Demystifying the influence of ferroptosis on Alzheimer’s and Parkinson’s diseases: A network and systems biology approach
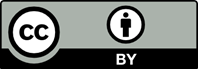
Research into the pathophysiology of Alzheimer’s disease (AD) and Parkinson’s disease (PD) has spanned decades, unraveling deregulated signaling cascades in these diseases. Recently, the discovery of the link between ferroptosis and neurodegeneration has opened new avenues for neurodegenerative disease research. Despite this, the key players in the ferroptotic pathway potentially governing the progression of neurodegenerative disease remain unidentified. Thus, in the present study, we reconstructed two protein–protein interaction networks (PPINs) for AD and PD with their respective differentially expressed genes from post-mortem tissues and identified 21 highly connected clusters within the AD PPIN and 17 clusters within the PD PPIN. Then, we identified 8 ferroptotic transcription factors (FerrTFs) that regulate hub genes from the 7 deregulated clusters of AD and 6 FerrTFs from the 4 deregulated clusters of PD. Functional enrichment analysis of these clusters revealed impairment in important neurological functions. Finally, we identified 681 drugs with potential therapeutic effects against the 8 FerrTFs associated with AD and 633 drugs against the 6 FerrTFs linked to PD. In addition, 126 and 114 miRNAs might silence 7 and 5 FerrTFs against AD and PD, respectively. This exploratory study identifies potential markers of ferroptosis that could exacerbate these neurodegenerative diseases and also suggests possible therapeutic measures against them.
- Sun Y, Chen P, Zhai B, et al., 2020, The emerging role of ferroptosis in inflammation. Biomed Pharmacother, 127: 110108. https://doi.org/10.1016/j.biopha.2020.110108
- Galaris D, Barbouti A, Pantopoulos K, 2019, Iron homeostasis and oxidative stress: An intimate relationship. Biochim Biophys Acta Mol Cell Res, 1866: 118535. https://doi.org/10.1016/j.bbamcr.2019.118535
- Reichert CO, de Freitas FA, Sampaio-Silva J, et al., 2020, Ferroptosis mechanisms involved in neurodegenerative diseases. Int J Mol Sci, 21: 8765. https://doi.org/10.3390/ijms21228765
- Xu Y, Zhao J, Zhao Y, et al., 2023, The role of ferroptosis in neurodegenerative diseases. Mol Biol Rep, 50: 1655–1661. https://doi.org/10.1007/s11033-022-08048-y
- Chen X, Yu C, Kang R, et al., 2020, Iron metabolism in ferroptosis. Front Cell Dev Biol, 8: 590226. https://doi.org/10.3389/fcell.2020.590226
- Munshi C, Bhattacharya S, 2022, The “irony” of ferroptosis: A review on neurological challenges. In: Xu K, editor. Cell Death and Disease. Rijeka: IntechOpen. https://doi.org/10.5772/intechopen.108737
- Ou M, Jiang Y, Ji Y, et al., 2022, Role and mechanism of ferroptosis in neurological diseases. Mol Metab, 61: 101502. https://doi.org/10.1016/j.molmet.2022.101502
- Yan H, Zou T, Tuo Q, et al., 2021, Ferroptosis: Mechanisms and links with diseases. Signal Transduct Target Ther, 6: 49. https://doi.org/10.1038/s41392-020-00428-9
- Ma H, Dong Y, Chu Y, et al., 2022, The mechanisms of ferroptosis and its role in Alzheimer’s disease. Front Mol Biosci, 9: 965064. https://doi.org/10.3389/fmolb.2022.965064
- Ward RJ, Zucca FA, Duyn JH, et al., 2014, The role of iron in brain ageing and neurodegenerative disorders. Lancet Neurol, 13: 1045–1060. https://doi.org/10.1016/S1474-4422(14)70117-6
- Zhang Y, Wang M, Chang W, 2022, Iron dyshomeostasis and ferroptosis in Alzheimer’s disease: Molecular mechanisms of cell death and novel therapeutic drugs and targets for AD. Front Pharmacol, 13: 983623. https://doi.org/10.3389/fphar.2022.983623
- Yang WS, Kim KJ, Gaschler MM, et al., 2016, Peroxidation of polyunsaturated fatty acids by lipoxygenases drives ferroptosis. Proc Natl Acad Sci U S A, 113: E4966–E4975. https://doi.org/10.1073/pnas.1603244113
- Chen K, Jiang X, Wu M, et al., 2021, Ferroptosis, a potential therapeutic target in Alzheimer’s disease. Front Cell Dev Biol, 9: 704298. https://doi.org/10.3389/fcell.2021.704298
- Chen JJ, Thiyagarajah M, Song J, et al., 2022, Lanctôt, altered central and blood glutathione in Alzheimer’s disease and mild cognitive impairment: A meta-analysis. Alzheimers Res Ther, 14: 23. https://doi.org/10.1186/s13195-022-00961-5
- Kuang F, Liu J, Tang D, et al., 2020, Oxidative damage and antioxidant defense in ferroptosis. Front Cell Dev Biol, 8: 586578. https://doi.org/10.3389/fcell.2020.586578
- Guzman-Martinez L, Maccioni RB, Andrade V, et al., 2019, Neuroinflammation as a common feature of neurodegenerative disorders. Front Pharmacol, 10: 1008. https://doi.org/10.3389/fphar.2019.01008
- Jankovic J, Tan EK, Parkinson’s disease: Etiopathogenesis and treatment. J Neurol Neurosurg Psychiatry, 91: 795–808. https://doi.org/10.1136/jnnp-2019-322338
- Fernández-Mendívil C, Luengo E, Trigo-Alonso P, et al., 2021, Protective role of microglial HO-1 blockade in aging: Implication of iron metabolism. Redox Biol, 38: 101789. https://doi.org/10.1016/j.redox.2020.101789
- Zhu Y, Wang B, Tao K, et al., 2017, Iron accumulation and microglia activation contribute to substantia nigra hyperechogenicity in the 6-OHDA-induced rat model of Parkinson’s disease. Parkinsonism Relat Disord, 36: 76–82. https://doi.org/10.1016/j.parkreldis.2017.01.003
- Novgorodov SA, Voltin JR, Gooz MA, et al., 2018, Acid sphingomyelinase promotes mitochondrial dysfunction due to glutamate-induced regulated necrosis. J Lipid Res, 59: 312–329. https://doi.org/10.1194/jlr.M080374
- Guennewig B, Lim J, Marshall L, et al., 2021, Defining early changes in Alzheimer’s disease from RNA sequencing of brain regions differentially affected by pathology. Sci Rep, 11: 4865. https://doi.org/10.1038/s41598-021-83872-z
- Robinson MD, McCarthy DJ, Smyth GK, 2010, edgeR: A bioconductor package for differential expression analysis of digital gene expression data. Bioinformatics, 26: 139–140. https://doi.org/10.1093/bioinformatics/btp616
- Szklarczyk D, Gable AL, Nastou KC, et al., 2020, The STRING database in 2021: Customizable protein-protein networks, and functional characterization of user-uploaded gene/measurement sets. Nucleic Acids Res, 49: D605–D612. https://doi.org/10.1093/nar/gkaa1074
- Shannon P, Markiel A, Ozier O, et al., 2003, Cytoscape: A software environment for integrated models. Genome Res, 13: 2498–2504. https://doi.org/10.1101/gr.1239303.metabolite
- Bader GD, Hogue CW, 2003, An automated method for finding molecular complexes in large protein interaction networks. BMC Bioinformatics, 4: 2. https://doi.org/10.1186/1471-2105-4-2
- Maere S, Heymans K, Kuiper M, 2005, BiNGO: A cytoscape plugin to assess overrepresentation of gene ontology categories in biological networks. Bioinformatics, 21: 3448–3449. https://doi.org/10.1093/bioinformatics/bti551
- Assenov Y, Ramírez F, Schelhorn SE, et al., 2008, Computing topological parameters of biological networks. Bioinformatics, 24: 282–284. https://doi.org/10.1093/bioinformatics/btm554
- Zhou N, Bao J, 2020, FerrDb: A manually curated resource for regulators and markers of ferroptosis and ferroptosis-disease associations. Database, 2020: baaa021. https://doi.org/10.1093/database/baaa021
- Han H, Cho JW, Lee S, et al., 2018, TRRUST v2: An expanded reference database of human and mouse transcriptional regulatory interactions. Nucleic Acids Res, 46: D380–D386. https://doi.org/10.1093/nar/gkx1013
- Liu ZP, Wu C, Miao H, et al., 2015, RegNetwork: An integrated database of transcriptional and post-transcriptional regulatory networks in human and mouse. Database (Oxford), 2015: bav095. https://doi.org/10.1093/database/bav095
- Yoo M, Shin J, Kim J, et al., 2015, DSigDB: Drug signatures database for gene set analysis. Bioinformatics, 31: 3069–3071. https://doi.org/10.1093/bioinformatics/btv313
- Lelos M, 2020, Chapter 2 Overview of Alzheimer’s and Parkinson’s diseases and the role of protein aggregation in these neurodegenerative diseases. In: Salgado AJ, editor. Handbook of Innovations in Central Nervous System Regenerative Medicine. Amsterdam: Elsevier, p29–53. https://doi.org/10.1016/B978-0-12-818084-6.00002-7
- Kajiwara Y, McKenzie A, Dorr N, et al., 2016, The human-specific CASP4 gene product contributes to Alzheimer-related synaptic and behavioural deficits. Hum Mol Genet, 25: 4315–4327. https://doi.org/10.1093/hmg/ddw265
- Gambuzza ME, Sofo V, Salmeri FM, et al., 2014, Toll-like receptors in Alzheimer’s disease: A therapeutic perspective. CNS Neurol Disord Drug Targets, 13: 1542–1558. https://doi.org/10.2174/1871527313666140806124850
- Hernandez MX, Namiranian P, Nguyen E, et al., 2017, C5a increases the injury to primary neurons elicited by fibrillar amyloid beta. ASN Neuro, 9. https://doi.org/10.1177/1759091416687871
- Boros FA, Vécsei L, Klivényi P, 2021, NEAT1 on the field of Parkinson’s disease: Offense, defense, or a player on the bench? J Parkinsons Dis, 11: 123–138. https://doi.org/10.3233/jpd-202374
- Venero JL, Burguillos MA, Brundin P, et al., 2011, The executioners sing a new song: Killer caspases activate microglia. Cell Death Differ, 18: 1679–1691. https://doi.org/10.1038/cdd.2011.107
- Halbgebauer S, Nagl M, Klafki H, et al., 2016, Modified serpinA1 as risk marker for Parkinson’s disease dementia: Analysis of baseline data. Sci Rep, 6: 26145. https://doi.org/10.1038/srep26145
- Peng X, Wang J, Peng W, et al., 2017, Protein-protein interactions: Detection, reliability assessment and applications. Brief Bioinform, 18: 798–819. https://doi.org/10.1093/bib/bbw066
- Szklarczyk D, Gable AL, Lyon D, et al., 2019, STRING v11: Protein-protein association networks with increased coverage, supporting functional discovery in genome-wide experimental datasets. Nucleic Acids Res, 47: D607–D613. https://doi.org/10.1093/nar/gky1131
- Do Van B, Gouel F, Jonneaux A, et al., 2016, Ferroptosis, a newly characterized form of cell death in Parkinson’s disease that is regulated by PKC. Neurobiol Dis, 94: 169–178. https://doi.org/10.1016/j.nbd.2016.05.011
- Han X, Zhu J, Zhang X, et al., 2018, Plin4-dependent lipid droplets hamper neuronal mitophagy in the MPTP/p-induced mouse model of Parkinson’s disease. Front Neurosci, 12: 397. https://doi.org/10.3389/fnins.2018.00397
- Zhang X, Du L, Qiao Y, et al., 2019, Ferroptosis is governed by differential regulation of transcription in liver cancer. Redox Biol, 24: 101211. https://doi.org/10.1016/j.redox.2019.101211
- Song J, Liu Y, Guan X, et al., 2021, A novel ferroptosis-related biomarker signature to predict overall survival of esophageal squamous cell carcinoma. Front Mol Biosci, 8: 675193. https://doi.org/10.3389/fmolb.2021.675193
- Visser PJ, Reus LM, Gobom J, et al., 2022, Cerebrospinal fluid tau levels are associated with abnormal neuronal plasticity markers in Alzheimer’s disease. Mol. Neurodegener, 17: 27. https://doi.org/10.1186/s13024-022-00521-3
- Liao S, Huang M, Liao Y, et al., 2023, HMOX1 promotes ferroptosis induced by erastin in lens epithelial cell through modulates Fe(2+) production. Curr Eye Res, 48: 25–33. https://doi.org/10.1080/02713683.2022.2138450
- Zhou Z, Lu J, Ma J, et al., 2022, Identification of potential ferroptosis key genes in the pathogenesis of lumbosacral spinal root avulsion by RNA sequencing and bioinformatics analysis. Front Mol Biosci, 9: 902607. https://doi.org/10.3389/fmolb.2022.902607
- Cressatti M, Song W, Turk AZ, et al., 2019, Glial HMOX1 expression promotes central and peripheral α-synuclein dysregulation and pathogenicity in parkinsonian mice. Glia, 67: 1730–1744. https://doi.org/10.1002/glia.23645
- Lan G, Wang P, Chan RB, et al., 2022, Astrocytic VEGFA: An essential mediator in blood-brain-barrier disruption in Parkinson’s disease. Glia, 70: 337–353. https://doi.org/10.1002/glia.24109
- KaoYC, Ho PC, Tu YK, et al., 2020, Lipids and Alzheimer’s disease. Int J Mol Sci, 21: 1505. https://doi.org/10.3390/ijms21041505
- Lin Z, Liu J, Kang R, et al., 2021, Lipid metabolism in ferroptosis. Adv Biol, 5: e2100396. https://doi.org/10.1002/adbi.202100396
- Tang D, Chen X, Kang R, et al., 2021, Ferroptosis: Molecular mechanisms and health implications. Cell Res, 31: 107–125. https://doi.org/10.1038/s41422-020-00441-1
- Tan JM, Wong ES, Lim KL, 2009, Protein misfolding and aggregation in Parkinson’s disease. Antioxid Redox Signal, 11: 2119–2134. https://doi.org/10.1089/ars.2009.2490
- Pitale PM, Gorbatyuk O, Gorbatyuk M, 2017, Neurodegeneration: Keeping ATF4 on a tight leash. Front Cell Neurosci, 11: 410. https://doi.org/10.3389/fncel.2017.00410
- Xu X, Huang E, Tai Y, et al., 2017, Nupr1 modulates methamphetamine-induced dopaminergic neuronal apoptosis and autophagy through CHOP-Trib3-mediated endoplasmic reticulum stress signaling pathway. Front Mol Neurosci, 10: 203. https://doi.org/10.3389/fnmol.2017.00203
- Santiago JA, Potashkin JA, 2015, Network-based metaanalysis identifies HNF4A and PTBP1 as longitudinally dynamic biomarkers for Parkinson’s disease. Proc Natl Acad Sci U S A, 112: 2257–2262. https://doi.org/10.1073/pnas.1423573112
- Hernandes MS, Lassègue B, Yepes M, et al., 2016, Abstract TP110: Polymerase δ-interacting protein 2 regulates astrocyte activation in ischemic stroke. Stroke, 47: ATP110. https://doi.org/10.1161/str.47.suppl\_1.tp110
- Poonaki E, Kahlert UD, Meuth SG, et al., 2022, The role of the ZEB1-neuroinflammation axis in CNS disorders. J Neuroinflammation, 19: 275. https://doi.org/10.1186/s12974-022-02636-2
- Liu CC, Liu CC, Kanekiyo T, et al., 2013, Apolipoprotein E and Alzheimer disease: Risk, mechanisms and therapy. Nat Rev Neurol, 9: 106–118. https://doi.org/10.1038/nrneurol.2012.263
- Mathys H, Davila-Velderrain J, Peng Z, et al., 2019, Single-cell transcriptomic analysis of Alzheimer’s disease. Nature, 570: 332–337. https://doi.org/10.1038/s41586-019-1195-2
- Kahl CR, Means AR, 2003, Regulation of cell cycle progression by calcium/calmodulin-dependent pathways. Endocr Rev, 24: 719–736. https://doi.org/10.1210/er.2003-0008
- Lee SD, Tontonoz P, 2015, Liver X receptors at the intersection of lipid metabolism and atherogenesis. Atherosclerosis, 242: 29–36. https://doi.org/10.1016/j.atherosclerosis.2015.06.042
- Bellucci A, Bubacco L, Longhena F, et al., 2020, Nuclear factor-κB dysregulation and α-synuclein pathology: Critical interplay in the pathogenesis of Parkinson’s disease. Front Aging Neurosci, 12: 68. https://doi.org/10.3389/fnagi.2020.00068
- De Rivero Vaccari J, Mejias NH, Travascio F, 2017, The NLRC4 inflammasome contributes to brain inflammaging. Innov Aging, 1: 978–979. https://doi.org/10.1093/geroni/igx004.3534
- Kamath T, Abdulraouf A, Burris SJ, et al., 2022, Single-cell genomic profiling of human dopamine neurons identifies a population that selectively degenerates in Parkinson’s disease. Nat Neurosci, 25: 588–595. https://doi.org/10.1038/s41593-022-01061-1
- Jembrek MJ, Oršolić N, Mandić L, et al., 2021, Anti-oxidative, anti-inflammatory and anti-apoptotic effects of flavonols: Targeting Nrf2, NF-κB and p53 pathways in neurodegeneration. Antioxidants (Basel, Switzerland), 10: 1628. https://doi.org/10.3390/antiox10101628
- Liu J, Zhang C, Wang J, et al., 2020, The regulation of ferroptosis by tumor suppressor p53 and its pathway. Int J Mol Sci, 21: 8387. https://doi.org/10.3390/ijms21218387
- Li X, Hu Y, 2021, Attribution of NF-κB activity to CHUK/ IKKα-involved carcinogenesis. Cancers (Basel), 13: 1411. https://doi.org/10.3390/cancers13061411
- Krämer OH, Baus D, Knauer SK, et al., 2006, Acetylation of Stat1 modulates NF-kappaB activity. Genes Dev, 20: 473–485. https://doi.org/10.1101/gad.364306
- Li JY, Yao YM, Tian YP, 2021, Ferroptosis: A trigger of proinflammatory state progression to immunogenicity in necroinflammatory disease. Front Immunol, 12: 701163. https://doi.org/10.3389/fimmu.2021.701163
- Abdel-Salam OM, Youness ER, Mohammed NA, et al., 2014, Citric acid effects on brain and liver oxidative stress in lipopolysaccharide-treated mice. J Med Food, 17: 588–598. https://doi.org/10.1089/jmf.2013.0065
- Zhang M, Bian Z, 2021, Alzheimer’s disease and microRNA-132: A widespread pathological factor and potential therapeutic target. Front Neurosci, 15: https://doi.org/10.3389/fnins.2021.687973