Genetic and non-genetic risk factors of idiopathic pulmonary fibrosis: A review
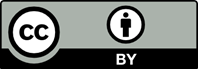
Idiopathic pulmonary fibrosis (IPF) is the most common form of fibrosis of internal organs. The etiology and pathogenesis of IPF are still not well understood. However, a growing line of evidence shows that both genetic and non-genetic factors contribute to IPF development. The release of pro-inflammatory cytokines activates the immune cells. The enhanced synthesis of interleukins and cytokines, especially transforming growth factor β1 leads to the proliferation of fibroblasts, increased extracellular matrix formation, and epithelial-mesenchymal transformation of the lung tissue. These pathological changes could lead to fibrosis. Polymorphisms of genes responsible for the function of mucociliary clearance (MUC5B), telomerases (TERT, TERC), as well as signaling pathway related-genes such as Sonic hedgehog, Wnt, and some other genes are also risk factors for IPF development. Epigenetic regulatory mechanisms, such as methylation and acetylation of DNA and histones, may also influence the development and progression of this disease. At present, the role of non-coding RNAs, in particular long non-coding RNAs (lncRNA) in the development of fibrotic processes, is actively studied. LncRNA is an RNA that is longer than 200 base pairs and does not code for any proteins. LncRNAs perform various functions in the cell, from nuclear compartmentation to epigenetic regulation of gene expression and post-translational modification of proteins. In this review, we present the important aspects in the pathogenesis of IPF.
Wynn TA, Ramalingam TR, 2012, Mechanisms of fibrosis: Therapeutic translation for fibrotic disease. Nat Med, 18: 1028–1040. https://doi.org/10.1038/nm.2807
Rockey DC, Bell PD, Hill JA, 2015, Fibrosis a common pathway to organ injury and failure. N Engl J Med, 372: 1138–1149. https://doi.org/10.1056/NEJMra1300575
Zhao X, Sun J, Chen Y, et al., 2018, LncRNA PFAR promotes lung fibroblast activation and fibrosis by targeting miR-138 to regulate the YAP1-twist axis. Mol Ther, 26: 2206–2217. https://doi.org/10.1016/j.ymthe.2018.06.020
Yan W, Wu Q, Yao W, et al., 2017, MiR-503 modulates epithelial-mesenchymal transition in silica-induced pulmonary fibrosis by targeting PI3K p85 and is sponged by lncRNA MALAT1. Sci Rep, 7: 11313. https://doi.org/10.1038/s41598-017-11904-8
Richeldi L, Collard HR, Jones MG, 2017, Idiopathic pulmonary fibrosis. Lancet, 389:1941–1952. https://doi.org/10.1016/S0140-6736(17)30866-8
Lederer DJ, Martinez FJ, 2018, Idiopathic pulmonary fibrosis. N Engl J Med, 378: 1811–1823. https://doi.org/10.1056/NEJMra1705751
Martinez FJ, Collard HR, Pardo A, et al., 2017, Idiopathic pulmonary fibrosis. Nat Rev Dis Primers, 3: 17074. https://doi.org/10.1038/nrdp.2017.74
Hirahara K, Aoki A, Morimoto Y, et al., 2019, The immunopathology of lung fibrosis: Amphiregulin-producing pathogenic memory T helper-2 cells control the airway fibrotic responses by inducing eosinophils to secrete osteopontin. Semin Immunopathol, 41: 339–348. https://doi.org/10.1007/s00281-019-00735-6
Henderson NC, Rieder F, Wynn TA, 2020, Fibrosis: From mechanisms to medicines. Nature, 587: 555–566. https://doi.org/10.1038/s41586-020-2938-9
Chanda D, Otoupalova E, Smith SR, et al., 2019, Developmental pathways in the pathogenesis of lung fibrosis. Mol Aspects Med, 65: 56–69. https://doi.org/10.1016/j.mam.2018.08.004
Zhu L, Fu X, Chen X, et al., 2017, M2 macrophages induce EMT through the TGF-β/Smad2 signaling pathway. Cell Biol Int, 41: 960–968. https://doi.org/10.1002/cbin.10788
Kugler MC, Joyner AL, Loomis CA, et al., 2015, Sonic hedgehog signaling in the lung. From development to disease. Am J Respir Cell Mol Biol, 52: 1-13. https://doi.org/10.1165/rcmb.2014-0132TR
Yang IV, Pedersen BS, Rabinovich E, et al., 2014, Relationship of DNA methylation and gene expression in idiopathic pulmonary fibrosis. Am J Respir Crit Care Med, 190: 1263–1272. https://doi.org/10.1164/rccm.201408-1452OC
Bechtel W, McGoohan S, Zeisberg EM, et al., 2010, Methylation determines fibroblast activation and fibrogenesis in the kidney. Nat Med, 16: 544–550. https://doi.org/10.1038/nm.2135
Xu X, Tan X, Tampe B, et al., 2015, Epigenetic balance of aberrant Rasal1 promoter methylation and hydroxymethylation regulates cardiac fibrosis. Cardiovasc Res, 105: 279–291. https://doi.org/10.1093/cvr/cvv015
Pastore F, Bhagwat N, Pastore A, et al., 2020, PRMT5 inhibition modulates E2F1 methylation and gene-regulatory networks leading to therapeutic efficacy in JAK2V617F-mutant MPN. Cancer Discov, 10: 1742–1757. https://doi.org/10.1158/2159-8290.CD-20-0026
Coward WR, Brand OJ, Pasini A, et al., 2018, Interplay between EZH2 and G9a regulates CXCL10 gene repression in idiopathic pulmonary fibrosis. Am J Respir Cell Mol Biol, 58: 449–460. https://doi.org/10.1165/rcmb.2017-0286OC
Wang Y, Zhang L, Huang T, et al., 2022, The methyl- CpG-binding domain 2 facilitates pulmonary fibrosis by orchestrating fibroblast to myofibroblast differentiation. Eur Respir J, 27: 2003697. https://doi.org/10.1183/13993003.03697-2020
Yang IV, Pedersen BS, Rabinovich E, et al., 2014, Relationship of DNA methylation and gene expression in idiopathic pulmonary fibrosis. Am J Respir Crit Care Med, 190: 1263–1272. https://doi.org/10.1164/rccm.201408-1452OC
Sanders YY, Pardo A, Selman M, et al., 2008, Thy-1 promoter hypermethylation: A novel epigenetic pathogenic mechanism in pulmonary fibrosis. Am J Respir Cell Mol Biol, 39: 610–618. https://doi.org/10.1165/rcmb.2007-0322OC
Sun L, Xu A, Li M, et al., 2021, Effect of methylation status of lncRNA-MALAT1 and MicroRNA-146a on pulmonary function and expression level of COX2 in patients with chronic obstructive pulmonary disease. Front Cell Dev Biol, 9: 667624. https://doi.org/10.3389/fcell.2021.667624
Cisneros J, Hagood J, Checa M, et al., 2012, Hypermethylation-mediated silencing of p14(ARF) in fibroblasts from idiopathic pulmonary fibrosis. Am J Physiol Lung Cell Mol Physiol, 303: L295–L303. https://doi.org/10.1152/ajplung.00332.2011
Zhou J, Yi Z, Fu Q, 2019, Dynamic decreased expression and hypermethylation of secreted frizzled-related protein 1 and 4 over the course of pulmonary fibrosis in mice. Life Sci, 218: 241–252. https://doi.org/10.1016/j.lfs.2018.12.041
Elkouris M, Kontaki H, Stavropoulos A, et al., 2016, SET9- mediated regulation of TGF-β signaling links protein methylation to pulmonary fibrosis. Cell Rep, 15: 2733–2744. https://doi.org/10.1016/j.celrep.2016.05.051
Jiang Y, Xiang C, Zhong F, et al., 2021, Histone H3K27 methyltransferase EZH2 and demethylase JMJD3 regulate hepatic stellate cells activation and liver fibrosis. Theranostics, 11: 361–378. https://doi.org/10.7150/thno.46360
Irifuku T, Doi S, Sasaki K, et al., 2016, Inhibition of H3K9 histone methyltransferase G9a attenuates renal fibrosis and retains klotho expression. Kidney Int, 89: 147–157. https://doi.org/10.1038/ki.2015.291
Müller D, Győrffy B, 2022, DNA methylation-based diagnostic, prognostic, and predictive biomarkers in colorectal cancer. Biochim Biophys Acta Rev Cancer, 1877: 188722. https://doi.org/10.1016/j.bbcan.2022.188722
Zhang X, Liu H, Zhou JQ, et al., 2022, Modulation of H4K16Ac levels reduces pro-fibrotic gene expression and mitigates lung fibrosis in aged mice. Theranostics, 12: 530–541. https://doi.org/10.7150/thno.62760
Schuetze KB, McKinsey TA, Long CS, 2014, Targeting cardiac fibroblasts to treat fibrosis of the heart: Focus on HDACs. J Mol Cell Cardiol, 70: 100–107. https://doi.org/10.1016/j.yjmcc.2014.02.015
Li M, Zheng Y, Yuan H, et al., 2017, Effects of dynamic changes in histone acetylation and deacetylase activity on pulmonary fibrosis. Int Immunopharmacol, 52: 272–280. https://doi.org/10.1016/j.intimp.2017.09.020
Zheng Q, Lei Y, Hui S, et al., 2022, HDAC3 promotes pulmonary fibrosis by activating NOTCH1 and STAT1 signaling and up-regulating inflammasome components AIM2 and ASC. Cytokine, 153: 155842. https://doi.org/10.1016/j.cyto.2022.155842
Saito S, Zhuang Y, Suzuki T, et al., 2019, HDAC8 inhibition ameliorates pulmonary fibrosis. Am J Physiol Lung Cell Mol Physiol, 316: L175–L186.https://doi.org/10.1152/ajplung.00551.2017
Kim S, Lim JH, Woo CH, 2013, ERK5 inhibition ameliorates pulmonary fibrosis via regulating Smad3 acetylation. Am J Pathol, 183: 1758–1768. https://doi.org/10.1016/j.ajpath.2013.08.014
Barnes PJ, Adcock IM, Ito K, 2005, Histone acetylation and deacetylation: Importance in inflammatory lung diseases. Eur Respir J, 25: 552–563. https://doi.org/10.1183/09031936.05.00117504
Peng WX, Koirala P, Mo YY, 2017, LncRNA-mediated regulation of cell signaling in cancer. Oncogene, 36: 5661– 5667. https://doi.org/10.1038/onc.2017.184
Zhang P, Wu W, Chen Q, et al., 2019, Non-coding RNAs and their integrated networks. J Integr Bioinform, 16: 20190027. https://doi.org/10.1515/jib-2019-0027
Ferrè F, Colantoni A, Helmer-Citterich M, 2016, Revealing protein-lncRNA interaction. Brief Bioinform, 17: 106–116. https://doi.org/10.1093/bib/bbv031
Hulshoff MS, Del Monte-Nieto G, Kovacic J, et al., 2019, Non-coding RNA in endothelial-to-mesenchymal transition. Cardiovasc Res, 115: 1716–1731. https://doi.org/10.1093/cvr/cvz211
Bridges MC, Daulagala AC, Kourtidis A, 2021, LNCcation: lncRNA localization and function. J Cell Biol, 220: e202009045. https://doi.org/10.1083/jcb.202009045
Wang J, Su Z, Lu S, et al., 2018, LncRNA HOXA-AS2 and its molecular mechanisms in human cancer. Clin Chim Acta, 485: 229–233. https://doi.org/10.1016/j.cca.2018.07.004
Xing C, Sun SG, Yue ZQ, et al., 2021, Role of lncRNA LUCAT1 in cancer. Biomed Pharmacother, 134: 111158. https://doi.org/10.1016/j.biopha.2020.111158
Lu Q, Guo Q, Xin M, 2021, LncRNA TP53TG1 promotes the growth and migration of hepatocellular carcinoma cells via activation of ERK signaling. Noncoding RNA, 7: 52. https://doi.org/10.3390/ncrna7030052
Wang H, Zhang Z, Zhang Y, et al., 2021, Long non-coding RNA TP53TG1 upregulates SHCBP1 to promote retinoblastoma progression by sponging miR-33b. Cell Transplant, 30: 9636897211025223. https://doi.org/10.1177/09636897211025223
Zhang Y, Yang H, Du Y, et al., 2019, Long noncoding RNA TP53TG1 promotes pancreatic ductal adenocarcinoma development by acting as a molecular sponge of microRNA-96. Cancer Sci, 110: 2760–2772. https://doi.org/10.1111/cas.14136
Liu R, Yang X, 2021, LncRNA LINC00342 promotes gastric cancer progression by targeting the miR-545-5p/CNPY2 axis. BMC Cancer, 21: 1163. https://doi.org/10.1186/s12885-021-08829-x
Shen P, Qu L, Wang J, et al., 2021, LncRNA LINC00342 contributes to the growth and metastasis of colorectal cancer via targeting miR-19a-3p/NPEPL1 axis. Cancer Cell Int, 21:105. https://doi.org/10.1186/s12935-020-01705-x
Chen QF, Kong JL, Zou SC, et al., 2019, LncRNA LINC00342 regulated cell growth and metastasis in non-small cell lung cancer via targeting miR-203a-3p. Eur Rev Med Pharmacol Sci, 23: 7408–7418. https://doi.org/10.26355/eurrev_201909_18849
Lv D, Tan L, Ma H, et al., 2020, WITHDRAWN: LINC00342 promotes thyroid carcinoma progression by targeting miR- 384/CHMP5 pathway. Pathol Res Pract, 223: 153272. https://doi.org/10.1016/j.prp.2020.153272
Chen C, Wang X, Liu T, et al., 2020, Overexpression of long non-coding RNA RP11-363E7.4 inhibits proliferation and invasion in gastric cancer. Cell Biochem Funct, 38: 921–931. https://doi.org/10.1002/cbf.3514
Jiang X, Ning Q, 2020, The mechanism of lncRNA H19 in fibrosis and its potential as novel therapeutic target. Mech Ageing Dev, 188: 111243. https://doi.org/10.1016/j.mad.2020.111243
Poulet C, Njock MS, Moermans C, et al., 2020, Exosomal long non-coding RNAs in lung diseases. Int J Mol Sci, 21: 3580. https://doi.org/10.3390/ijms21103580
Xiao T, Zou Z, Xue J, et al., 2021, LncRNA H19-mediated M2 polarization of macrophages promotes myofibroblast differentiation in pulmonary fibrosis induced by arsenic exposure. Environ Pollut, 268: 115810. https://doi.org/10.1016/j.envpol.2020.115810
Zhang S, Chen H, Yue D, et al., 2021, Long non-coding RNAs: Promising new targets in pulmonary fibrosis. J Gene Med, 23: e3318. https://doi.org/10.1002/jgm.3318
Gao Y, Zhang J, Liu Y, et al., 2017, Regulation of TERRA on telomeric and mitochondrial functions in IPF pathogenesis. BMC Pulm Med, 17: 163. https://doi.org/10.1186/s12890-017-0516-1
Li Y, Sun W, Pan H, et al., 2021, LncRNA-PVT1 activates lung fibroblasts via miR-497-5p and is facilitated by FOXM1. Ecotoxicol Environ Saf, 213: 112030.https://doi.org/10.1016/j.ecoenv.2021.112030
Lin S, Zhang R, Xu L, et al., 2020, LncRNA Hoxaas3 promotes lung fibroblast activation and fibrosis by targeting miR-450b-5p to regulate runx1. Cell Death Dis, 11: 706. https://doi.org/10.1038/s41419-020-02889-w
Li X, Yu T, Shan H, et al., 2018, LncRNA PFAL promotes lung fibrosis through CTGF by competitively binding miR- 18a. FASEB J, 32: 5285–5297. https://doi.org/10.1096/fj.201800055R
Savary G, Dewaeles E, Diazzi S, et al., 2019, The long noncoding RNA DNM3OS Is a reservoir of fibromirs with major functions in lung fibroblast response to TGF-β and pulmonary fibrosis. Am J Respir Crit Care Med, 200: 184–198. https://doi.org/10.1164/rccm.201807-1237OC
Yang Y, Tai W, Lu N, et al., 2020, LncRNA ZFAS1 promotes lung fibroblast-to-myofibroblast transition and ferroptosis via functioning as a ceRNA through miR-150-5p/SLC38A1 axis. Aging (Albany, NY), 12: 9085–9102. https://doi.org/10.18632/aging.103176
Li X, Peng C, Zhu Z, et al., 2021, The networks of m6A-SARS-CoV-2 related genes and immune infiltration patterns in idiopathic pulmonary fibrosis. Aging (Albany, NY), 13: 6273–6288. https://doi.org/10.18632/aging.202725
Zhang C, Wu Z, Li JW, et al., 2020, Discharge may not be the end of treatment: Pay attention to pulmonary fibrosis caused by severe COVID-19. J Med Virol, 93: 1378–1386. https://doi.org/10.1002/jmv.26634
George PM, Wells AU, Jenkins RG, 2020, Pulmonary fibrosis and COVID-19: The potential role for antifibrotic therapy. Lancet Respir Med, 8: 807–815. https://doi.org/10.1016/S2213-2600(20)30225-3
Spagnolo P, Kropski JA, Jones MG, et al., 2021, Idiopathic pulmonary fibrosis: Disease mechanisms and drug development. Pharmacol Ther, 222: 107798. https://doi.org/10.1016/j.pharmthera.2020.107798
Glass DS, Grossfeld D, Renna HA, et al., 2022, Idiopathic pulmonary fibrosis: Current and future treatment. Clin Respir J, 16: 84–96. https://doi.org/10.1111/crj.13466
Somogyi V, Chaudhuri N, Torrisi SE, et al., 2019, The therapy of idiopathic pulmonary fibrosis: What is next? Eur Respir Rev, 28: 190021. https://doi.org/10.1183/16000617.0021-2019
Hirani N, MacKinnon AC, Nicol L, et al., 2021, Target inhibition of galectin-3 by inhaled TD139 in patients with idiopathic pulmonary fibrosis. Eur Respir J, 57: 2002559. https://doi.org/10.1183/13993003.02559-2020
Guiot J, Moermans C, Henket M, et al., 2017, Blood biomarkers in idiopathic pulmonary fibrosis. Lung, 195: 273–280. https://doi.org/10.1007/s00408-017-9993-5
Drakopanagiotakis F, Wujak L, Wygrecka M, et al., 2018, Biomarkers in idiopathic pulmonary fibrosis. Matrix Biol, 68–69: 404–421. https://doi.org/10.1016/j.matbio.2018.01.023
Kreuter M, Lee JS, Tzouvelekis A, et al., 2021, Monocyte count as a prognostic biomarker in patients with idiopathic pulmonary fibrosis. Am J Respir Crit Care Med, 204: 74–81. https://doi.org/10.1164/rccm.202003-0669OC