In silico investigation of lipid-based compounds implicated in amyotrophic lateral sclerosis
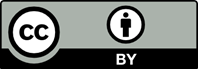
Amyotrophic lateral sclerosis (ALS) is a fatal, currently incurable neurodegenerative disease that progressively affects the upper motor neurons in the brain and lower motor neurons in the spinal cord. A significant portion of patients exhibit abnormalities in lipid metabolism, which appear to contribute to denervation and subsequent motor neuron degeneration. This study aimed to computationally identify lipid-based compounds associated with ALS and elucidate their pathological and therapeutic roles to facilitate effective ALS diagnosis and treatment. The methodologies employed encompassed rational database searches, pharmacokinetic and target predictions, as well as docking and molecular dynamics simulations (MDS). The findings from the disease-to-lipids analysis identified 20 lipid compounds correlated with ALS, primarily categorized as potential inhibitors within the functional classes of vitamins, antibiotics, organic acids, and phytochemicals. Pharmacokinetic predictions revealed that only four compounds exhibited permeability across the blood–brain barrier, namely 4-hydroxynonenal, resveratrol, rotenone, and valproic acid. Docking simulations indicated the highest binding affinity for 4-hydroxynonenal with alkaline ceramidase 2 (−4.516 kcal/mol), resveratrol with carbonic anhydrase 6 (−7.070 kcal/mol), rotenone with cytochrome P450 2C19 (−7.378 kcal/mol), and valproic acid with glutamate carboxypeptidase 2 (−5.629 kcal/mol). Furthermore, MDS and molecular mechanics/generalized born surface area calculations demonstrated the stability and binding energies of the complexes under simulated physiological conditions. In summary, further investigation is warranted to explore the synergistic effects of resveratrol and valproic acid in ALS, the mechanisms underlying 4-hydroxynonenal adduct-assisted aggregate formation of certain enzymes in ALS, and the impact of prolonged human exposure to rotenone from marine food sources (such as fish) on the development of ALS.
- Rowland LP, Shneider NA. Amyotrophic lateral sclerosis. N Engl J Med. 2001;344:1688-1700. doi: 10.1056/NEJM200105313442207
- Yáñez M, Galán L, Matías-Guiu J, Vela A, Guerrero A, García AG. CSF from amyotrophic lateral sclerosis patients produces glutamate independent death of rat motor brain cortical neurons: Protection by resveratrol but not riluzole. Brain Res. 2011;1423:77-86. doi: 10.1016/j.brainres.2011.09.025
- Saez-Atienzar S, Bandres-Ciga S, Langston RG, et al. Genetic analysis of amyotrophic lateral sclerosis identifies contributing pathways and cell types. Sci Adv. 2021;7(3):eabd9036. doi: 10.1126/sciadv.abd9036
- Fatoki TH, Chukwuejim S, Udenigwe CC, Aluko RE. In silico exploration of metabolically active peptides as potential therapeutic agents against amyotrophic lateral sclerosis. Int J Mol Sci. 2023;24(6):5828. doi: 10.3390/ijms24065828
- Gyawali A, Latif S, Choi SH, Hyeon SJ, Ryu H, Kang YS. Monocarboxylate transporter functions and neuroprotective effects of valproic acid in experimental models of amyotrophic lateral sclerosis. J Biomed Sci. 2022;29:2. doi: 10.1186/s12929-022-00785-3
- Desport JC, Preux PM, Magy L, et al. Factors correlated with hypermetabolism in patients with amyotrophic lateral sclerosis. Am J Clin Nutr. 2001;74(3):328-334. doi: 10.1093/ajcn/74.3.328
- Funalot B, Desport JC, Sturtz F, Camu W, Couratier P. High metabolic level in patients with familial amyotrophic lateral sclerosis. Amyotroph Lateral Scler. 2009;10(2):113-117. doi: 10.1080/17482960802295192
- Kwong KCN, Mehta AR, Nedergaard M, Chandran S. Defining novel functions for cerebrospinal fluid in ALS pathophysiology. Acta Neuropathol Commun. 2020;8:140. doi: 10.1186/s40478-020-01018-0
- Wong JK, Roselle AK, Shue TM, et al. Apolipoprotein B-100-mediated motor neuron degeneration in sporadic amyotrophic lateral sclerosis. Brain Commun. 2022;44:fcac207. doi: 10.1093/braincomms/fcac207
- Dupuis L, Pradat PF, Ludolph AC, Loeffler JP. Energy metabolism in amyotrophic lateral sclerosis. Lancet Neurol. 2011;10(1):75-82. doi: 10.1016/S1474-4422(10)70224-6
- D’Amico E, Grosso G, Nieves JW, Zanghì A, Factor-Litvak P, Mitsumoto H. Metabolic abnormalities, dietary risk factors and nutritional management in amyotrophic lateral sclerosis. Nutrients. 2021;13(7):2273. doi: 10.3390/nu13072273
- Dodge JC, Treleaven CM, Pacheco J, et al. Glycosphingolipids are modulators of disease pathogenesis in amyotrophic lateral sclerosis. Proc Natl Acad Sci U S A. 2015;112(26):8100-8105. doi: 10.1073/pnas.1508767112
- Henriques A, Croixmarie V, Priestman DA, et al. Amyotrophic lateral sclerosis and denervation alter sphingolipids and up-regulate glucosylceramide synthase. Hum Mol Genet. 2015;24(25):7390-73405. doi: 10.1093/hmg/ddv439
- Blasco H, Veyrat-Durebex C, Bocca C, et al. Lipidomics reveals cerebrospinal-fluid signatures of ALS. Sci Rep. 2017;7:17652. doi: 10.1038/s41598-017-17389-9
- Chaves-Filho AB, Pinto IFD, Dantas LS, et al. Alterations in lipid metabolism of spinal cord linked to amyotrophic lateral sclerosis. Sci Rep. 2019;9(1):11642. doi: 10.1038/s41598-019-48059-7
- Luikinga S, Henriques A, Ngo ST, et al. Profound lipid dysregulation in mutant TDP-43 mice is ameliorated by the glucocerebrosidase 2 inhibitor ambroxol. BioRxiv Preprint. 2022. doi: 10.1101/2022.08.30.505901
- Keiser MJ, Roth BL, Armbruster BN, Ernsberger P, Irwin JJ, Shoichet BK. Relating protein pharmacology by ligand chemistry. Nat Biotech. 2007;25(2):197-206. doi: 10.1038/nbt1284
- Daina A, Michielin O, Zoete V. SwissADME: A free web tool to evaluate pharmacokinetics, drug-likeness and medicinal chemistry friendliness of small molecules. Sci Rep. 2017;7:42717. doi: 10.1038/srep42717
- Morris SM. Recent advances in arginine metabolism: Roles and regulation of the arginases. Br J Pharmacol. 2009;157:922-930. doi: 10.1111/j.1476-5381.2009.00278.x
- Trott O, Olson AJ. AutoDock Vina: Improving the speed and accuracy of docking with a new scoring function, efficient optimization, and multithreading. J Comput Chem. 2010;31(2):455-461. doi: 10.1002/jcc.21334
- Eberhardt J, Santos-Martins D, Tillack AF, Forli S. AutoDock Vina 1.2.0: New docking methods, expanded force field, and python bindings. J Chem Inf Model. 2021;61(8):3891-3898. doi: 10.1021/acs.jcim.1c00203
- Fatoki T, Chukwuejim S, Ibraheem O, et al. Harmine and 7,8-dihydroxyflavone synergistically suitable for amyotrophic lateral sclerosis management: An in silico study. Res Results Pharmacol. 2022;8(3):49-61. doi: 10.3897/rrpharmacology.8.83332
- Fatoki TH, Faleye BC, Nwagwe OR, et al. Friedelin Could moderately modulate human carbonic anhydrases: An in silico study. Biointerface Res Appl Chem. 2024;14(2):49. doi: 10.33263/BRIAC142.049
- Tao A, Huang Y, Shinohara Y, Caylor ML, Pashikanti S, Xu D. ezCADD: A rapid 2D/3D visualization-enabled web modeling environment for democratizing computer-aided drug design. J Chem Inf Model. 2019;59(1):18-24. doi: 10.1021/acs.jcim.8b00633
- Schrödinger. Schrödinger Release 2018-3. Desmond Molecular Dynamics System: Maestro Desmond Interoperability Tools. New York: D. E. Shaw Research, Schrödinger; 2018.
- Ali I, Iqbal MN, Ibrahim M, Haq IU, Alonazi WB, Siddiqi AR. Computational exploration of novel ROCK2 inhibitors for cardiovascular disease management; insights from high-throughput virtual screening, molecular docking, DFT and MD simulation. PLoS One. 2023;18(11):e0294511. doi: 10.1371/journal.pone.0294511
- Fatoki TH. Effect of pH on structural dynamics of HMG-CoA reductase and binding affinity to β-sitosterol. J Biomol Struct Dyn. 2023;41(10):4398-4404. doi: 10.1080/07391102.2022.2067240
- Schrödinger. What Do All the Prime MM-GBSA Energy Properties Mean ? 2019. Available from: https://www. schrodinger.com/kb/1875 [Last accessed on 2023 Nov 20].
- Novak V, Rogelj B, Župunski V. Therapeutic potential of polyphenols in amyotrophic lateral sclerosis and frontotemporal dementia. Antioxidants (Basel). 2021;10(8):1328. doi: 10.3390/antiox10081328
- Carrera-Juliá S, Moreno ML, Barrios C, de la Rubia Ortí JE, Drehmer E. Antioxidant alternatives in the treatment of amyotrophic lateral sclerosis: A comprehensive review. Front Physiol. 2020;11:63. doi: 10.3389/fphys.2020.00063
- Bono S, Feligioni M, Corbo M. Impaired antioxidant KEAP1-NRF2 system in amyotrophic lateral sclerosis: NRF2 activation as a potential therapeutic strategy. Mol Neurodegen. 2021;16:71. doi: 10.1186/s13024-021-00479-8
- Pedersen WA, Cashman NR, Mattson MP. The lipid peroxidation product 4-hydroxynonenal impairs glutamate and glucose transport and choline acetyltransferase activity in NSC-19 motor neuron cells. Exp Neurol. 1999;155(1):1-10. doi: 10.1006/exnr.1998.6890
- Banerjee P, Eckert AO, Schrey AK, Preissner R. ProTox-II: A webserver for the prediction of toxicity of chemicals. Nucleic Acids Res. 2018;46(W1):W257-W263. doi: 10.1093/nar/gky318
- Tellone E, Galtieri A, Russo A, Giardina B, Ficarra S. Resveratrol: A focus on several neurodegenerative diseases. Oxid Med Cell Longev. 2015;2015:392169. doi: 10.1155/2015/392169
- Han S, Choi JR, Soon Shin K, Kang SJ. Resveratrol upregulated heat shock proteins and extended the survival of G93A-SOD1 mice. Brain Res. 2012;1483:112-117. doi: 10.1016/j.brainres.2012.09.022
- Mancuso R, del Valle J, Modol L, et al. Resveratrol improves motoneuron function and extends survival in SOD1(G93A) ALS mice. Neurotherapeutics. 2014;11(2):419-432. doi: 10.1007/s13311-013-0253-y
- Andrade S, Ramalho MJ, Pereira, MC, Loureiro JA. Resveratrol brain delivery for neurological disorders prevention and treatment. Front Pharmacol. 2018;9:1261. doi: 10.3389/fphar.2018.01261
- Nagy JA, Semple C, Lo P, Rutkove SB. Assessing the therapeutic impact of resveratrol in ALS SOD1-G93A mice with electrical impedance myography. Front Neurol. 2022;13:1059743. doi: 10.3389/fneur.2022.1059743
- Parrella E, Porrini V, Scambi I, et al. Synergistic association of resveratrol and histone deacetylase inhibitors as treatment in amyotrophic lateral sclerosis. Front Pharmacol. 2022;13:1017364. doi: 10.3389/fphar.2022.1017364
- Peterson GM, Naunton M. Valproate: A simple chemical with so much to offer. J Clin Pharm Ther. 2005;30(5):417-421. doi: 10.1111/j.1365-2710.2005.00671.x
- Crochemore C, Virgili M, Bonamassa B, et al. Long-term dietary administration of valproic acid does not affect, while retinoic acid decreases, the lifespan of G93A mice, a model for amyotrophic lateral sclerosis. Muscle Nerve. 2009;39(4):548-552. doi: 10.1002/mus.21260
- Bang WS, Kim KT, Cho DC, Kim HJ, Sung JK. Valproic Acid increases expression of neuronal stem/progenitor cell in spinal cord injury. J Korean Neurosurg Soc. 2013;54(1):8-13. doi: 10.3340/jkns.2013.54.1.8
- Zhu MM, Li HL, Shi LH, Chen XP, Luo J, Zhang ZL. The pharmacogenomics of valproic acid. J Human Genet. 2017;62(12):1009-1014. doi: 10.1038/jhg.2017.91
- Smith RG, Henry YK, Mattson MP, Appel SH. Presence of 4-hydroxynonenal in cerebrospinal fluid of patients with sporadic amyotrophic lateral sclerosis. Ann Neurol. 1998;44(4):696-699. doi: 10.1002/ana.410440419
- Pedersen WA, Fu W, Keller JN, et al. Protein modification by the lipid peroxidation product 4-hydroxynonenal in the spinal cords of amyotrophic lateral sclerosis patients. Ann Neurol. 1998;44(5):819-824. doi: 10.1002/ana.410440518
- Benedusi V, Martorana F, Brambilla L, Maggi A, Rossi D. The peroxisome proliferator-activated receptor γ (PPARγ) controls natural protective mechanisms against lipid peroxidation in amyotrophic lateral sclerosis. J Biol Chem. 2012;287(43):35899-35911. doi: 10.1074/jbc.M112.366419
- Siow RC, Ishii T, Mann GE. Modulation of antioxidant gene expression by 4-hydroxynonenal: Atheroprotective role of the Nrf2/ARE transcription pathway. Redox Rep. 2007;12(1):11-15. doi: 10.1179/135100007X162167
- Milkovic L, Zarkovic N, Marusic Z, Zarkovic K, Jaganjac M. The 4-hydroxynonenal-protein adducts and their biological relevance: Are some proteins preferred targets? Antioxidants (Basel). 2023;12:856. doi: 10.3390/antiox12040856
- Chandra A, Srivastava SK. A synthesis of 4-hydroxy-2- trans-nonenal and 4-(3H) 4-hydroxy-2-trans-nonenal. Lipids. 1997;32(7):779-782. doi: 10.1007/s11745-997-0100-6
- Valko M, Leibfritz D, Moncol J, Cronin MT, Mazur M, Telser J. Free radicals and antioxidants in normal physiological functions and human disease. Int J Biochem Cell Biol. 2007;39(1):44-84. doi: 10.1016/j.biocel.2006.07.001
- Kabuta C, Kono K, Wada K, Kabuta T. 4-Hydroxynonenal induces persistent insolubilization of TDP-43 and alters its intracellular localization. Biochem Biophys Res Commun. 2015;463(1-2):82-87. doi: 10.1016/j.bbrc.2015.05.027
- Ling N. Rotenone - A Review of Its Toxicity and Use for Fisheries Management. Science for Conservation. New Zealand: Department of Conservation; 2003. p. 1-40.
- Singer TP, Ramsay RR. The reaction sites of rotenone and ubiquinone with mitochondrial NADH dehydrogenase. Biochim Biophys Acta. 1994;1187(2):198-202. doi: 10.1016/0005-2728(94)90110-4
- DeWilde AR, Heyndrickx A, Carton D. A case of fatal rotenone poisoning in a child. J Forensic Sci. 1986;31:1492-1498.
- Wood DM, Alsahaf H, Streete P, Dargan PI, Jones AL. Fatality after deliberate ingestion of the pesticide rotenone: A case report. Crit Care. 2005;9:R280-R284. doi: 10.1186/cc3528.
- Yarmohammadi F, Hayes AW, Najafi N, Karimi G. The protective effect of natural compounds against rotenone-induced neurotoxicity. J Biochem Mol Toxicol. 2020;34:e22605. doi: 10.1002/jbt.22605
- Sai Y, Zhen J, Wu Q, Liu H, Zho J, Dong Z. Phosphorylated- ERK 1/2 and neuronal degeneration induced by rotenone in the hippocampus neuron. Environ Toxicol Pharmacol. 2008;27:366-372. doi: 10.1016/j.etap.2008.12.004
- Goldstein DS, Sullivan P, Cooney A, Jinsmaa Y, Kopin IJ, Sharabi Y. Rotenone decreases intracellular aldehyde dehydrogenase activity: Implications for the pathogenesis of Parkinson’s disease. J Neurochem. 2015;356:483-492. doi: 10.1111/jnc.13042
- Garabadu D, Agrawal N. Naringin exhibits neuroprotection against rotenone-induced neurotoxicity in experimental rodents. Neuromolecular Med. 2020;22:314-330. doi: 10.1007/s12017-019-08590-2
- Elmazoglu Z, Yar Saglam AS, Sonmez C, Karasu C. Luteolin protects microglia against rotenone-induced toxicity in a hormetic manner through targeting oxidative stress response, genes associated with Parkinson’s disease and inflammatory pathways. Drug Chem Toxicol. 2018;43:96-103. doi: 10.1080/01480545.2018.1504961
- Li X, Chen H, Guan Y, et al. Acetic acid activates the AMP-activated protein kinase signaling pathway to regulate lipid metabolism in bovine hepatocytes. PLoS One. 2013;8(7):e67880. doi: 10.1371/journal.pone.0067880
- Li L, He M, Xiao H, Liu X, Wang K, Zhang Y. Acetic acid influences BRL-3A cell lipid metabolism via the AMPK signalling pathway. Cell Physiol Biochem. 2018;45(5):2021-2030. doi: 10.1159/000487980
- Marino C, Grimaldi M, Sommella EM, et al. The metabolomic profile in amyotrophic lateral sclerosis changes according to the progression of the disease: An exploratory study. Metabolites. 2022;12(9):837. doi: 10.3390/metabo12090837
- Mähler A, Mandel S, Lorenz M, et al. Epigallocatechin- 3-gallate: A useful, effective and safe clinical approach for targeted prevention and individualised treatment of neurological diseases? EPMA J. 2013;4(1):5. doi: 10.1186/1878-5085-4-5
- Yu J, Jia Y, Guo Y, et al. Epigallocatechin-3-gallate protects motor neurons and regulates glutamate level. FEBS Lett. 2010;584(13):2921-2925. doi: 10.1016/j.febslet.2010.05.011
- Giudice ML, Cocco A, Reggiardo G, Lalli S, Albanese A. Tauro-urso-deoxycholic acid trials in amyotrophic lateral sclerosis: What is achieved and what to expect. Clin Drug Investig. 2023;43(12):893-903. doi: 10.1007/s40261-023-01324-0
- Luan H, Li X, Zhang W, Luan T. Thermal proteome profiling unveils protein targets of deoxycholic acid in living neuronal cells. Adv Biotechnol. 2023;1:7. doi: 10.1007/s44307-023-00007-3
- Fatoki TH. Human adenovirus DNA polymerase is evolutionarily and functionally associated with human telomerase reverse transcriptase based on in silico molecular characterization that implicate abacavir and zidovudine. Front Bioinform. 2023;3:123307. doi: 10.3389/fbinf.2023.1123307
- Wong F, Krishnan A, Zheng EJ, et al. Benchmarking AlphaFold-enabled molecular docking predictions for antibiotic discovery. Mol Syst Biol. 2022;18(9):e11081. doi: 10.15252/msb.202211081