NMDA receptors: Biological properties and their roles in neuropsychiatric disorders
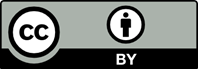
Proper signal transmission is the fundamental process of the brain activity. Changes and adaption of neuroplasticity based on the strength of synaptic transmission are essential for the information propagation in the central nervous system, which contribute to cognition, learning, and memory. Being the major excitatory neurotransmitter in the central nervous system, glutamate acts primarily through binding to the glutamate receptors, the glutamate-gated ion channels localized on post-synaptic membrane. The ionotropic glutamate receptors, pharmacologically grouped into α-amino-3-hydroxy-5-methyl-4-isoxazole propionic acid receptors, N-methyl-D-aspartic acid (NMDA) receptors, and kainate receptors, have been shown to play distinct roles in excitatory neurotransmission and synaptic plasticity. Due to their high permeability to Ca2+, the NMDA receptors have very unique function in neurotransmission and particular importance in the induction of long-term synaptic plasticity. Dysfunction of NMDA receptors causes impairment in synaptic plasticity and learning and memory. In recent years, with the development of genome-wide association studies and next-generation sequencing technology, mutations of NMDA receptor subunits have been in a variety of neuropsychiatric disorders, such as cognitive impairment, schizophrenia, autism or epilepsy. In clinical practice, NMDA receptors are known as the targets for the treatment of many neuropsychiatric disorders. In current review, we summarize current knowledge of NMDA receptors with different subunit compositions in the context of expression pattern, channel properties, protein trafficking, and synaptic plasticity as well as their roles in neuropsychiatric disorders.
Traynelis SF, Wollmuth LP, McBain CJ, et al., 2010, Glutamate receptor ion channels: Structure, regulation, and function. Pharmacol Rev, 62(3): 405–496. https://doi.org/10.1124/pr.109.002451
Alzheimer’s Association, 2022, 2022 Alzheimer’s disease facts and figures. Alzheimers Dement, 18(4): 700–789. https://doi.org/10.1002/alz.12638
Avila J, Perry GA, 2021, Multilevel view of the development of Alzheimer’s disease. Neuroscience, 457: 283–293. https://doi.org/10.1016/j.neuroscience.2020.11.015
Christensen DL, Baio J, Braun KV, et al., 2018, Prevalence and characteristics of autism spectrum disorder among children aged 8 year autism and developmental disabilities monitoring network, 11 sites, United States, 2012. Morb Mort Wkly Rep, 65: 1–23. https://doi.org/10.15585/mmwr.ss6513a1
Lord C, Brugha TS, Charman T, et al., 2020, Autism spectrum disorder. Nat Rev Dis Primers, 6(1): 5. https://doi.org/10.1038/s41572-019-0138-4
Anderson RM, Hadjichrysanthou C, Evans S, et al., 2017, Why do so many clinical trials of therapies for Alzheimer’s disease fail? Lancet, 390: 2327–2329. https://doi.org/10.1016/S0140-6736(17)32399-1
Mitsumoto H, Brooks BR, Silani V, 2014, Clinical trials in amyotrophic lateral sclerosis: Why so many negative trials and how can trials be improved? Lancet Neurol, 13: 1127–1138. https://doi.org/10.1016/S1474-4422(14)70129-2
Henriquez F, Cabello V, Baez S, et al., 2021, Multidimensional clinical assessment in frontotemporal dementia and its spectrum in latin America and the Caribbean: A narrative review and a glance at future challenges. Front Neurol, 12: 768591. https://doi.org/10.3389/fneur.2021.768591
Panza, F, Solfrizzi V, Seripa D, et al., 2015, Progresses in treating agitation: A major clinical challenge in Alzheimer’s disease. Expert Opin Pharmacother, 16: 2581–2588. https://doi.org/10.1517/14656566.2015.1092520
Song J, Patel RV, Sharif M, et al., 2022, Chemogenetics as a neuromodulatory approach to treating neuropsychiatric diseases and disorders. Mole Ther, 30: 990–1005. https://doi.org/10.1016/j.ymthe.2021.11.019
Thompson PM, Andreassen OA, Arias-Vasquez A, et al., 2017, ENIGMA and the individual: Predicting factors that affect the brain in 35 countries worldwide. NeuroImage, 145(Pt B): 389–408. https://doi.org/10.1016/j.neuroimage.2015.11.057
Schang AL, Saberan-Djoneidi D, Mezger V, 2018, The impact of epigenomic next-generation sequencing approaches on our understanding of neuropsychiatric disorders. Clin Genet, 93(3): 467–480. https://doi.org/10.1111/cge.13097
Dorado G, Galvez S, Rosales TE, et al., 2021, Analyzing modern biomolecules: The revolution of nucleic-acid sequencing review. Biomolecules, 11(8): 1111. https://doi.org/10.3390/biom11081111
Dehghan A, 2018, Genome-wide association studies. Methods Mol Biol, 1793: 37–49. https://doi.org/10.1007/978-1-4939-7868-7_4
Qin Y, Du Y, Chen L, et al., 2022, A recurrent SHANK1 mutation implicated in autism spectrum disorder causes autistic-like core behaviors in mice via downregulation of mGluR1-IP3R1-calcium signaling. Mole Psychiatry, 27(7): 2985–2998. https://doi.org/10.1038/s41380-022-01539-1
Peng SX, Pei J, Ge Y, et al., 2022, Dysfunction of AMPA receptor GluA3 is associated with aggressive behavior in human. Mol Psychiatry, Online ahead of print. https://doi.org/10.1038/s41380-022-01659-8
Li QQ, Chen J, Hu P, et al., 2022, Enhancing GluN2A-type NMDA receptors impairs long-term synaptic plasticity and learning and memory. Mol Psychiatry, Online ahead of print. https://doi.org/10.1038/s41380-022-01579-7
Teng XY, Hu P, Chen Y, et al., 2022, A novel Lgi1 mutation causes white matter abnormalities and impairs motor coordination in mice. FASEB J, 36: e22212. https://doi.org/10.1096/fj.202101652R
Hall J, Bray NJ, 2022, Schizophrenia genomics: Convergence on synaptic development, adult synaptic plasticity, or both? Biol Psychiatry, 91(8): 709–717. https://doi.org/10.1016/j.biopsych.2021.10.018
Ding YD, Chen X, Guo WB, et al., 2022, Reduced nucleus accumbens functional connectivity in reward network and default mode network in patients with recurrent major depressive disorder. Transl Psychiatry, 12: 236. https://doi.org/10.1038/s41398-022-01995-x
Paoletti P, 2011, Molecular basis of NMDA receptor functional diversity. Eur J Neurosci, 33: 1351–1365. https://doi.org/10.1111/j.1460-9568.2011.07628.x
Cull-Candy SG, Leszkiewicz DN, 2004, Role of distinct NMDA receptor subtypes at central synapses. Sci STKE, 2004(255): re16. https://doi.org/10.1126/stke.2552004re16
Rumbaugh G, Prybylowski K, Wang JF, et al., 2000, Exon 5 and spermine regulate deactivation of NMDA receptor subtypes. J Neurophysiol, 83: 1300–1306. https://doi.org/10.1152/jn.2000.83.3.1300
Vance KM, Hansen KB, Traynelis SF, 2012, GluN1 splice variant control of GluN1/GluN2D NMDA receptors. J Physiol, 590(16): 3857–3875. https://doi.org/10.1113/jphysiol.2012.234062
Horak M, Wenthold RJ, 2009, Different roles of C-terminal cassettes in the trafficking of full-length NR1 subunits to the cell surface. J Biol Chem, 284(15): 9683–9691. https://doi./org/10.1074/jbc.M807050200
Paoletti P, Bellone C, Zhou Q, 2013, NMDA receptor subunit diversity: Impact on receptor properties, synaptic plasticity and disease. Nat Rev Neurosci, 14: 383–400. https://doi.org/10.1038/nrn3504
Mayer ML, 2011, Emerging models of glutamate receptor ion channel structure and function. Structure, 19(10): 1370–1380. https://doi.org/10.1016/j.str.2011.08.009
Won S, Incontro S, Nicoll RA, et al., 2016, PSD-95 stabilizes NMDA receptors by inducing the degradation of STEP61. Proc Natl Acad Sci U S A, 113: E4736–E4744. https://doi.org/10.1073/pnas.1609702113
Incontro S, Díaz-Alonso J, Iafrati J, et al., 2019, The CaMKII/ NMDA receptor complex controls hippocampal synaptic transmission by kinase-dependent and independent mechanisms. Nat Commun, 9(1): 2069. https://doi.org/10.1038/s41467-018-04439-7
Karakas E, Furukawa H, 2014, Crystal structure of a heterotetrameric NMDA receptor ion channel. Science, 344: 992–997. https://doi.org/10.1126/science.1251915
Lee CH, Lü W, Michel JC, et al., 2014, NMDA receptor structures reveal subunit arrangement and pore architecture. Nature, 511(7508): 191–197. https://doi.org/10.1038/nature13548
Meyerson JR, Kumar J, Chittori S, et al., 2014, Structural mechanism of glutamate receptor activation and desensitization. Nature, 514(7522): 328–334. https://doi.org/10.1038/nature13603
Sobolevsky AI, 2015, Structure and gating of tetrameric glutamate receptors. J Physiol, 593(1): 29–38. https://doi.org/10.1113/jphysiol.2013.264911
Tajima N, Karakas E, Grant T, et al., 2016, Activation of NMDA receptors and the mechanism of inhibition by ifenprodil. Nature, 534(7605): 63–68. https://doi.org/10.1038/nature17679
Zhu S, Stein RA, Yoshioka C, et al., 2016, Mechanism of NMDA receptor inhibition and activation. Cell, 165(3): 704–714. https://doi.org/10.1016/j.cell.2016.03.028
Romero-Hernandez A, Simorowski N, Karakas E, et al., 2016, Molecular basis for subtype specificity and high-affinity zinc inhibition in the GluN1-GluN2A NMDA receptor amino-terminal domain. Neuron, 92(6): 1324–1336. https://doi.org/10.1016/j.neuron.2016.11.006
Furukawa H, Singh SK, Mancusso R, et al., 2005, Subunit arrangement and function in NMDA receptors. Nature, 438(7065): 185–192. https://doi.org/10.1038/nature04089
Hackos DH, Lupardus PJ, Grand T, et al., 2016, Positive allosteric modulators of GluN2A-containing NMDARs with distinct modes of action and impacts on circuit function. Neuron, 89(5): 983–999. https://doi.org/10.1016/j.neuron.2016.01.016
Volgraf M, Sellers BD, Jiang Y, et al., 2016, Discovery of GluN2A-selective NMDA receptor positive allosteric modulators (PAMs): Tuning deactivation kinetics via structure-based design. J Med Chem, 59(6): 2760–2779. https://doi.org/10.1021/acs.jmedchem.5b02010
Yi F, Mou TC, Dorsett KN, et al., 2016, Structural basis for negative allosteric modulation of GluN2A-Containing NMDA receptors. Neuron, 91(6): 1316–1329. https://doi.org/10.1016/j.neuron.2016.08.014
Jones KS, Van Dongen HM, Van Dongen AM, 2002, The NMDA receptor M3 segment is a conserved transduction element coupling ligand binding to channel opening. J Neurosci, 22(6): 2044–2053. https://doi.org/10.1523/JNEUROSCI.22-06-02044.2002
Yuan H, Erreger K, Dravid SM, et al., 2005, Conserved structural and functional control of N-methyl-D-aspartate receptor gating by transmembrane domain M3. J Biol Chem, 280(33): 29708–29716. https://doi.org/10.1074/jbc.M414215200
Chang HR, Kuo CC, 2008, The activation gate and gating mechanism of the NMDA receptor. J Neurosci, 28(7): 1546–1556. https://doi.org/10.1523/JNEUROSCI.3485-07.2008
Twomey EC, Sobolevsky AI, 2018, Structural mechanisms of gating in ionotropic glutamate receptors. Biochemistry, 57(3): 267–276. https://doi.org/10.1021/acs.biochem.7b00891
Gibb AJ, Ogden KK, McDaniel MJ, et al., 2018, A structurally derived model of subunit-dependent NMDA receptor function. J Physiol, 596(17): 4057–4089. https://doi.org/10.1113/JP276093
Watanabe M, Inoue Y, Sakimura K, et al., 1992, Developmental changes in distribution of NMDA receptor channel subunit mRNAs. Neuroreport, 3(12): 1138–1140. https://doi.org/10.1097/00001756-199212000-00027
Akazawa C, Shigemoto R, Bessho Y, et al., 1994, Differential expression of five N-methyl-D-aspartate receptor subunit mRNAs in the cerebellum of developing and adult rats. J Comp Neurol, 347(1): 150–160. https://doi.org/10.1002/cne.903470112
Monyer H, Burnashev N, Laurie DJ, et al., 1994, Developmental and regional expression in the rat brain and functional properties of four NMDA receptors. Neuron, 12(3): 529–540. https://doi.org/10.1016/0896-6273(94)90210-0
Laurie DJ, Seeburg PH, 1994, Regional and developmental heterogeneity in splicing of the rat brain NMDAR1 mRNA. J Neurosci, 14(5): 3180–3194. https://doi.org/10.1523/JNEUROSCI.14-05-03180.1994
Ishii T, Moriyoshi K, Sugihara H, et al., 1993, Molecular characterization of the family of the N-methyl-D-aspartate receptor subunits. J Biol Chem, 268(4): 2836–2843.
Sheng M, Cummings J, Roldan LA, et al., 1994, Changing subunit composition of heteromeric NMDA receptors during development of rat cortex. Nature, 368(6467): 144–147. https://doi.org/10.1038/368144a0
Henson MA, Roberts AC, Perez-Otano I, et al., 2010, Influence of the NR3A subunit on NMDA receptor functions. Prog Neurobiol, 91: 23–37. https://doi.org/10.1016/j.pneurobio.2010.01.004
Pachernegg S, Strutz-Seebohm N, Hollmann M, 2012, GluN3 subunit-containing NMDA receptors: Not just one-trick ponies. Trends Neurosci, 35(4): 240–249. https://doi.org/10.1016/j.tins.2011.11.010
Johnson JW, Ascher P, 1987, Glycine potentiates the NMDA response in cultured mouse brain neurons. Nature, 325(6104): 529–531. https://doi.org/10.1038/325529a0
Kleckner NW, Dingledine R, 1988, Requirement for glycine in activation of NMDA-receptors expressed in Xenopus oocytes. Science, 241(4867): 835–837. https://doi.org/10.1126/science.284175
Watkins JC, Evans RH, 1981, Excitatory amino acid transmitters. Annu Rev Pharmacol Toxicol, 21: 165–204. https://doi.org/10.1146/annurev.pa.21.040181.001121
Patneau DK, Mayer ML, 1990, Structure-activity relationships for amino acid transmitter candidates acting at N-methyl-D-aspartate and quisqualate receptors. J Neurosci, 10(7): 2385–2399. https://doi.org/10.1523/JNEUROSCI.10-07-02385.199
Clements JD, Westbrook GL, 1991, Activation kinetics reveal the number of glutamate and glycine binding sites on the N-methyl-D-aspartate receptor. Neuron, 7(4): 605–613. https://doi.org/10.1016/0896-6273(91)90373-8
Chatterton JE, Awobuluyi M, Premkumar LS, et al., 2002, Excitatory glycine receptors containing the NR3 family of NMDA receptor subunits. Nature, 415(6873): 793–798. https://doi.org/10.1038/nature715
Iijima K, Takase S, Tsumuraya K, et al., 1978, Changes in free amino acids of cerebrospinal fluid and plasma in various neurological diseases. Tohoku J Exp Med, 126(2): 133–150. https://doi.org/10.1620/tjem.126.133
Papouin T, Ladépêche L, Ruel J, et al., 2012, Synaptic and extrasynaptic NMDA receptors are gated by different endogenous coagonists. Cell, 150(3): 633–646. https://doi.org/10.1016/j.cell.2012.06.029
Erreger K, Geballe MT, Kristensen A, et al., 2007, Subunit-specific agonist activity at NR2A, NR2B-, NR2C, and NR2D-containing N-methyl-D-aspartate glutamate receptors. Mol Pharmacol, 72(4): 907–920. https://doi.org/10.1124/mol.107.037333
Madry C, Betz H, Geiger JR, et al., 2010, Potentiation of glycine-gated NR1/NR3A NMDA receptors relieves ca-dependent outward rectification. Front Mol Neurosci, 3: 6. https://doi.org/10.3389/fnmol.2010.00006
Ulbrich MH, Isacoff EY, 2007, Subunit counting in membrane-bound proteins. Nat Methods, 4(4): 319–321. https://doi.org/10.1038/nmeth1024
Ulbrich MH, Isacoff EY, 2008, Rules of engagement for NMDA receptor subunits. Proc Natl Acad Sci U S A, 105(37): 14163–14168.https://doi.org/10.1073/pnas.0802075105
Awobuluyi M, Yang J, Ye Y, et al., 2007, Subunit-specific roles of glycine-binding domains in activation of NR1/ NR3 N-methyl-D-aspartate receptors. Mol Pharmacol, 71(1): 112–122. https://doi.org/10.1124/mol.106.030700
Madry C, Mesic I, Nicke A, et al., 2007, Principal role of NR3 subunits in NR1/NR3 excitatory glycine receptor function. Biochem Biophys Res Commun, 354(1): 102–108. https://doi.org/10.1016/j.bbrc.2006.12.153
Retchless S, Gao B, Johnson J W, 2012, A single GluN2 subunit residue controls NMDA receptor channel properties via intersubunit interaction. Nat Neurosci, 15: 406–413, S401–S402. https://doi.org/10.1038/nn.3025
Chen N, Luo T, Raymond LA, 1999, Subtype-dependence of NMDA receptor channel open probability. J Neurosci, 19(16): 6844–6854. https://doi.org/10.1523/JNEUROSCI.19-16-06844.1999
Errege K, Dravid SM, Banke TG, et al., 2005, Subunit-specific gating controls rat NR1/NR2A and NR1/NR2B NMDA channel kinetics and synaptic signalling profiles. J Physiol, 563(Pt 2): 345–358. https://doi.org/10.1113/jphysiol.2004.080028
Sobczyk A, Scheuss V, Svoboda K, 2005, NMDA receptor subunit-dependent [Ca2+] signaling in individual hippocampal dendritic spines. J Neurosci, 25(26): 6037–6046. https://doi.org/10.1523/JNEUROSCI.1221-05.2005
Sprengel R, Suchanek B, Amico C, et al., 1998, Importance of the intracellular domain of NR2 subunits for NMDA receptor function in vivo. Cell, 92(2): 279–289. https://doi.org/10.1016/s0092-8674(00)80921-6
Martel MA, Ryan TJ, Bell KF, et al., 2012, The subtype of GluN2 C-terminal domain determines the response to excitotoxic insults. Neuron, 74(3): 543–556. https://doi.org/10.1016/j.neuron.2012.03.021
Sanz-Clemente A, Nicoll RA, Roche KW, 2013, Diversity in NMDA receptor composition: Many regulators, many consequences. Neuroscientist, 19(1): 62–75. https://doi.org/10.1177/1073858411435129
Mu Y, Otsuka T, Horton AC, et al., 2003, Activity-dependent mRNA splicing controls ER export and synaptic delivery of NMDA receptors. Neuron, 40(3): 581–594. https://doi.org/10.1016/s0896-6273(03)00676-7
Perez-Otano I, Luján R, Tavalin SJ, et al., 2006, Endocytosis and synaptic removal of NR3A-containing NMDA receptors by PACSIN1/syndapin1. Nat Neurosci, 9(5): 611–621. https://doi.org/10.1038/nn1680
Horak M, Petralia RS, Kaniakova M, et al., 2014, ER to synapse trafficking of NMDA receptors. Front Cell Neurosci, 8: 394. https://doi./org/10.3389/fncel.2014.00394
McIlhinney RA, Le Bourdellès B, Molnár E, et al., 1998, Assembly intracellular targeting and cell surface expression of the human N-methyl-D-aspartate receptor subunits NR1a and NR2A in transfected cells. Neuropharmacology, 37(10–11): 1355–1367. https://doi.org/10.1016/s0028-3908(98)00121-x
Fukaya M, Kato A, Lovett C, et al., 2003, Retention of NMDA receptor NR2 subunits in the lumen of endoplasmic reticulum in targeted NR1 knockout mice. Proc Natl Acad Sci U S A, 100(8): 4855–4860. https://doi.org/10.1073/pnas.0830996100
Horak M, Chang K, Wenthold RJ, 2008, Masking of the endoplasmic reticulum retention signals during assembly of the NMDA receptor. J Neurosci, 28(13): 3500–3509. https://doi.org/10.1523/JNEUROSCI.5239-07.2008
Standley S, Roche KW, McCallum J, et al., 2000, PDZ domain suppression of an ER retention signal in NMDA receptor NR1 splice variants. Neuron, 28(3): 887–898. https://doi.org/10.1016/s0896-6273(00)00161-6
Scott DB, Blanpied TA, Swanson GT, et al, 2001, An NMDA receptor ER retention signal regulated by phosphorylation and alternative splicing. J Neurosci, 21(3): 3063–3072. https://doi.org/10.1523/JNEUROSCI.21-09-03063.2001
Prybylowski K, Fu Z, Losi G, et al., 2002, Relationship between availability of NMDA receptor subunits and their expression at the synapse. J Neurosci, 22(20): 8902–8910. https://doi.org/10.1523/JNEUROSCI.22-20-08902.2002
Hawkins LM, Prybylowski K, Chang K, et al., 2004, Export from the endoplasmic reticulum of assembled N-methyl-d-aspartic acid receptors is controlled by a motif in the c terminus of the NR2 subunit. J Biol Chem, 279(28): 28903–28910. https://doi.org/10.1074/jbc.M402599200
Yang W, Zheng C, Song Q, et al., 2007, A three amino acid tail following the TM4 region of the N-methyl-D-aspartate receptor (NR) 2 subunits is sufficient to overcome endoplasmic reticulum retention of NR1-1a subunit. J Biol Chem, 282(1): 9269–9278. https://doi.org/10.1074/jbc.M700050200
Sans N, Prybylowski K, Petralia RS, et al., 2003, NMDA receptor trafficking through an interaction between PDZ proteins and the exocyst complex. Nat Cell Biol, 5(6): 520–530. https://doi.org/10.1038/ncb990
Sans N, Wang PY, Du Q, et al., 2005, mPins modulates PSD-95 and SAP102 trafficking and influences NMDA receptor surface expression. Nat Cell Biol, 7(12): 1179–1190. https://doi.org/10.1038/ncb1325
Sans N, Petralia RS, Wang YX, et al., 2000, A developmental change in NMDA receptor-associated proteins at hippocampal synapses. J Neurosci, 20(3): 1260–1271. https://doi.org/10.1523/JNEUROSCI.20-03-01260.2000
Muller BM, Kistner U, Kindler S, et al., 1996, SAP102, a novel postsynaptic protein that interacts with NMDA receptor complexes in vivo. Neuron, 17(2): 255–265. https://doi.org/10.1016/s0896-6273(00)80157-9
Jeyifous O, Waites CL, Specht CG, et al., 2009, SAP97 and CASK mediate sorting of NMDA receptors through a previously unknown secretory pathway. Nat Neurosci, 12(8): 1011-1019. https://doi.org/10.1038/nn.2362
Setou M, Nakagawa T, Seog DH, et al., 2000, Kinesin superfamily motor protein KIF17 and mLin-10 in NMDA receptor-containing vesicle transport. Science, 288: 1796–1802. https://doi.org/10.1126/science.288.5472.1796
Guillaud L, Setou M, Hirokawa N, 2003, KIF17 dynamics and regulation of NR2B trafficking in hippocampal neurons. J Neurosci, 23(1): 131–140. https://doi.org/10.1523/JNEUROSCI.23-01-00131.2003
Yin X, Takei Y, Kido MA, et al., 2011, Molecular motor KIF17 is fundamental for memory and learning via differential support of synaptic NR2A/2B levels. Neuron, 70(2): 310–325. https://doi.org/10.1016/j.neuron.2011.02.049
Lin EI, Jeyifous O, Green WN, 2013, CASK regulates SAP97 conformation and its interactions with AMPA and NMDA receptors. J Neurosci, 33(29): 12067–12076. https://doi.org/10.1523/JNEUROSCI.0816-13.2013
Gardoni F, Mauceri D, Fiorentini C, et al., 2003, CaMKII-dependent phosphorylation regulates SAP97/NR2A interaction. J Biol Chem, 278(45): 44745–44752. https://doi.org/10.1074/jbc.M303576200
Mauceri D, Gardoni F, Marcello E, et al., 2007, Dual role of CaMKII-dependent SAP97 phosphorylation in mediating trafficking and insertion of NMDA receptor subunit NR2A. J Neurochem, 100(4): 1032–1046. https://doi.org/10.1111/j.1471-4159.2006.04267.x
Yin X, Feng X, Takei Y, et al., 2012, Regulation of NMDA receptor transport: A KIF17-cargo binding/releasing underlies synaptic plasticity and memory in vivo. J Neurosci, 32: 5486–5499. https://doi.org/10.1523/JNEUROSCI.0718-12.2012
Thomas GM, Huganir RL, 2013, Palmitoylation-dependent regulation of glutamate receptors and their PDZ domain-containing partners. Biochem Soc Trans, 41: 72–78. https://doi.org/10.1042/BST20120223
Lussier MP, Sanz-Clemente A, Roche KW, 2015, Dynamic regulation of N-Methyl-d-aspartate (NMDA) and alpha-amino-3-hydroxy-5-methyl-4-isoxazolepropionic acid (AMPA) receptors by posttranslational modifications. J Biol Chem, 290: 28596–28603. https://doi.org/10.1074/jbc.R115.652750
Hayashi Y, Shi SH, Esteban JA, et al., 2000, Driving AMPA receptors into synapses by LTP and CaMKII: Requirement for GluR1 and PDZ domain interaction. Science, 287: 2262–2267. https://doi.org/10.1126/science.287.5461.2262
Mattison HA, Hayashi T, Barria A, 2012, Palmitoylation at two cysteine clusters on the C-terminus of GluN2A and GluN2B differentially control synaptic targeting of NMDA receptors. PLoS One, 7: e49089. https://doi.org/10.1371/journal.pone.0049089
Gu Y, Huganir RL, 2016, Identification of the SNARE complex mediating the exocytosis of NMDA receptors. Proc Natl Acadf Sci U S A, 113: 12280–12285. https://doi.org/10.1073/pnas.1614042113
Ladepeche L, Dupuis JP, Groc L, 2014, Surface trafficking of NMDA receptors: Gathering from a partner to another. Seminars Cell Dev Biol, 27: 3–13. https://doi.org/10.1016/j.semcdb.2013.10.005
Sudhof TC, Rothman JE, 2009, Membrane fusion: grappling with SNARE and SM proteins. Science, 323: 474–477. https://doi.org/10.1126/science.1161748
Lan JY, Skeberdis VA, Jover T, et al., 2001, Protein kinase C modulates NMDA receptor trafficking and gating. Nat Neurosci, 4(4): 382–390. https://doi.org/10.1038/86028
Lan JY, Skeberdis VA, Jover T, et al., 2001, Activation of metabotropic glutamate receptor 1 accelerates NMDA receptor trafficking. J Neurosci, 21(16): 6058–6068.
Lau CG, Takayasu Y, Rodenas-Ruano A, et al., 2010, SNAP- 25 is a target of protein kinase C phosphorylation critical to NMDA receptor trafficking. J Neurosci, 30(1): 242–254. https://doi.org/10.1523/JNEUROSCI.4933-08.2010
Jurado S, Goswami D, Zhang Y, et al., 2013, LTP requires a unique postsynaptic SNARE fusion machinery. Neuron, 77(3): 542–558. https://doi.org/10.1016/j.neuron.2012.11.029
Washbourne P, Liu XB, Jones EG, et al., 2004, Cycling of NMDA receptors during trafficking in neurons before synapse formation. J Neurosci, 24(38): 8253–8264. https://doi.org/10.1523/JNEUROSCI.2555-04.2004
Suh YH, Terashima A, Petralia RS, et al., 2010, A neuronal role for SNAP-23 in postsynaptic glutamate receptor trafficking. Nat Neurosci, 13(3): 338–343. https://doi.org/10.1038/nn.2488
Tovar KR, Westbrook GL, 2002, Mobile NMDA receptors at hippocampal synapses. Neuron, 34(2): 255–264. https://doi.org/10.1016/s0896-6273(02)00658-x
Groc L, Heine M, Cousins SL, et al., 2006, NMDA receptor surface mobility depends on NR2A-2B subunits. Proc Natl Acad Sci U S A, 103(49): 18769–18774. https://doi.org/10.1073/pnas.0605238103
Dupuis JP, Ladepeche L, Seth H, et al., 2014, Surface dynamics of GluN2B-NMDA receptors controls plasticity of maturing glutamate synapses. EMBO J, 33(8): 842–861. https://doi.org/10.1002/embj.201386356
Ferreira JS, Papouin T, Ladépêche L, et al., 2017, Co-agonists differentially tune GluN2B-NMDA receptor trafficking at hippocampal synapses. ELife 6: e25492. https://doi.org/10.7554/eLife.25492
Sun YJ, Xu YG, Chen XK, et al., 2018, The differences between GluN2A and GluN2B signaling in the brain. J Neuro Res, 96: 1430–1443. https://doi.org/10.1002/jnr.24251
Kellermayer B, Ferreira JS, Dupuis J, et al., 2018, Differential nanoscale topography and functional role of GluN2-NMDA receptor subtypes at glutamatergic synapses. Neuron, 100(1): 106–119. e107. https://doi.org/10.1016/j.neuron.2018.09.012
Bard L, Sainlos M, Bouchet D, et al., 2010, Dynamic and specific interaction between synaptic NR2-NMDA receptor and PDZ proteins. Proc Natl Acad Sci U S A, 107(45): 19561–19566. https://doi.org/10.1073/pnas.1002690107
Chung HJ, Huang YH, Lau LF, et al., 2004, Regulation of the NMDA receptor complex and trafficking by activity-dependent phosphorylation of the NR2B subunit PDZ ligand. J Neurosci, 24: 10248–10259. https://doi.org/10.1523/JNEUROSCI.0546-04.2004
Chiu AM, Wang J, Fiske MP, et al., 2019, NMDAR-Activated PP1 Dephosphorylates GluN2B to Modulate NMDAR Synaptic Content. Cell Rep, 28(2): 332–341 e335. https://doi.org/10.1016/j.celrep.2019.06.030
Lesept F, Chevilley A, Jezequel J, et al., 2016, Tissue-type plasminogen activator controls neuronal death by raising surface dynamics of extrasynaptic NMDA receptors. Cell Death Dis, 7(11): e2466. https://doi.org/10.1038/cddis.2016.279
Michaluk P, Groc L, Mikasova L, et al., 2009, Matrix metalloproteinase-9 controls NMDA receptor surface diffusion through integrin beta1 signaling. J Neurosci, 29(18): 6007–6012. https://doi.org/10.1523/JNEUROSCI.5346-08.2009
Mikasova L, Xiong H, Kerkhofs A, et al., 2017, Stress hormone rapidly tunes synaptic NMDA receptor through membrane dynamics and mineralocorticoid signalling. Sci Rep, 7: 8053. https://doi.org/10.1038/s41598-017-08695-3
Potier M, Georges F, Brayda-Bruno L, et al., 2016, Temporal memory and its enhancement by estradiol requires surface dynamics of hippocampal CA1 N-methyl-D-aspartate receptors. Biol Psychiatry, 79: 735–745. https://doi.org/1016/j.biopsych.2015.07.017
Roche KW, Standley S, McCallum J, et al., 2001, Molecular determinants of NMDA receptor internalization. Nature Neurosci, 4: 794–802. https://doi.org/1038/90498
Lavezzari G, McCallum J, Dewey CM, et al., 2004, Subunit-specific regulation of NMDA receptor endocytosis. J Neurosci, 24: 6383–6391. https://doi.org/1523/JNEUROSCI.1890-04.2004
Blanpied TA, Scott DB, Ehlers MD, 2002, Dynamics and regulation of clathrin coats at specialized endocytic zones of dendrites and spines. Neuron, 36: 435–449. https://doi.org/1016/s0896-6273(02)00979-0
Prybylowski K, Chang K, Sans N, et al., 2005, The synaptic localization of NR2B-containing NMDA receptors is controlled by interactions with PDZ proteins and AP-2. Neuron, 47: 845–857. https://doi.org/1016/j.neuron.2005.08.016
Lavezzari G, McCallum J, Lee R, et al., 2003, Differential binding of the AP-2 adaptor complex and PSD-95 to the C-terminus of the NMDA receptor subunit NR2B regulates surface expression. Neuropharmacology, 45: 729–737. https://doi.org/1016/s0028-3908(03)00308-3
Scott DB, Michailidis I, Mu Y, et al., 2004, Endocytosis and degradative sorting of NMDA receptors by conserved membrane-proximal signals. J Neurosci, 24: 7096–7109. https://doi.org/1523/JNEUROSCI.0780-04.2004
Bliss TV, Lomo T, 1973, Long-lasting potentiation of synaptic transmission in the dentate area of the anaesthetized rabbit following stimulation of the perforant path. J Physiol, 232: 331–356. https://doi.org/1113/jphysiol.1973.sp010273
Collingridge GL, Kehl SJ, McLennan H, 1983, The antagonism of amino acid-induced excitations of rat hippocampal CA1 neurones in vitro. J Physiol, 334: 19–31. https://doi.org/1113/jphysiol.1983.sp014477
Morris RG, 1989, Synaptic plasticity and learning: Selective impairment of learning rats and blockade of long-term potentiation in vivo by the N-methyl-D-aspartate receptor antagonist AP5. J Neurosci, 9: 3040–3057.
Morris RG, Anderson E, Lynch GS, et al., 1986, Selective impairment of learning and blockade of long-term potentiation by an N-methyl-D-aspartate receptor antagonist, AP5. Nature, 319: 774–776. https://doi.org/1038/319774a0
Norris CM, Foster TC, 1999, MK-801 improves retention in aged rats: Implications for altered neural plasticity in age-related memory deficits. Neurobiol Learn Mem, 71: 194–206. https://doi.org/1006/nlme.1998.3864
Villarreal DM, Do V, Haddad E, et al., NMDA receptor antagonists sustain LTP and spatial memory: Active processes mediate LTP decay. Nat Neurosci, 5: 48–52. https://doi.org/1038/nn776
Josselyn SA, Nguyen PV, 2005, CREB, synapses and memory disorders: past progress and future challenges. Curr Drug Targets CNS Neurol Disord, 4: 481–497. https://doi.org/2174/156800705774322058
Malinow R, Malenka RC, 2002, AMPA receptor trafficking and synaptic plasticity. Annu Rev Neurosci, 25: 103–126. https://doi.org/1146/annurev.neuro.25.112701.142758
Citri A, Malenka RC, 2008, Synaptic plasticity: multiple forms, functions, and mechanisms. Neuropsychopharmacology, 33: 18–41. https://doi.org/1038/sj.npp.1301559
Malenka RC, 2003, Synaptic plasticity and AMPA receptor trafficking. Ann N Y Acad Sci, 1003: 1–11. https://doi.org/1196/annals.1300.001
Malenka RC, Bear MF, 2004, LTP and LTD: an embarrassment of riches. Neuron, 44: 5–21. https://doi.org/1016/j.neuron.2004.09.012
Strack S, Colbran RJ, 1998, Autophosphorylation-dependent targeting of calcium/calmodulin-dependent protein kinase II by the NR2B subunit of the N-methyl- D-aspartate receptor. J Biol Chem, 273: 20689–20692. https://doi.org/1074/jbc.273.33.20689
Barria A, Malinow R, 2005, NMDA receptor subunit composition controls synaptic plasticity by regulating binding to CaMKII. Neuron, 48: 289–301. https://doi.org/1016/j.neuron.2005.08.034
Lu HC, Gonzalez E, Crair MC, 2001, Barrel cortex critical period plasticity is independent of changes in NMDA receptor subunit composition. Neuron, 32: 619–634. https://doi.org/1016/s0896-6273(01)00501-3
Zhao MG, Toyoda H, Lee YS, et al., 2005, Roles of NMDA NR2B subtype receptor in prefrontal long-term potentiation and contextual fear memory. Neuron, 47: 859-872. https://doi.org/1016/j.neuron.2005.08.014
Akashi K, Kakizaki T, Kamiya H, et al., 2009, NMDA receptor GluN2B (GluR epsilon 2/NR2B) subunit is crucial for channel function, postsynaptic macromolecular organization, and actin cytoskeleton at hippocampal CA3 synapses. J Neurosci, 29: 10869–10882. https://doi.org/1523/JNEUROSCI.5531-08.2009
Brigman JL, Wright T, Talani G, et al., 2010, Loss of GluN2B-containing NMDA receptors in CA1 hippocampus and cortex impairs long-term depression, reduces dendritic spine density, and disrupts learning. J Neurosci, 30: 4590–4600. https://doi.org/1523/JNEUROSCI.0640-10.2010
Von Engelhardt, J., Doganci B, Jensen V, et al., 2008, Contribution of hippocampal and extra-hippocampal NR2B-containing NMDA receptors to performance on spatial learning tasks. Neuron, 60: 846–860. https://doi.org/1016/j.neuron.2008.09.039(2008)
Tang YP, Shimizu E, Dube GR, et al., 1999, Genetic enhancement of learning and memory in mice. Nature, 401: 63–69. https://doi.org/1038/43432
Zhou Y, Takahashi E, Li W, et al., 2007, Interactions between the NR2B receptor and CaMKII modulate synaptic plasticity and spatial learning. J Neurosci, 27: 13843–13853. https://doi.org/1523/JNEUROSCI.4486-07.2007
Berberich S, Punnakkal P, Jensen V, et al., Lack of NMDA receptor subtype selectivity for hippocampal long-term potentiation. J Neurosci, 25: 6907–6910. https://doi.org/1523/JNEUROSCI.1905-05.2005
Weitlauf C, Honse Y, Auberson YP, et al., 2005, Activation of NR2A-containing NMDA receptors is not obligatory for NMDA receptor-dependent long-term potentiation. J Neurosci, 25: 8386–8390. https://doi.org/1523/JNEUROSCI.2388-05.2005
Cui Z, Feng R, Jacobs S, et al., Increased NR2A: NR2B ratio compresses long-term depression range and constrains long-term memory. Sci Rep, 3: 1036. https://doi.org/1038/srep01036
Hawasli AH, Benavides DR, Nguyen C, et al., 2007, Cyclin-dependent kinase 5 governs learning and synaptic plasticity via control of NMDAR degradation. Nat Neurosci, 10: 880–886. https://doi.org/1038/nn1914
Liu L, Wong TP, Pozza MF, et al., 2004, Role of NMDA receptor subtypes in governing the direction of hippocampal synaptic plasticity. Science, 304: 1021–1024. https://doi.org/1126/science.1096615
Li R, Huang FS, Abbas AK, et al., 2007, Role of NMDA receptor subtypes in different forms of NMDA-dependent synaptic plasticity. BMC Neurosci, 8: 55. https://doi.org/1186/1471-2202-8-55
Sakimura K, Kutsuwada T, Ito I, et al., 1995, Reduced hippocampal LTP and spatial learning in mice lacking NMDA receptor epsilon 1 subunit. Nature, 373: 151–155. https://doi.org/1038/373151a0
Foster KA, McLaughlin N, Edbauer D, et al., 2010, Distinct roles of NR2A and NR2B cytoplasmic tails in long-term potentiation. J Neurosci, 30: 2676–2685. https://doi.org/1523/JNEUROSCI.4022-09.2010
Delaney AJ, Sedlak PL, Autuori E, et al., 2013, Synaptic NMDA receptors in basolateral amygdala principal neurons are triheteromeric proteins: Physiological role of GluN2B subunits. J Neurophysiol, 109: 1391–1402. https://doi.org/1152/jn.00176.2012
Morishita W, Lu W, Smith GB, et al., 2007, Activation of NR2B-containing NMDA receptors is not required for NMDA receptor-dependent long-term depression. Neuropharmacology, 52: 71–76. https://doi.org/1016/j.neuropharm.2006.07.005
Hendricson AW, Miao CL, Lippmann MJ, et al., 2002, Ifenprodil and ethanol enhance NMDA receptor-dependent long-term depression. J Pharmacol Exp Ther, 301: 938-944. https://doi.org/1124/jpet.301.3.938
Gardoni F, Mauceri D, Malinverno M, et al., 2009, Decreased NR2B subunit synaptic levels cause impaired long-term potentiation but not long-term depression. J Neurosci, 29: 669–677. https://doi.org/1523/JNEUROSCI.3921-08.2009
Wang D, Cui Z, Zeng Q, et al., 2009, Genetic enhancement of memory and long-term potentiation but not CA1 long-term depression in NR2B transgenic rats. PLoS One, 4: e7486. https://doi.org/1371/journal.pone.0007486
Bartlett TE, Bannister NJ, Collett VJ, et al., 2007, Differential roles of NR2A and NR2B-containing NMDA receptors in LTP and LTD in the CA1 region of two-week old rat hippocampus. Neuropharmacology, 52: 60–70. https://doi.org/1016/j.neuropharm.2006.07.013
Ge Y, Dong Z, Bagot RC, et al., 2010, Hippocampal long-term depression is required for the consolidation of spatial memory. Proc Natl Acad Sci U S A, 107: 16697–16702. https://doi.org/1073/pnas.1008200107
Longordo F, Kopp C, Mishina M, et al., 2009, NR2A at CA1 synapses is obligatory for the susceptibility of hippocampal plasticity to sleep loss. J Neurosci., 29: 9026–9041. https://doi.org/1523/JNEUROSCI.1215-09.2009
Kalia LV, Kalia SK, Salter MW, 2008, NMDA receptors in clinical neurology: excitatory times ahead. Lancet Neurol, 7: 742–755. https://doi.org/1016/S1474-4422(08)70165-0
Berman RM, Cappiello A, Anand A, et al., 2000, Antidepressant effects of ketamine in depressed patients. Biol Psychiatry, 47: 351–354. https://doi.org/1016/s0006-3223(99)00230-9
Murrough JW, Perez AM, Pillemer S, et al., 2013, Rapid and longer-term antidepressant effects of repeated ketamine infusions in treatment-resistant major depression. Biol Psychiatry, 74: 250–256. https://doi.org/1016/j.biopsych.2012.06.022
Preskorn S, Macaluso M, Mehra DO, et al., 2015, Randomized proof of concept trial of GLYX-13, an N-methyl-D-aspartate receptor glycine site partial agonist, in major depressive disorder nonresponsive to a previous antidepressant agent. J Psychiatric Pract, 21: 140–149. https://doi.org/1097/01.pra.0000462606.17725.93
Jiang J, Jiang H, 2015, Efficacy and adverse effects of memantine treatment for Alzheimer’s disease from randomized controlled trials. Neurol Sci, 36: 1633–1641. https://doi.org/1007/s10072-015-2221-2
Knapp M, King D, Romeo R, et al., 2017, Cost-effectiveness of donepezil and memantine in moderate to severe Alzheimer’s disease (the DOMINO-AD trial). Int J Geriatr Psychiatry, 32: 1205–1216. https://doi.org/1002/gps.4583
O’Collins VE, Macleod MR, Donnan GA, et al., 2006, 1,026 experimental treatments in acute stroke. Ann Neurol, 59: 467–477. https://doi.org/1002/ana.20741
Feng B, Morley RM, Jane DE, et al., 2005, The effect of competitive antagonist chain length on NMDA receptor subunit selectivity. Neuropharmacology, 48: 354–359. https://doi.org/1016/j.neuropharm.2004.11.004
Auberson YP, Allgeier H, Bischoff S, et al., 2002, 5-Phosphonomethylquinoxalinediones as competitive NMDA receptor antagonists with a preference for the human 1A/2A, rather than 1A/2B receptor composition. Bioorg Med Chem Lett, 12: 1099–1102. https://doi.org/1016/s0960-894x(02)00074-4
Hood WF, Sun ET, Compton RP, et al., 1989, 1-Aminocyclobutane-1-carboxylate (ACBC): A specific antagonist of the N-methyl-D-aspartate receptor coupled glycine receptor. Eur J Pharmacol, 161: 281–282. https://doi.org/1016/0014-2999(89)90861-3
Kemp JA, Foster AC, Leeson PD, et al., 1988, 7-Chlorokynurenic acid is a selective antagonist at the glycine modulatory site of the N-methyl-D-aspartate receptor complex. Proc Natl Acad Sci U S A, 85: 6547–6550. https://doi.org/1073/pnas.85.17.6547
Davies J, Watkins JC, 1972, Is 1-hydroxy-3- aminopyrrolidone-2 (HA-966) a selective excitatory amino-acid antagonist? Nat New Biol, 238: 61–63. https://doi.org/1038/newbio238061a0
Priestley T, Laughton P, Macaulay AJ, et al., 1996, Electrophysiological characterisation of the antagonist properties of two novel NMDA receptor glycine site antagonists, L-695,902 and L-701,324. Neuropharmacology, 35: 1573–1581. https://doi.org/1016/s0028-3908(96)00141-4
Anis N, Sherby S, Goodnow R Jr., et al., 1990, Structure-activity relationships of philanthotoxin analogs and polyamines on N-methyl-D-aspartate and nicotinic acetylcholine receptors. J Pharmacol Exp Ther, 254: 764–773.
Lodge D, Johnson KM, 1990, Noncompetitive excitatory amino acid receptor antagonists. Trends Pharmacol Sci, 11: 81–86. https://doi.org/1016/0165-6147(90)90323-z
Wong EH, Kemp JA, Priestley T, et al., 1986, The anticonvulsant MK-801 is a potent N-methyl-D-aspartate antagonist. Proc Natl Acad Sci U S A, 83: 7104–7108. https://doi.org/1073/pnas.83.18.7104
Carter C, Rivy JP, Scatton B, 1989, Ifenprodil and SL 82.0715 are antagonists at the polyamine site of the N-methyl-D-aspartate (NMDA) receptor. Eur J Pharmacol, 164: 611–612. https://doi.org/1016/0014-2999(89)90275-6
Karakas E, Simorowski N, Furukawa H, 2011, Subunit arrangement and phenylethanolamine binding in GluN1/ GluN2B NMDA receptors. Nature, 475: 249–253. https://doi.org/1038/nature10180
Chenard BL, Bordner J, Butler TW, et al., 1995, (1S,2S)-1- (4-hydroxyphenyl)-2-(4-hydroxy-4-phenylpiperidino)- 1-propanol: A potent new neuroprotectant which blocks N-methyl-D-aspartate responses. J Med Chem, 38: 3138–3145. https://doi.org/1021/jm00016a017
Fischer G, et al., 1997, Ro 25-6981, a highly potent and selective blocker of N-methyl-D-aspartate receptors containing the NR2B subunit. Characterization in vitro. J Pharmacol Exp Ther, 283: 1285–1292.
Bettini E, Sava A, Griffante C, et al., 2010, Identification and characterization of novel NMDA receptor antagonists selective for NR2A- over NR2B-containing receptors. J Pharmacol Exp Ther, 335: 636–644. https://doi.org/1124/jpet.110.172544
Hansen KB, Ogden KK, Traynelis SF, 2012, Subunit-selective allosteric inhibition of glycine binding to NMDA receptors. J Neurosci, 32: 6197–6208. https://doi.org/1523/JNEUROSCI.5757-11.2012
Edman S, McKay S, MacDonald LJ, et al., 2012, TCN 201 selectively blocks GluN2A-containing NMDARs in a GluN1 co-agonist dependent but non-competitive manner. Neuropharmacology, 63: 441–449. https://doi.org/1016/j.neuropharm.2012.04.
Mosley CA, Acker TM, Hansen KB, et al., 2010, Quinazolin- 4-one derivatives: A novel class of noncompetitive NR2C/D subunit-selective N-methyl-D-aspartate receptor antagonists. J Med Chem, 53: 5476–5490. https://doi.org/1021/jm100027p
Hansen KB, Traynelis SF, 2011, Structural and mechanistic determinants of a novel site for noncompetitive inhibition of GluN2D-containing NMDA receptors. J Neurosci, 31: 3650–3661. https://doi.org/1523/JNEUROSCI.5565-10.2011
Costa BM, Irvine MW, Fang G, et al., 2010, A novel family of negative and positive allosteric modulators of NMDA receptors. J Pharmacol Exp Ther, 335: 614–621. https://doi.org/1124/jpet.110.174144
Costa BM, Irvine MW, Fang G, et al., 2012, Structure-activity relationships for allosteric NMDA receptor inhibitors based on 2-naphthoic acid. Neuropharmacology, 62: 1730–1736. https://doi.org/1016/j.neuropharm.2011.11.019
Kloda A, Clements JD, Lewis RJ, et al., 2004, Adenosine triphosphate acts as both a competitive antagonist and a positive allosteric modulator at recombinant N-methyl-D-aspartate receptors. Mol Pharmacol, 65: 1386–1396. https://doi.org/1124/mol.65.6.1386
Williams K, 1994, Subunit-specific potentiation of recombinant N-methyl-D-aspartate receptors by histamine. Mol Pharmacol, 46: 531–541.
Williams K, Zappia AM, Pritchett DB, et al., 1994, Sensitivity of the N-methyl-D-aspartate receptor to polyamines is controlled by NR2 subunits. Mol Pharmacol, 45: 803–809.
Sedlacek M, Korínek M, Petrovic M, et al., 2008, Neurosteroid modulation of ionotropic glutamate receptors and excitatory synaptic transmission. Physiol Res, 57(Suppl 3): S49–S57. https://doi.org/33549/physiolres.931600
Paoletti P, Neyton J, Ascher P, 1995, Glycine-independent and subunit-specific potentiation of NMDA responses by extracellular Mg2+. Neuron, 15: 1109–1120. https://doi.org/1016/0896-6273(95)90099-3
Malayev A, Gibbs TT, Farb DH, 2002, Inhibition of the NMDA response by pregnenolone sulphate reveals subtype selective modulation of NMDA receptors by sulphated steroids. Br J Pharmacol, 135: 901–909. https://doi.org/1038/sj.bjp.0704543
Petrovic M, Sedlacek M, Cais O, et al., 2009, Pregnenolone sulfate modulation of N-methyl-D-aspartate receptors is phosphorylation dependent. Neuroscience, 160: 616–628. https://doi.org/1016/j.neuroscience.2009.02.052
Horak M, Vlcek K, Petrovic M, et al., 2004, Molecular mechanism of pregnenolone sulfate action at NR1/NR2B receptors. J Neurosci, 24: 10318–10325. https://doi.org/1523/JNEUROSCI.2099-04.2004
Bowlby MR, 1993, Pregnenolone sulfate potentiation of N-methyl-D-aspartate receptor channels in hippocampal neurons. Mol Pharmacol, 43: 813–819.
Wong M, Moss RL, 1994, Patch-clamp analysis of direct steroidal modulation of glutamate receptor-channels. J Neuroendocrinol, 6: 347–355. https://doi.org/1111/j.1365-2826.1994.tb00592.x
Jang MK, Mierke DF, Russek SJ, et al., 2004, A steroid modulatory domain on NR2B controls N-methyl-D-aspartate receptor proton sensitivity. Proc Natl Acad Sci U S A, 101: 8198–8203. https://doi.org/1073/pnas.0401838101
Monaghan DT, Irvine MW, Costa BM, et al., 2012, Pharmacological modulation of NMDA receptor activity and the advent of negative and positive allosteric modulators. Neurochem Int, 61: 581–592. https://doi.org/1016/j.neuint.2012.01.004
Sapkota K, Irvine MW, Fang G, et al., 2017, Mechanism and properties of positive allosteric modulation of N-methyl-d-aspartate receptors by 6-alkyl 2-naphthoic acid derivatives. Neuropharmacology, 125: 64–79. https://doi.org/1016/j.neuropharm.2017.07.007
Chopra DA, Sapkota K, Irvine MW, et al., 2017, A single-channel mechanism for pharmacological potentiation of GluN1/GluN2A NMDA receptors. Sci Rep, 7: 6933. https://doi.org/1038/s41598-017-07292-8
Irvine MW, Costa BM, Dlaboga D, et al., 2012, Piperazine- 2,3-dicarboxylic acid derivatives as dual antagonists of NMDA and GluK1-containing kainate receptors. J Med Chem, 55: 327–341. https://doi.org/1021/jm201230z
Mullasseril P, Hansen KB, Vance KM, et al., 2010, A subunit-selective potentiator of NR2C- and NR2D-containing NMDA receptors. Nat Commun, 1: 90. https://doi.org/1038/ncomms1085
Zhang X, Feng ZJ, Chergui K, 2014, Allosteric modulation of GluN2C/GluN2D-containing NMDA receptors bidirectionally modulates dopamine release: implication for Parkinson’s disease. Br J Pharmacol, 171: 3938–3945. https://doi.org/1111/bph.12758
Wang TM, Brown BM, Deng L, et al., 2017, A novel NMDA receptor positive allosteric modulator that acts via the transmembrane domain. Neuropharmacology, 121: 204–218. https://doi.org/1016/j.neuropharm.2017.04.041
Kessels HW, Nabavi S, Malinow R, 2013, Metabotropic NMDA receptor function is required for beta-amyloid-induced synaptic depression. Proc Natl Acad Sci U S A, 110: 4033–4038. https://doi.org/1073/pnas.1219605110
Ronicke R, Mikhaylova M, Rönicke S, et al., 2011, Early neuronal dysfunction by amyloid beta oligomers depends on activation of NR2B-containing NMDA receptors. Neurobiol Aging, 32: 2219–2228. https://doi.org/1016/j.neurobiolaging.2010.01.011
Li S, Jin M, Koeglsperger T, et al., 2011, Soluble Abeta oligomers inhibit long-term potentiation through a mechanism involving excessive activation of extrasynaptic NR2B-containing NMDA receptors. J Neurosci, 31: 6627–6638. https://doi.org/1523/JNEUROSCI.0203-11.2011
Hu NW, Klyubin I, Anwyl R, et al., 2009, GluN2B subunit-containing NMDA receptor antagonists prevent Abeta-mediated synaptic plasticity disruption in vivo. Proc Natl Acad Sci U S A, 106: 20504–20509. https://doi.org/1073/pnas.0908083106
Li S, Hong S, Shepardson NE, et al., 2009, Soluble oligomers of amyloid Beta protein facilitate hippocampal long-term depression by disrupting neuronal glutamate uptake. Neuron, 62: 788–801. https://doi.org/1016/j.neuron.2009.05.012
Ittner LM, Ke YD, Delerue F, et al., 2010, Dendritic function of tau mediates amyloid-beta toxicity in Alzheimer’s disease mouse models. Cell, 142: 387–397. https://doi.org/1016/j.cell.2010.06.036
Bordji K, Becerril-Ortega J, Nicole O, et al., 2010, Activation of extrasynaptic, but not synaptic, NMDA receptors modifies amyloid precursor protein expression pattern and increases amyloid-ss production. J Neurosci, 30: 15927–15942. https://doi.org/1523/JNEUROSCI.3021-10.2010
Lai TW, Shyu WC, Wang YT, 2011, Stroke intervention pathways: NMDA receptors and beyond. Trends Mol Med, 17: 266–275. https://doi.org/1016/j.molmed.2010.12.008
Dunah AW, Wang Y, Yasuda RP, et al., 2000, Alterations in subunit expression, composition, and phosphorylation of striatal N-methyl-D-aspartate glutamate receptors in a rat 6-hydroxydopamine model of Parkinson’s disease. Mol Pharmacol, 57: 342–352.
Gardoni F, Picconi B, Ghiglieri V, et al., 2006, A critical interaction between NR2B and MAGUK in L-DOPA induced dyskinesia. J Neurosci, 26: 2914–2922. https://doi.org/1523/JNEUROSCI.5326-05.2006
Mony L, Kew JN, Gunthorpe MJ, et al., 2009, Allosteric modulators of NR2B-containing NMDA receptors: Molecular mechanisms and therapeutic potential. Br J Pharmacol, 157: 1301–1317. https://doi.org/1111/j.1476-5381.2009.00304.x
Sgambato-Faure V, Cenci MA, 2012, Glutamatergic mechanisms in the dyskinesias induced by pharmacological dopamine replacement and deep brain stimulation for the treatment of Parkinson’s disease. Prog Neurobiol, 96: 69–86. https://doi.org/1016/j.pneurobio.2011.10.005
Gardoni F, Sgobio C, Pendolino V, et al., 2012, Targeting NR2A-containing NMDA receptors reduces L-DOPA-induced dyskinesias. Neurobiol Aging, 33: 2138–2144. https://doi.org/1016/j.neurobiolaging.2011.06.019
Nakazawa K, Sapkota K, 2020, The origin of NMDA receptor hypofunction in schizophrenia. Pharmacol Ther, 205: 107426. https://doi.org/1016/j.pharmthera.2019.107426
Howes OD, Kapur S, 2009, The dopamine hypothesis of schizophrenia: version III--the final common pathway. Schizophr Bull, 35: 549–562. https://doi.org/1093/schbul/sbp006
Moghaddam B, Javitt D, 2012, From revolution to evolution: the glutamate hypothesis of schizophrenia and its implication for treatment. Neuropsychopharmacology, 37: 4–15. https://doi.org/1038/npp.2011.181
Mohn AR, Gainetdinov RR, Caron MG, et al., 1999, Mice with reduced NMDA receptor expression display behaviors related to schizophrenia. Cell, 98: 427–436. https://doi.org/1016/s0092-8674(00)81972-8
Nakao K, Jeevakumar V, Jiang SZ, et al., 2019, Schizophrenia-like dopamine release abnormalities in a mouse model of NMDA receptor hypofunction. Schizophr Bull, 45: 138–147. https://doi.org/1093/schbul/sby003
Coyle JT, Tsai G, Goff D, 2003, Converging evidence of NMDA receptor hypofunction in the pathophysiology of schizophrenia. Ann N Y Acad Sci, 1003: 318–327. https://doi.org/1196/annals.1300.020
Javitt DC, Zukin SR, 1991, Recent advances in the phencyclidine model of schizophrenia. Am J Psychiatry, 148: 1301–1308. https://doi.org/1176/ajp.148.10.1301
Krystal JH, Karper LP, Seibyl JP, et al., 1994, Subanesthetic effects of the noncompetitive NMDA antagonist, ketamine, in humans. Psychotomimetic, perceptual, cognitive, and neuroendocrine responses. Arch Gen Psychiatry, 51: 199–214. https://doi.org/1001/archpsyc.1994.03950030035004
Koek W, Woods JH, Winger GD, 1988, MK-801, a proposed noncompetitive antagonist of excitatory amino acid neurotransmission, produces phencyclidine-like behavioral effects in pigeons, rats and rhesus monkeys. J Pharmacol Exp Ther, 245: 969–974.
Kovacic P, Somanathan R, 2010, Clinical physiology and mechanism of dizocilpine (MK-801): Electron transfer, radicals, redox metabolites and bioactivity. Oxid Med Cell Longev, 3: 13–22. https://doi.org/4161/oxim.3.1.10028
Dalmau J, Lancaster E, Martinez-Hernandez E, et al., 2011, Clinical experience and laboratory investigations in patients with anti-NMDAR encephalitis. Lancet Neurol, 10: 63–74. https://doi.org/1016/S1474-4422(10)70253-2
1993, A novel gene containing a trinucleotide repeat that is expanded and unstable on Huntington’s disease chromosomes. The Huntington’s Disease Collaborative Research Group. Cell, 72: 971–983. https://doi.org/1016/0092-8674(93)90585-e
Fan MM, Raymond LA, 2007, N-methyl-D-aspartate (NMDA) receptor function and excitotoxicity in Huntington’s disease. Prog Neurobiol, 81: 272–293. https://doi.org/1016/j.pneurobio.2006.11.003
Milnerwood AJ, Gladding CM, Pouladi MA, et al., 2010, Early increase in extrasynaptic NMDA receptor signaling and expression contributes to phenotype onset in Huntington’s disease mice. Neuron, 65: 178–190. https://doi.org/1016/j.neuron.2010.01.008
Okamoto S, Pouladi MA, Talantova M, et al., 2009, Balance between synaptic versus extrasynaptic NMDA receptor activity influences inclusions and neurotoxicity of mutant huntingtin. Nat Med, 15: 1407–1413. https://doi.org/1038/nm.2056
GBD 2015 Disease and Injury Incidence and Prevalence Collaborators, 2016, Global, regional, and national incidence, prevalence, and years lived with disability for 310 diseases and injuries, 1990-2015: A systematic analysis for the global burden of disease study 2015. Lancet, 388: 1545–1602. https://doi.org/1016/S0140-6736(16)31678-6
Tiller JW, 2013, Depression and anxiety. Med J Aust, 199: S28–S31. https://doi.org/5694/mja12.10628
Diefenbach GJ, McCarthy-Larzelere ME, Williamson DA, et al., 2001, Anxiety, depression, and the content of worries. Depression Anxiety, 14: 247–250. https://doi.org/1002/da.1075
Feifel D, 2016, Breaking sad: Unleashing the breakthrough potential of Ketamine’s rapid antidepressant effects. Drug Dev Res, 77: 489–494. https://doi.org/1002/ddr.21347
Fukumoto K, Toki H, Iijima M, et al., 2017, Antidepressant potential of (R)-ketamine in rodent models: Comparison with (S)-ketamine. J Pharmacol Exp Ther, 361: 9–16. https://doi.org/1124/jpet.116.239228
Ionescu DF, Fu DJ, Qiu X, et al., 2021, Esketamine nasal spray for rapid reduction of depressive symptoms in patients with major depressive disorder who have active suicide ideation with intent: results of a phase 3, double-blind, randomized study (ASPIRE II). Int J Neuropsychopharmacol, 24: 22–31. https://doi.org/1093/ijnp/pyaa068
Pittenger C, Sanacora G, Krystal JH, 2007, The NMDA receptor as a therapeutic target in major depressive disorder. CNS Neurol Disord Drug Targets, 6: 101–115. https://doi.org/2174/187152707780363267
Feyissa AM, Chandran A, Stockmeier CA, et al., 2009, Reduced levels of NR2A and NR2B subunits of NMDA receptor and PSD-95 in the prefrontal cortex in major depression. Prog Neuropsychopharmacol Biol Psychiatry, 33: 70–75. https://doi.org/1016/j.pnpbp.2008.10.005
Karolewicz B, Stockmeier CA, Ordway GA, 2005, Elevated levels of the NR2C subunit of the NMDA receptor in the locus coeruleus in depression. Neuropsychopharmacology, 30: 1557–1567. https://doi.org/1038/sj.npp.1300781
Karolewicz B, Stockmeier CA, Ordway GA, 2009, Elevated levels of NR2A and PSD-95 in the lateral amygdala in depression. Int J Neuropsychopharmacol, 12: 143–153. https://doi.org/1017/S1461145708008985
Jiang A, Su P, Li S, et al., 2021, Disrupting the alpha7nAChR-NR2A protein complex exerts antidepressant-like effects. Mol Brain, 14: 107. https://doi.org/1186/s13041-021-00817-3
Zanos P, Moaddel R, Morris PJ, et al., 2016, NMDAR inhibition-independent antidepressant actions of ketamine metabolites. Nature, 533: 481–486. https://doi.org/1038/nature17998
Bullock R, Zauner A, Woodward JJ, et al., 1998, Factors affecting excitatory amino acid release following severe human head injury. J Neurosurg, 89: 507–518. https://doi.org/3171/jns.1998.89.4.0507
Simon RP, Swan JH, Griffiths T, et al., 1984, Blockade of N-methyl-D-aspartate receptors may protect against ischemic damage in the brain. Science, 226: 850–852. https://doi.org/1126/science.6093256
Chen MH, Bullock R, Graham DI, et al., 1991, Ischemic neuronal damage after acute subdural hematoma in the rat: effects of pretreatment with a glutamate antagonist. J Neurosurg, 74: 944–950. https://doi.org/3171/jns.1991.74.6.0944
Margaill I, Parmentier S, Callebert J, et al., 1996, Short therapeutic window for MK-801 in transient focal cerebral ischemia in normotensive rats. J Cerebral Blood Flow Metab, 16: 107–113. https://doi.org/1097/00004647-199601000-00013
Liu Y, Wong TP, Aarts M, et al., 2007, NMDA receptor subunits have differential roles in mediating excitotoxic neuronal death both in vitro and in vivo. J Neurosci, 27: 2846–2857. https://doi.org/1523/JNEUROSCI.0116-07.2007
Chen M, Lu TJ, Chen XJ, et al., 2008, Differential roles of NMDA receptor subtypes in ischemic neuronal cell death and ischemic tolerance. Stroke, 39: 3042–3048. https://doi.org/1161/STROKEAHA.108.521898
Yang Y, Li Q, Yang T, et al., 2003, Reduced brain infarct volume and improved neurological outcome by inhibition of the NR2B subunit of NMDA receptors by using CP101,606-27 alone and in combination with rt-PA in a thromboembolic stroke model in rats. J Neurosurg, 98: 397–403. https://doi.org/3171/jns.2003.98.2.0397
Aarts M, Liu Y, Liu L, et al., 2002, Treatment of ischemic brain damage by perturbing NMDA receptor- PSD-95 protein interactions. Science, 298: 846–850. https://doi.org/1126/science.1072873
Cook DJ, Teves L, Tymianski M, 2012, A translational paradigm for the preclinical evaluation of the stroke neuroprotectant Tat-NR2B9c in gyrencephalic nonhuman primates. Sci Transl Med, 4: 154ra133. https://doi.org/1126/scitranslmed.3003824
Hill MD, Martin RH, Mikulis D, et al., 2012, Safety and efficacy of NA-1 in patients with iatrogenic stroke after endovascular aneurysm repair (ENACT): A phase 2, randomised, double-blind, placebo-controlled trial. Lancet Neurol, 11: 942–950. https://doi.org/1016/S1474-4422(12)70225-9
Beauchamp K, Mutlak H, Smith WR, et al., 2008, Pharmacology of traumatic brain injury: Where is the “golden bullet”? Mol Med, 14: 731–740. https://doi.org/2119/2008-00050.Beauchamp
Biegon A, Fry PA, Paden CM, et al., 2004, Dynamic changes in N-methyl-D-aspartate receptors after closed head injury in mice: Implications for treatment of neurological and cognitive deficits. Proc Natl Acad Sci U S A, 101: 5117–5122. https://doi.org/1073/pnas.0305741101
Carter DS, Deshpande LS, Rafiq A, et al., 2011, Characterization of spontaneous recurrent epileptiform discharges in hippocampal-entorhinal cortical slices prepared from chronic epileptic animals. Seizure, 20: 218–224. https://doi.org/1016/j.seizure.2010.11.022
Meldrum BS, 1993, Excitotoxicity and selective neuronal loss in epilepsy. Brain Pathol, 3: 405–412. https://doi.org/1111/j.1750-3639.1993.tb00768.x
Jensen PJ, Millan N, Mack KJ, 1997, Cortical NMDAR-1 gene expression is rapidly upregulated after seizure. Brain Res Mol Brain Res, 44: 157–162. https://doi.org/1016/s0169-328x(96)00262-8
Kikuchi S, Iwasa H, Sato T, 2000, Lasting changes in NMDAR1 mRNA level in various regions of cerebral cortex in epileptogenesis of amygdaloid-kindled rat. Psychiatry Clin Neurosci, 54: 573–577. https://doi.org/1046/j.1440-1819.2000.00755.x
Gerfin-Moser A, Grogg F, Rietschin L, et al., 1995, Alterations in glutamate but not GABAA receptor subunit expression as a consequence of epileptiform activity in vitro. Neuroscience, 67: 849-865. https://doi.org/1016/0306-4522(95)00130-b
Mathern GW, Pretorius JK, Leite JP, et al., 1998, Hippocampal AMPA and NMDA mRNA levels and subunit immunoreactivity in human temporal lobe epilepsy patients and a rodent model of chronic mesial limbic epilepsy. Epilepsy Res, 32: 154–171. https://doi.org/1016/s0920-1211(98)00048-5
Lason W, Turchan J, Przewłocki R, et al., 1997, Effects of pilocarpine and kainate-induced seizures on N-methyl-D-aspartate receptor gene expression in the rat hippocampus. Neuroscience, 78: 997–1004. https://doi.org/1016/s0306-4522(96)00635-5
Hosain S, Nagarajan L, Carson D, et al., 1997, Felbamate for refractory infantile spasms. J Child Neurol, 12: 466–468. https://doi.org/1177/088307389701200711
Zupanc ML, Werner RR, Schwabe MS, et al., 2010, Efficacy of felbamate in the treatment of intractable pediatric epilepsy. Pediatr Neurol, 42: 396–403. https://doi.org/1016/j.pediatrneurol.2010.02.013
McCabe RT, Wasterlain CG, Kucharczyk N, et al., 1993, Evidence for anticonvulsant and neuroprotectant action of felbamate mediated by strychnine-insensitive glycine receptors. J Pharmacol Exp Ther, 264: 1248–1252.
White HS, Harmsworth WL, Sofia RD, et al., 1995, Felbamate modulates the strychnine-insensitive glycine receptor. Epilepsy Res, 20: 41–48. https://doi.org/1016/0920-1211(94)00066-6
Rho JM, Donevan SD, Rogawski MA, 1994, Mechanism of action of the anticonvulsant felbamate: opposing effects on N-methyl-D-aspartate and gamma-aminobutyric acidA receptors. Ann Neurol, 35: 229–234. https://doi.org/1002/ana.410350216
Singh L, Oles RJ, Vass CA, et al., 1991, A slow intravenous infusion of N-methyl-DL-aspartate as a seizure model in the mouse. J Neurosci Methods, 37: 227–232. https://doi.org/1016/0165-0270(91)90028-x
Balosso S, Maroso M, Sanchez-Alavez M, et al., 2008, A novel non-transcriptional pathway mediates the proconvulsive effects of interleukin-1beta. Brain, 131: 3256–3265. https://doi.org/1093/brain/awn271
Yen W, Williamson J, Bertram EH, et al., 2004, A comparison of three NMDA receptor antagonists in the treatment of prolonged status epilepticus. Epilepsy Res, 59: 43–50. https://doi.org/1016/j.eplepsyres.2004.03.004
Kleinrok Z, Turski WA, Czuczwar SJ, 1995, Excitatory amino acid antagonists and the anticonvulsive activity of conventional antiepileptic drugs. Polish J Pharmacol, 47: 247–252.
Brackett RL, Pouw B, Blyden JF, et al., 2000, Prevention of cocaine-induced convulsions and lethality in mice: Effectiveness of targeting different sites on the NMDA receptor complex. Neuropharmacology, 39: 407–418. https://doi.org/1016/s0028-3908(99)00151-3
Vataev SI, Zhabko EP, Lukomskaia N, et al., 2009, Effects of memantine on convulsive reactions and sleep-waking cycle in Krushinskii-Molodkina strain rats with the inherited predisposition to audiogenic convulsions. Ross Fiziol Zh Im I M Sechenova, 95: 802–812.
Frey HH, Voits M, 1991, Effect of psychotropic agents on a model of absence epilepsy in rats. Neuropharmacology, 30: 651–656. https://doi.org/1016/0028-3908(91)90086-q
Czuczwar SJ, Turski WA, Kleinrok Z, 1996, Interactions of excitatory amino acid antagonists with conventional antiepileptic drugs. Metab Brain Dis, 11: 143–152. https://doi.org/1007/BF02069501
Wu LJ, Zhuo M, 2009, Targeting the NMDA receptor subunit NR2B for the treatment of neuropathic pain. Neurotherapeutics, 6: 693–702. https://doi.org/1016/j.nurt.2009.07.008
Woolf CJ, Salter MW, Neuronal plasticity: increasing the gain in pain. Science, 288: 1765–1769. https://doi.org/1126/science.288.5472.1765
Isaev D, Gerber G, Park SK, et al., 2000, Facilitation of NMDA-induced currents and Ca2+ transients in the rat substantia gelatinosa neurons after ligation of L5-L6 spinal nerves. Neuroreport, 11: 4055–4061. https://doi.org/1097/00001756-200012180-00030
Ultenius C, Linderoth B, Meyerson BA, et al., 2006, Spinal NMDA receptor phosphorylation correlates with the presence of neuropathic signs following peripheral nerve injury in the rat. Neurosci Lett, 399: 85–90. https://doi.org/1016/j.neulet.2006.01.018
Gao X, Kim HK, Chung JM, et al., 2005, Enhancement of NMDA receptor phosphorylation of the spinal dorsal horn and nucleus gracilis neurons in neuropathic rats. Pain, 116: 62–72. https://doi.org/1016/j.pain.2005.03.045
Suzuki R, Matthews EA, Dickenson AH, 2001, Comparison of the effects of MK-801, ketamine and memantine on responses of spinal dorsal horn neurones in a rat model of mononeuropathy. Pain, 91: 101–109. https://doi.org/1016/s0304-3959(00)00423-1
Iwata H, Takasusuki T, Yamaguchi S, et al., 2007, NMDA receptor 2B subunit-mediated synaptic transmission in the superficial dorsal horn of peripheral nerve-injured neuropathic mice. Brain Res, 1135: 92–101. https://doi.org/1016/j.brainres.2006.12.014
Correll GE, Maleki J, Gracely EJ, et al., 2004, Subanesthetic ketamine infusion therapy: A retrospective analysis of a novel therapeutic approach to complex regional pain syndrome. Pain Med, 5: 263–275. https://doi.org/1111/j.1526-4637.2004.04043.x.
Kiefer RT, Rohr P, Ploppa A, et al., 2008, Efficacy of ketamine in anesthetic dosage for the treatment of refractory complex regional pain syndrome: An open-label phase II study. Pain Med, 9: 1173–1201. https://doi.org/1111/j.1526-4637.2007.00402.x
Duale C, Sibaud F, Guastella V, et al., 2009, Perioperative ketamine does not prevent chronic pain after thoracotomy. Eur J Pain, 13: 497–505. https://doi.org/1016/j.ejpain.2008.06.013
Pud D, Eisenberg E, Spitzer A, et al., 1998, The NMDA receptor antagonist amantadine reduces surgical neuropathic pain in cancer patients: A double blind, randomized, placebo controlled trial. Pain, 75: 349–354. https://doi.org/1016/s0304-3959(98)00014-1
Fukui S, Komoda Y, Nosaka S, 2001, Clinical application of amantadine, an NMDA antagonist, for neuropathic pain. J Anesth, 15: 179–181. https://doi.org/1007/s005400170025
Lancaster CL, Teeters JB, Gros DF, et al., 2016, Posttraumatic stress disorder: Overview of evidence-based assessment and treatment. J Clin Med, 5: 105. https://doi.org/3390/jcm5110105
Gates MA, Holowka DW, Vasterling JJ, et al., 2012, Posttraumatic stress disorder in veterans and military personnel: Epidemiology, screening, and case recognition. Psychol Serv, 9: 361–382. https://doi.org/1037/a0027649
Yuan K, Gong YM, Liu L, et al., Prevalence of posttraumatic stress disorder after infectious disease pandemics in the twenty-first century, including COVID-19: A meta-analysis and systematic review. Mol Psychiatry, 26: 4982–4998. https://doi.org/1038/s41380-021-01036-x
Sofuoglu M, Rosenheck R, Petrakis I, 2014, Pharmacological treatment of comorbid PTSD and substance use disorder: Recent progress. Addict Behav, 39: 428–433. https://doi.org/1016/j.addbeh.2013.08.014
Bestha D, Soliman L, Blankenship K, et al., 2018, The walking wounded: Emerging treatments for PTSD. Curr Psychiatry Rep, 20: 94. https://doi.org/1007/s11920-018-0941-8
Krystal JH, Abdallah CG, Averill LA, et al., 2017, Synaptic loss and the pathophysiology of PTSD: Implications for ketamine as a prototype novel therapeutic. Curr Psychiatry Rep, 19: 74. https://doi.org/1007/s11920-017-0829-z
Feder A, Parides MK, Murrough JW, et al. Efficacy of intravenous ketamine for treatment of chronic posttraumatic stress disorder: A randomized clinical trial. JAMA Psychiatry, 71: 681–688. https://doi.org/1001/jamapsychiatry.2014.62
McGhee LL, Maani CV, Garza TH, et al., 2008, The correlation between ketamine and posttraumatic stress disorder in burned service members. J Trauma, 64: S195–8; discussion S197–8. https://doi.org/1097/TA.0b013e318160ba1d
Zhang LM, Zhou WW, Ji YJ, et al., 2015, Anxiolytic effects of ketamine in animal models of posttraumatic stress disorder. Psychopharmacology, 232: 663–672. https://doi.org/1007/s00213-014-3697-9
Zimmerman JM, Maren S, 2010, NMDA receptor antagonism in the basolateral but not central amygdala blocks the extinction of Pavlovian fear conditioning in rats. Eur J Neurosci, 31: 1664–1670. https://doi.org/1111/j.1460-9568.2010.07223.x
Liu JL, Li M, Dang XR, et al., 2009, A NMDA receptor antagonist, MK-801 impairs consolidating extinction of auditory conditioned fear responses in a Pavlovian model. PLoS One, 4: e7548. https://doi.org/1371/journal.pone.0007548
Fry AE, Fawcett KA, Zelnik N, et al., 2018, De novo mutations in GRIN1 cause extensive bilateral polymicrogyria. Brain, 141: 698–712. https://doi.org/1093/brain/awx358
Yuan H, Low CM, Moody OA, et al., 2015, Ionotropic GABA and glutamate receptor mutations and human neurologic diseases. Mol Pharmacol, 88: 203–217. https://doi.org/1124/mol.115.097998
Hamdan FF, Gauthier J, Araki Y, et al., 2011, Excess of de novo deleterious mutations in genes associated with glutamatergic systems in nonsyndromic intellectual disability. Am J Hum Genet, 88: 306–316. https://doi.org/1016/j.ajhg.2011.02.001
Lemke JR, Geider K, Helbig KL, et al., 2016, Delineating the GRIN1 phenotypic spectrum: A distinct genetic NMDA receptor encephalopathy. Neurology, 86: 2171–2178. https://doi.org/1212/WNL.0000000000002740
Chen W, Shieh C, Swanger SA, et al., 2017, GRIN1 mutation associated with intellectual disability alters NMDA receptor trafficking and function. J Hum Genet, 62: 589–597. https://doi.org/1038/jhg.2017.19
Xu XX, Luo JH, 2018, Mutations of N-methyl-D-aspartate receptor subunits in epilepsy. Neurosci Bull, 34: 549–565. https://doi.org/1007/s12264-017-0191-5
Ohba C, Shiina M, Tohyama J, et al., 2015, GRIN1 mutations cause encephalopathy with infantile-onset epilepsy, and hyperkinetic and stereotyped movement disorders. Epilepsia, 56: 841–848. https://doi.org/1111/epi.12987
Pierson TM, Yuan H, Marsh ED, et al., 2014, GRIN2A mutation and early-onset epileptic encephalopathy: Personalized therapy with memantine. Ann Clin Transl Neurol, 1: 190-198. https://doi.org/1002/acn3.39
Carvill GL, Regan BM, Yendle SC, et al., 2013, GRIN2A mutations cause epilepsy-aphasia spectrum disorders. Nat Genet, 45: 1073–1076. https://doi.org/1038/ng.2727
Lemke JR, Lal D, Reinthaler EM, et al., 2013, Mutations in GRIN2A cause idiopathic focal epilepsy with rolandic spikes. Nat Genet, 45: 1067–1072. https://doi.org/1038/ng.2728
Lesca G, Rudolf G, Bruneau N, et al., 2013, GRIN2A mutations in acquired epileptic aphasia and related childhood focal epilepsies and encephalopathies with speech and language dysfunction. Nat Genet, 45: 1061–1066. https://doi.org/1038/ng.2726
Xu XX, Liu XR, Fan CY, et al., 2018, Functional investigation of a GRIN2A variant associated with rolandic epilepsy. Neurosci Bull, 34: 237–246. https://doi.org/1007/s12264-017-0182-6
Yuan H, Tankovic A, Burger PB, et al., 2014, Functional analysis of a de novo GRIN2A missense mutation associated with early-onset epileptic encephalopathy. Nat Commun, 5: 3251. https://doi.org/1038/ncomms4251
Chen W, et al., 2017, Functional evaluation of a de novo GRIN2A mutation identified in a patient with profound global developmental delay and refractory epilepsy. Mol Pharmacol, 91: 317–330. https://doi.org/1124/mol.116.106781
Endele S, Rosenberger G, Geider K, et al., 2010, Mutations in GRIN2A and GRIN2B encoding regulatory subunits of NMDA receptors cause variable neurodevelopmental phenotypes. Nat Genet, 42: 1021–1026. https://doi.org/1038/ng.677
Hansen KB, Yi F, Perszyk RE, et al., 2018, Structure, function, and allosteric modulation of NMDA receptors. J Gen Physiol, 150: 1081–1105. https://doi.org/1085/jgp.201812032
Wollmuth LP, Kuner T, Sakmann B, 1998, Adjacent asparagines in the NR2-subunit of the NMDA receptor channel control the voltage-dependent block by extracellular Mg2+. J Physiol, 506(Pt 1): 13–32. https://doi.org/1111/j.1469-7793.1998.013bx.x
Mayer ML, 2006, Glutamate receptors at atomic resolution. Nature, 440: 456–462. https://doi.org/1038/nature04709
Gao K, Tankovic A, Zhang Y, et al., 2017, A de novo loss-of-function GRIN2A mutation associated with childhood focal epilepsy and acquired epileptic aphasia. PLoS One, 12: e0170818. https://doi.org/1371/journal.pone.0170818
Swanger SA, Chen W, Wells G, et al., 2016, Mechanistic insight into nmda receptor dysregulation by rare variants in the GluN2A and GluN2B agonist binding domains. Am J Hum Genet, 99: 1261–1280. https://doi.org/1016/j.ajhg.2016.10.002
Vieira MM, Nguyen TA, Wu K, et al., 2020, An epilepsy-associated GRIN2A rare variant disrupts CaMKIIalpha phosphorylation of GluN2A and NMDA receptor trafficking. Cell Rep, 32: 108104. https://doi.org/1016/j.celrep.2020.108104
Fedele L, Newcombe J, Topf M, et al., 2018, Disease-associated missense mutations in GluN2B subunit alter NMDA receptor ligand binding and ion channel properties. Nat Commun, 9: 957. https://doi.org/1038/s41467-018-02927-4
Glasgow NG, Retchless BS, Johnson JW, 2015, Molecular bases of NMDA receptor subtype-dependent properties. J Physiol, 593: 83–95. https://doi.org/1113/jphysiol.2014.273763
Andreoli V, De Marco EV, Trecroci F, et al., 2014, Potential involvement of GRIN2B encoding the NMDA receptor subunit NR2B in the spectrum of Alzheimer’s disease. J Neural Transm, 121: 533–542. https://doi.org/1007/s00702-013-1125-7
Myers RA, Casals F, Gauthier J, et al., 2011, A population genetic approach to mapping neurological disorder genes using deep resequencing. PLoS Genet, 7: e1001318. https://doi.org/1371/journal.pgen.1001318
Hu C, Chen W, Myers SJ, et al., 2016, Human GRIN2B variants in neurodevelopmental disorders. J Pharmacol Sci, 132: 115–121. https://doi.org/1016/j.jphs.2016.10.002
Tarabeux J, Kebir O, Gauthier J, et al., 2011, Rare mutations in N-methyl-D-aspartate glutamate receptors in autism spectrum disorders and schizophrenia. Transl Psychiatry, 1: e55. https://doi.org/1038/tp.2011.52
Retterer K, Juusola J, Cho MT, et al., 2016, Clinical application of whole-exome sequencing across clinical indications. Genet Med, 18: 696–704. https://doi.org/1038/gim.2015.148
Wells G, Yuan H, McDaniel MJ, et al., 2018, The GluN2B-Glu413Gly NMDA receptor variant arising from a de novo GRIN2B mutation promotes ligand-unbinding and domain opening. Proteins, 86: 1265–1276. https://doi.org/1002/prot.25595
Shin W, Kim K, Serraz B, et al., 2020, Early correction of synaptic long-term depression improves abnormal anxiety-like behavior in adult GluN2B-C456Y-mutant mice. PLoS Biol, 18: e3000717. https://doi.org/1371/journal.pbio.3000717
Soto D, Olivella M, Grau C, et al., 2019, L-Serine dietary supplementation is associated with clinical improvement of loss-of-function GRIN2B-related pediatric encephalopathy. Sci Signal, 12: eaaw0936. https://doi.org/1126/scisignal.aaw0936
Lemke JR, Hendrickx R, Geider K, et al., 2014, GRIN2B mutations in West syndrome and intellectual disability with focal epilepsy. Ann Neurol, 75: 147–154. https://doi.org/1002/ana.24073
Mullier B, Wolff C, Sands ZA, et al., 2017, GRIN2B gain of function mutations are sensitive to radiprodil, a negative allosteric modulator of GluN2B-containing NMDA receptors. Neuropharmacology, 123: 322–331. https://doi.org/1016/j.neuropharm.2017.05.017
Li D, Yuan H, Ortiz-Gonzalez XR, et al., 2016, GRIN2D recurrent de novo dominant mutation causes a severe epileptic encephalopathy treatable with NMDA receptor channel blockers. Am J Human Genet, 99: 802–816. https://doi.org/1016/j.ajhg.2016.07.013
Chen HS, Lipton SA, 2006, The chemical biology of clinically tolerated NMDA receptor antagonists. J Neurochem, 97: 1611–1626. https://doi.org/1111/j.1471-4159.2006.03991.x