MicroRNA-195 rescues the impaired MS-dCA1 neural circuit and spatial memory in rats with chronic cerebral hypoperfusion
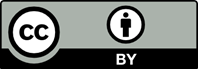
Chronic cerebral hypoperfusion (CCH) is a common risk factor for vascular dementia and Alzheimer’s disease (AD). The previous studies have shown that CCH-induced multiple AD-like pathological changes in the hippocampus and cortex through downregulating the microRNA-195 (miR-195) expression. However, whether and how miR-195 participates in the dysfunction of the medial septum (MS)-dorsal hippocampal CA1 (dCA1) neural circuit following CCH is still obscure. In the present study, we found that miR-195 was downregulated in the MS region of CCH rats. Moreover, using electrophysiological recording and immunofluorescence staining technique, we found that the knockdown of miR-195 through the injection of lenti- AMO-195 into the MS region led to a pathological change that mimicked the damage to the MS-dCA1 neural circuit in CCH rats, including the decreased input-output (I/O) curve, increased paired-pulse ratio (PPR), decreased numbers of ChAT+ and PV+ neurons in MS, and diminished theta rhythm in the hippocampus. More importantly, exogenously supplemented miR-195 into the MS region rescued the damaged neural circuit in MS-dCA1 of CCH rats by injecting lenti-pre-miR-195. Gain-of-function of miR-195 in the MS region significantly improved the cognitive dysfunction in CCH rats assessed by the Morris water maze test. In conclusion, knockdown of miR-195 in the MS region can impair MS-dCA1 neural circuit function, while upregulation of miR- 195 can rescue the impaired function of MS-dCA1 neural circuit and spatial memory ability in CCH rats. This provides a valuable reference for future anti-dementia therapy involving miR-195.
Santos CY, Snyder PJ, Wu WC, et al., 2017, Pathophysiologic relationship between Alzheimer’s disease, cerebrovascular disease, and cardiovascular risk: A review and synthesis. Alzheimers Dement (Amst), 7: 69–87.
O’Brien JT, Thomas A, 2015, Vascular dementia. Lancet, 386(10004): 1698–706.
Zhao Y, Gong CX, 2015, From chronic cerebral hypoperfusion to Alzheimer-like brain pathology and neurodegeneration. Cell Mol Neurobiol, 35(1): 101–110. https://doi.org/10.1007/s10571-014-0127-9
Wang X, Xing A, Xu C, et al., 2010, Cerebrovascular hypoperfusion induces spatial memory impairment, synaptic changes, and amyloid-β oligomerization in rats. J Alzheimers Dis, 21(3): 813–822. https://doi.org/10.3233/jad-2010-100216
Choi BR, Lee SR, Han JS, et al., 2011, Synergistic memory impairment through the interaction of chronic cerebral hypoperfusion and amlyloid toxicity in a rat model. Stroke, 42(9): 2595–2604. https://doi.org/10.1161/strokeaha.111.620179
Zhao Q, Murakami Y, Tohda M, et al., 2007, Chotosan, a kampo formula, ameliorates chronic cerebral hypoperfusion-induced deficits in object recognition behaviors and central cholinergic systems in mice. J Pharmacol Sci, 103(4): 360–373. https://doi.org/10.1254/jphs.fp0061457
Ai J, Sun LH, Che H, et al., 2013, MicroRNA-195 protects against dementia induced by chronic brain hypoperfusion via its anti-amyloidogenic effect in rats. J Neurosci, 33(9): 3989–4001. https://doi.org/10.1523/jneurosci.1997-12.2013
Chen X, Jiang XM, Zhao LJ, et al., 2017, MicroRNA-195 prevents dendritic degeneration and neuron death in rats following chronic brain hypoperfusion. Cell Death Dis, 8(6): e2850. https://doi.org/10.1038/cddis.2017.243
CD, Wang Q, Zong K, et al., 2016, Knockdown of microRNA-195 contributes to protein phosphatase-2A inactivation in rats with chronic brain hypoperfusion. Neurobiol Aging, 45: 76–87. https://doi.org/10.1016/j.neurobiolaging.2016.05.010
Mao M, Xu Y, Zhang XY, et al., 2020, MicroRNA-195 prevents hippocampal microglial/macrophage polarization towards the M1 phenotype induced by chronic brain hypoperfusion through regulating CX3CL1/CX3CR1 signaling. J Neuroinflammation, 17(1): 244. https://doi.org/10.1186/s12974-020-01919-w
Yan ML, Zhang S, Zhao HM, et al., 2020, MicroRNA-153 impairs presynaptic plasticity by blocking vesicle release following chronic brain hypoperfusion. Cell Commun Signal, 18(1): 57. https://doi.org/10.1186/s12964-020-00551-8
Xu Y, Zhang S, Sun Q, et al., 2020, Cholinergic dysfunction involvement in chronic cerebral hypoperfusion-induced impairment of medial septum-dCA1 neurocircuit in rats. Front Cell Neurosci, 14: 586591. https://doi.org/10.3389/fncel.2020.586591
Huh CY, Goutagny R, Williams S, 2010, Glutamatergic neurons of the mouse medial septum and diagonal band of Broca synaptically drive hippocampal pyramidal cells: Relevance for hippocampal theta rhythm. J Neurosci, 30(47): 15951–15961. https://doi.org/10.1523/jneurosci.3663-10.2010
Hangya B, Borhegyi Z, Szilágyi N, et al., 2009, GABAergic neurons of the medial septum lead the hippocampal network during theta activity. J Neurosci, 29(25): 8094–8102. https://doi.org/10.1523/jneurosci.5665-08.2009
Newman EL, Gillet SN, Climer JR, et al., 2013, Cholinergic blockade reduces theta-gamma phase amplitude coupling and speed modulation of theta frequency consistent with behavioral effects on encoding. J Neurosci, 33(50): 19635–19646. https://doi.org/10.1523/jneurosci.2586-13.2013
Nuñez A, Buño W, 2021, The theta rhythm of the hippocampus: From neuronal and circuit mechanisms to behavior. Front Cell Neurosci, 15: 649262. https://doi.org/10.3389/fncel.2021.649262
Schmitz TW, Spreng RN, 2016, Basal forebrain degeneration precedes and predicts the cortical spread of Alzheimer’s pathology. Nat Commun, 7: 13249. https://doi.org/10.1038/ncomms13249
Provost P, 2010, Interpretation and applicability of microRNA data to the context of Alzheimer’s and age-related diseases. Aging (Albany NY), 2(3): 166–169. https://doi.org/10.18632/aging.100131
Takousis P, Sadlon A, Schulz J, et al., 2019, Differential expression of microRNAs in Alzheimer’s disease brain, blood, and cerebrospinal fluid. Alzheimers Dement, 15(11): 1468–1477.
Boissonneault V, Plante I, Rivest S, et al., 2009, MicroRNA-298 and microRNA-328 regulate expression of mouse beta-amyloid precursor protein-converting enzyme 1. J Biol Chem, 284(4): 1971–1981. https://doi.org/10.1074/jbc.m807530200
Hébert SS, Horré K, Nicolaï L, et al., 2009, MicroRNA regulation of Alzheimer’s amyloid precursor protein expression. Neurobiol Dis, 33(3): 422–428.
Zong Y, Wang H, Dong W, et al., 2011, miR-29c regulates BACE1 protein expression. Brain Res, 1395: 108–115. https://doi.org/10.1016/j.brainres.2011.04.035
Smith P, Al Hashimi A, Girard J, et al., 2011, In vivo regulation of amyloid precursor protein neuronal splicing by microRNAs. J Neurochem, 116(2): 240–247. https://doi.org/10.1111/j.1471-4159.2010.07097.x
Cao J, Huang M, Guo L, et al., 2021, MicroRNA-195 rescues ApoE4-induced cognitive deficits and lysosomal defects in Alzheimer’s disease pathogenesis. Mol Psychiatry, 26(9): 4687–4701. https://doi.org/10.1038/s41380-020-0824-3
Chang L, Zhang W, Shi S, et al., microRNA-195 attenuates neuronal apoptosis in rats with ischemic stroke through inhibiting KLF5-mediated activation of the JNK signaling pathway. Mol Med, 26(1): 31. https://doi.org/10.1186/s10020-020-00150-w
Ren X, Wang Z, Guo C, 2021, MiR-195-5p ameliorates cerebral ischemia-reperfusion injury by regulating the PTEN-AKT signaling pathway. Neuropsychiatr Dis Treat, 17: 1231–1242. https://doi.org/10.2147/ndt.s297975
Cheng HY, Wang YS, Hsu PY, et al., 2019, miR-195 has a potential to treat ischemic and hemorrhagic stroke through neurovascular protection and neurogenesis. Mol Ther Methods Clin Dev, 13: 121–132. https://doi.org/10.1016/j.omtm.2018.11.011
Yang J, Hu ZL, Jiang B, et al., 2011, Effect of chloramine-T on long-term potentiation at synapses between perforant path and dentate gyrus in hippocampus of rats in vivo. Neurotoxicology, 32(2): 199–205. https://doi.org/10.1016/j.neuro.2011.01.006
Jedlicka P, Schwarzacher SW, Winkels R, et al., 2009, Impairment of in vivo theta-burst long-term potentiation and network excitability in the dentate gyrus of synaptopodin-deficient mice lacking the spine apparatus and the cisternal organelle. Hippocampus, 19(2): 130–140. https://doi.org/10.1002/hipo.20489
Varga V, Hangya B, Kránitz K, et al., 2008, The presence of pacemaker HCN channels identifies theta rhythmic GABAergic neurons in the medial septum. J Physiol, 586(16): 3893–3915. https://doi.org/10.1113/jphysiol.2008.155242
Liu XJ, Yuan L, Yang D, et al., 2013, Melatonin protects against amyloid-β-induced impairments of hippocampal LTP and spatial learning in rats. Synapse, 67(9): 626–636. https://doi.org/10.1002/syn.21677
Stevens WD, Fortin T, Pappas BA, 2002, Retinal and optic nerve degeneration after chronic carotid ligation: Time course and role of light exposure. Stroke, 33(4): 1107–1112. https://doi.org/10.1161/01.str.0000014204.05597.0c
Regehr WG, 2012, Short-term presynaptic plasticity. Cold Spring Harb Perspect Biol, 4: a005702. https://doi.org/10.1101/cshperspect.a005702
Deng M, Zhang Q, Wu Z, et al., 2020, Mossy cell synaptic dysfunction causes memory imprecision via miR-128 inhibition of STIM2 in Alzheimer’s disease mouse model. Aging Cell, 19: e13144. https://doi.org/10.1111/acel.13327
Sun Y, Nguyen AQ, Nguyen JP, et al., 2014, Cell-type-specific circuit connectivity of hippocampal CA1 revealed through Cre-dependent rabies tracing. Cell Rep, 7(1): 269–280. https://doi.org/10.1016/j.celrep.2014.02.030
Vandecasteele M, Varga V, Berényi A, et al., 2014, Optogenetic activation of septal cholinergic neurons suppresses sharp wave ripples and enhances theta oscillations in the hippocampus. Proc Natl Acad Sci U S A, 111(37): 13535–13540. https://doi.org/10.1073/pnas.1411233111
Zhang H, Lin SC, Nicolelis MA, 2010, Spatiotemporal coupling between hippocampal acetylcholine release and theta oscillations in vivo. J Neurosci, 30(40): 13431–13440. https://doi.org/10.1523/jneurosci.1144-10.2010
Buzsáki G, 2002, Theta oscillations in the hippocampus. Neuron, 33(3): 325–340. https://doi.org/10.1016/s0896-6273(02)00586-x
Cummings JL, Tong G, Ballard C, 2019, Treatment combinations for Alzheimer’s disease: Current and future pharmacotherapy options. J Alzheimers Dis, 67(3): 779–794. https://doi.org/10.3233/jad-180766
Aitta-Aho T, Hay YA, Phillips BU, et al., 2018, Basal forebrain and brainstem cholinergic neurons differentially impact amygdala circuits and learning-related behavior. Curr Biol, 28(16): 2557–2569.e4. https://doi.org/10.1016/j.cub.2018.06.064
Szeto JY, Lewis SJ, 2016, Current treatment options for Alzheimer’s disease and Parkinson’s disease dementia. Curr Neuropharmacol, 14(4): 326–338.
Giacchino J, Criado JR, Games D, et al., 2000, In vivo synaptic transmission in young and aged amyloid precursor protein transgenic mice. Brain Res, 876(1–2): 185–190. https://doi.org/10.1016/s0006-8993(00)02615-9
Oddo S, Caccamo A, Shepherd JD, et al., 2003, Triple-transgenic model of Alzheimer’s disease with plaques and tangles: Intracellular Abeta and synaptic dysfunction. Neuron, 39(3): 409–421.
Shankar GM, Li S, Mehta TH, et al., 2008, Amyloid-beta protein dimers isolated directly from Alzheimer’s brains impair synaptic plasticity and memory. Nat Med, 14(8): 837–842. https://doi.org/10.3410/f.1115634.571693
Li Q, Yang C, Zhang X, et al., 2019, Arginine vasopressin attenuates dysfunction of hippocampal theta and gamma oscillations in chronic cerebral hypoperfusion via V1a receptor. Brain Res Bull, 153: 84–92. https://doi.org/10.1016/j.brainresbull.2019.08.012
Gu Z, Yakel JL, 2011, Timing-dependent septal cholinergic induction of dynamic hippocampal synaptic plasticity. Neuron, 71(1): 155–165. https://doi.org/10.1016/j.neuron.2011.04.026
Woolf NJ, 1991, Cholinergic systems in mammalian brain and spinal cord. Prog Neurobiol, 37(6): 475–524. https://doi.org/10.1016/0301-0082(91)90006-m
Freund TF, Antal M, 1988, GABA-containing neurons in the septum control inhibitory interneurons in the hippocampus. Nature, 336(6195): 170–173. https://doi.org/10.1038/336170a0
Freund TF, Katona I, 2007, Perisomatic inhibition. Neuron, 56(1): 33–42. https://doi.org/10.1016/j.neuron.2007.09.012
Tóth K, Freund TF, Miles R, 1997, Disinhibition of rat hippocampal pyramidal cells by GABAergic afferents from the septum. J Physiol, 500(Pt 2): 463–474. https://doi.org/10.1113/jphysiol.1997.sp022033
Loreth D, Ozmen L, Revel FG, et al., 2012, Selective degeneration of septal and hippocampal GABAergic neurons in a mouse model of amyloidosis and tauopathy. Neurobiol Dis, 47(1): 1–12. https://doi.org/10.1016/j.nbd.2012.03.011