Additive-manufactured synthetic bone model with biomimicking tunable mechanical properties for evaluation of medical implants
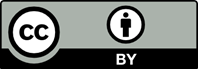
Additive manufacturing has enabled the customization of biomedical systems, including transplantable medical devices, to achieve mechanical biocompatibility. For bone implants, patient-specific bone models must be used to evaluate the mechanical properties of implant compression and subsidence. This study proposes a methodology for designing and fabricating bone models to evaluate patient-specific bone implants. The method involves three-dimensional printing of infill-varied structure, with alternating high-low-high infill density regions, which undergo sequential deformation from the surficial region during compression with an implant. Based on this deformation behavior, the relationship between infill density parameters and mechanical properties was confirmed with the tunability of mechanical properties involving stiffness and failure load. The infill-varied design was applied to the inner structures of artificial vertebra models based on computed tomography scans for cadaver specimens. By tailoring the infill density conditions, the stiffness and failure load were approximated to those of the natural vertebrae. Furthermore, this infill-varied artificial vertebra could be used to evaluate additive-manufactured patient-specific implants. The patient-specific implant had greater resistance to subsidence than the commercial implant, suggesting the feasibility of a biomimicking bone model. The bone-mimetic infill-varied structure could be used to evaluate patient-specific manufactured implants and could be applied to other bone engineering structures with optimized biomechanical properties.
- Daminabo SC, Goel S, Grammatikos SA, Nezhad HY, Thakur VK. Fused deposition modeling-based additive manufacturing (3D printing): Techniques for polymer material systems. Mater Today Chem. 2020;16:100248. doi: 10.1016/j.mtchem.2020.100248
- Peng X, Kuang X, Roach DJ, et al. Integrating digital light processing with direct ink writing for hybrid 3D printing of functional structures and devices. Addit Manuf. 2021;40:101911. doi: 10.1016/j.addma.2021.101911
- Mitra I, Bose S, Dernell WS, et al. 3D printing in alloy design to improve biocompatibility in metallic implants. Mater Today. 2021;45:20-34. doi: 10.1016%2Fj.mattod.2020.11.021
- Kim H, Lin Y, Tseng TLB. A review on quality control in additive manufacturing. Rapid Prototyp J. 2018;24(3). doi: 10.1108/RPJ-03-2017-0048
- Andreu A, Kim S, Dittus J, et al. Hybrid material extrusion 3D printing to strengthen interlayer adhesion through hot rolling. Addit Manuf. 2022;55:102773. doi: 10.1016/j.addma.2022.102773
- Kan WH, Jiang D, Humbert M. Effect of in-situ layer-by-layer rolling on the microstructure, mechanical properties, and corrosion resistance of a directed energy deposited 316L stainless steel. Addit Manuf. 2022;55:102863. doi: 10.1016/j.addma.2022.102863
- Lee JE, Park SJ, Son Y, Park K, Park S-H. Mechanical reinforcement of additive-manufactured constructs using in situ auxiliary heating process. Addit Manuf. 2021;43: 101995. doi: 10.1016/j.addma.2021.101995
- Park SJ, Park SJ, Son Y, Hyuk Ahn I. Reducing anisotropy of a part fabricated by material extrusion via warm isostatic pressure (WIP) process. Addit Manuf. 2022;55:102841. doi: 10.1016/j.addma.2022.102841
- Park SJ, Lee JE, Park J, Lee N-K, Son Y, Park S-H. High-temperature 3D printing of polyetheretherketone products: Perspective on industrial manufacturing applications of super engineering plastics. Mater Design. 2021;211:110163. doi: 10.1016/j.matdes.2021.110163
- Zhu J, Zhou H, Wang C, et al. A review of topology optimization for additive manufacturing: Status and challenges. Chinese J Aeronaut. 2021;34(1):91-110. doi 10.1016/j.cja.2020.09.020
- Wang P, Song J, Nai MLS, Wei J. Experimental analysis of additively manufactured component and design guidelines for lightweight structures: A case study using electron beam melting. Addit Manuf. 2020;33:101088. doi: 10.1016/j.addma.2020.101088
- Springer H, Baron C, Mostaghimi F, Poveleit J, Mädler L, Uhlenwinkel V. Additive manufacturing of high modulus steels: New possibilities for lightweight design. Addit Manuf. 2020;32:101033. doi: 10.1016/j.addma.2019.101033
- Chen W, Watts S, Jackson JA, Smith WL, Tortorelli DA. Spadaccini CM. Stiff isotropic lattices beyond the Maxwell criterion. Sci Adv. 2019;5(9):eaaw1937. doi: 10.1126/sciadv.aaw1937
- Gobbi SJ, Gobbi VJ, Rocha Y. Requirements for selection/ development of a biomaterial. Biomed J Sci Tech Res. 2019;14(3):1-6. doi: 10.26717/BJSTR.2019.14.002554
- Banse X, Sims TJ, Bailey AJ. Mechanical properties of adult vertebral cancellous bone: Correlation with collagen intermolecular cross‐links. J Bone Miner Res. 2002;17(3):1621-1628. doi: 10.1359/jbmr.2002.17.9.1621
- Roy ME, Rho JY, Tsui TY, Evans ND, Pharr GM. Mechanical and morphological variation of the human lumbar vertebral cortical and trabecular bone. J Biomed Mater Res. 1999;44(2):191-197. doi: 10.1002/(sici)1097-4636(199902)44:2%3C191::aid-jbm9%3E3.0.co;2-g
- Gu XN, Zheng YF. A review on magnesium alloys as biodegradable materials. Front Mater Sci China. 2010;4:111. doi: 10.1007/s11706-010-0024-1
- Ma H, Suonan A, Zho J, et al. PEEK (Polyether-ether-ketone) and its composite materials in orthopedic implantation. Arab J Chem. 2021;14(3):102977. doi: 10.1016/j.arabjc.2020.102977
- Parthasarathy J, Starly B, Raman S, Christensen A. Mechanical evaluation of porous titanium (Ti6Al4V) structures with electron beam melting (EBM). J Mech Behav Biomed Mater. 2010;3(3):249-259. doi: 10.1016/j.jmbbm.2009.10.006
- Ghouse S, Reznikov N, Boughton OR. The design and in vivo testing of a locally stiffness-matched porous scaffold. Appl Mater Today. 2019;15:377-388. doi: 10.1016/j.apmt.2019.02.017
- Barba D, Alabort E, Reed RC. Synthetic bone: Design by additive manufacturing. Acta Biomater. 2019;97: 637-656. doi: 10.1016/j.actbio.2019.07.049
- Su R, Chen J, Zhang X, et al. 3D‐printed micro/nano‐scaled mechanical metamaterials: Fundamentals, technologies, progress, applications, and challenges. Small. 2023;19(29):2206391. doi: 10.1002/smll.202206391
- Distefano F, Pasta S, Epasto G. Titanium lattice structures produced via additive manufacturing for a bone scaffold: A review. J Funct Biomater. 2023;14(3):125. doi: 10.3390/jfb14030125
- Zhou Q, Su X, Wu J, et al. Additive manufacturing of bioceramic implants for restoration bone engineering: Technologies, advances, and future perspectives. ACS Biomater Sci Eng. 2023;9(3):1164-1189. doi: 10.1021/acsbiomaterials.2c01164
- Jiao C, Xie D, He Z, et al. Additive manufacturing of bio-inspired ceramic bone scaffolds: Structural design, mechanical properties and biocompatibility. Mater Design. 2022;217:110610. doi: 10.1016/j.matdes.2022.110610
- Yang Y, Wang G, Liang H, et al. Additive manufacturing of bone scaffolds. Int J Bioprint. 2019;5(1):148. doi: 10.18063%2FIJB.v5i1.148
- Kramschuster A, Turng LS. Fabrication of tissue engineering scaffolds. Handbook of Biopolymers and Biodegradable Plastics: Properties, Processing and Applications. 2012; 427-446. doi: 10.1016/B978-1-4557-2834-3.00017-3
- Arifvianto B, Zhou J. Fabrication of metallic biomedical scaffolds with the space holder method: A review. Materials. 2014;7(5):3588-3622. doi: 10.3390/ma7053588
- Lowe TG, Hashim S, Wilson LA. A biomechanical study of regional endplate strength and cage morphology as it relates to structural interbody support. Spine. 2004;29(21): 2389-2394. doi: 10.1097/01.brs.0000143623.18098.e5
- James H. Use of cadavers to train surgeons: What are the ethical issues? J Med Ethics. 2020;46:470-471. doi: 10.1136/medethics-2019-105873
- ASTM F1839-08. Standard Specification for Rigid Polyurethane Foam for Use as a Standard Material for Testing Orthopaedic Devices and Instruments. ASTM International, West Conshohocken, PA. 2012. doi: 10.1520/F1839-08R21
- American Society for Testing and Materials. Standard Test Methods for Measuring Load Induced Subsidence of Intervertebral Body Fusion Device Under Static Axial Compression. ASTM International, West Conshohocken, PA. 2011. doi: 10.1520/F2267-22
- Closkey RF, Parsons JR, Lee CK, M F Blacksin, Zimmerman MC. Mechanics of interbody spinal fusion. Analysis of critical bone graft area. Spine. 1993;18(8):1011-1015. doi: 10.1097/00007632-199306150-00010
- Lim TH, Kwon H, Jeon CH, et al. Effect of endplate conditions and bone mineral density on the compressive strength of the graft–endplate interface in anterior cervical spine fusion. Spine. 2001;26(8):951-956. doi: 10.1097/00007632-200104150-00021
- Grant JP, Oxland TR, Dvorak MF. Mapping the structural properties of the lumbosacral vertebral endplates. Spine. 2001;26(8):889-896. doi: 10.1097/00007632-200104150-00012
- Hakato J, Pezowicz C, Wronski J, Bedziński R, Kasprowicz M. The process of subsidence after cervical stabilizations by cage alone, cage with plate and plate-cage. A biomechanical comparative study. Neurol Neurochir Pol. 2007;41(5):411.
- Marulanda GA, Nayak A, Murtagh R, Brandon G Santoni, Billys JB, Castellvi AE. A cadaveric radiographic analysis on the effect of extreme lateral interbody fusion cage placement with supplementary internal fixation on indirect spine decompression. Clin Spine Surg. 2014;27(5):263-270. doi: 10.1097/bsd.0b013e31828f9da1
- Schumacher Y. Comparison of two loading surface preparation methods on rat vertebral bodies for compression testing. Queen’s University (Canada). 2013. http://hdl.handle.net/1974/8359
- Collino RR, Kiapour A, Begley MR. Subsidence of additively-manufactured cages in foam substrates: effect of contact topology. J Biomech Eng. 2020;142(9):091003. doi: 10.1115/1.4046584
- Flores-Johnson EA, Li QM. Indentation into polymeric foams. Int J Solid Structur. 2010;47(16):1987-1995. doi: 10.1016/j.ijsolstr.2010.03.025
- Swan CC, Kosaka I. Voigt–Reuss topology optimization for structures with nonlinear material behaviors. Int J Numer Methods Eng. 1997;40(20):3785-3814. Doi: 10.1002/(SICI)1097-0207(19971030)40:20%3C3785::AID-NME240%3E3.0.CO;2-v
- Hosseini HS, Clouthier AL, Zysset PK. Experimental validation of finite element analysis of human vertebral collapse under large compressive strains. J Biomech Eng. 2014;136(4):041006. doi: 10.1115/1.4026409
- Bouzakis KD, Mitsi S, Michailidis N, et al. Loading simulation of lumbar spine vertebrae during a compression test using the finite elements method and trabecular bone strength properties, determined by means of nanoindentations. J Musculoskelet Neuronal Interact. 2004;4(2):152-158.
- Kurutz M, Donáth J, Gálos M, Varga Péter, Fornet B. Age-and sex-related regional compressive strength characteristics of human lumbar vertebrae in osteoporosis. J Multidiscip Healthc. 2008;1:105-121. doi: 10.2147/jmdh.s4103
- de Beer N, Scheffer C. Reducing subsidence risk by using rapid manufactured patient-specific intervertebral disc implants. Spine J. 2012;12(11):1060-1066. doi: 10.1016/j.spinee.2012.10.003
- Choudhury S, Raja D, Roy S, Datta S. Stress analysis of different types of cages in cervical vertebrae: A finite element study. IOP Conf Ser: Mater Sci Eng. 2020;912:022025. doi: 10.1088/1757-899X/912/2/022025
- 47. Cadman J, Sutterlin III C, Dabirrahmani D, Appleyard R. 2016; The importance of loading the periphery of the vertebral endplate. J Spine Surg. 2(3):178. doi: 10.21037/jss.2016.09.08