res A. 2019;107:145-153. doi: 10.1002/jbm.a.36541
- Su Y, Li Z, Zhu H, He J, Wei B, Li D. Electrohydrodynamic fabrication of triple-layered polycaprolactone dura mater substitute with antibacterial and enhanced osteogenic capability. Chin J Mech Eng Addit Manuf Front. 2022;1(2):100026. doi: 10.1016/j.cjmeam.2022.100026
- Han X, Sun M, Chen B, et al. Lotus seedpod-inspired internal vascularized 3D printed scaffold for bone tissue repair. Bioact Mater. 2021;6:1639-1652. doi: 10.1016/j.bioactmat.2020.11.019
- Yan Y, Chen H, Zhang H, et al. Vascularized 3D printed scaffolds for promoting bone regeneration. Biomaterials. 2019;190:97-110. doi: 10.1016/j.biomaterials.2018.10.033
- Li Z, Wang S, Xu G, Hu X, Han L, Zhao Y. Synergy effect of Sr and rhBMP-2: a potential solution to osteolysis caused by rhBMP-2. Med Hypotheses. 2020;144:109895. doi: 10.1016/j.mehy.2020.109895
- Yi Q, Liang P, Liang D, Shi J-F, Sha S, Chang Q. Multifunction Sr doped microporous coating on pure magnesium of antibacterial, osteogenic and angiogenic activities. Ceram Int. 2021;47:8133-8141. doi: 10.1016/j.ceramint.2020.11.168
- Chen A, Su J, Li Y, et al. 3D/4D printed bio-piezoelectric smart scaffolds for next-generation bone tissue engineering. Int J Extreme Manuf. 2023;5(3):032007. doi: 10.1088/2631-7990/acd88f
- Lee NH, Kang MS, Kim TH, et al. Dual actions of osteoclastic-inhibition and osteogenic-stimulation through strontium-releasing bioactive nanoscale cement imply biomaterial-enabled osteoporosis therapy. Biomaterials. 2021;276:121025. doi: 10.1016/j.biomaterials.2021.121025
- Sun T, Li Z, Zhong X, et al. Strontium inhibits osteoclastogenesis by enhancing LRP6 and β-catenin-mediated OPG targeted by miR-181d-5p. J Cell Commun Signal. 2019;13:85-97. doi: 10.1007/s12079-018-0478-y
- Wu Q, Hu L, Yan R, et al. Strontium-incorporated bioceramic scaffolds for enhanced osteoporosis bone regeneration. Bone Res. 2022;10(1):55. doi: 10.1038/s41413-022-00224-x
- Jiang S, Wang X, Ma Y, et al. Synergistic effect of micro-nano-hybrid surfaces and Sr doping on the osteogenic and angiogenic capacity of hydroxyapatite bioceramics scaffolds. Int J Nanomed. 2022;17:783-797. doi: 10.2147/IJN.S345357
- Quade M, Vater C, Schlootz S, et al. Strontium enhances BMP‐2 mediated bone regeneration in a femoral murine bone defect model. J Biomed Mater Res B. 2020;108(1):174-182. doi: 10.1002/jbm.b.34376
- Cheng D, Liang Q, Li Y, et al. Strontium incorporation improves the bone-forming ability of scaffolds derived from porcine bone. Colloids Surf B Biointerfaces. 2018; 162:279-287. doi: 10.1016/j.colsurfb.2017.11.070
- Meininger S, Mandal S, Kumar A, Groll J, Basu B, Gbureck U. Strength reliability and in vitro degradation of three-dimensional powder printed strontium-substituted magnesium phosphate scaffolds. Acta Biomater. 2016; 31:401-411. doi: 10.1016/j.actbio.2015.11.050
- Denry I, Goudouri OM, Fredericks DC, Akkouch A, Acevedo MR, Holloway JA. Strontium-releasing fluorapatite glass-ceramic scaffolds: structural characterization and in vivo performance. Acta Biomater. 2018;75: 463-471. doi: 10.1016/j.actbio.2018.05.047