Discovery of new antibiotics using AI-guided spectroscopy and 3D drug-protein computer simulation technologies to combat MDR bacteria-associated mortality
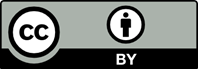
Multidrug-resistant (MDR), extensively drug-resistant (XDR), and totally drug-resistant bacteria can cause sepsis and death in patients due to their ability to inactivate most antibiotics, including ampicillin, tetracycline, streptomycin, chloramphenicol, erythromycin, and ciprofloxacin. This paper aims to review recent advancements in synthetic antibiotics, lantibiotics, and phytoantibiotics and to present our research on phytoantibiotics, specifically focusing on CU1 and NU2. While third- and fifth-generation synthetic antibiotics such as meropenem, moxifloxacin, amikacin, and tigecycline are currently relied upon for treating MDR infections, research is underway to develop peptide antibiotics known as lantibiotics (e.g., nisins, bacteriocins, and salivaricins). Lantibiotics such as nisin-A and salivaricin-B have demonstrated efficacy in curing numerous MDR infections, while phytochemicals such as artemisinin and quinine have shown effectiveness against chloroquine-resistant Plasmodium falciparum infections (malaria). In our study, we utilized techniques such as mass spectroscopy, nuclear magnetic resonance, and Fourier transform infrared spectroscopy in conjunction with artificial intelligence (AI) and computer simulation technologies to determine the structure of phytochemicals. Our results revealed that CU1, derived from Cassia fistula bark ethanol extract, exhibits potent antibiotic activity against XDR bacteria by targeting the RNA polymerases of Escherichia coli and Mycobacterium tuberculosis. Consequently, our MDR-Cure extract containing CU1 represents a promising antibacterial Ayurvedic medicine specifically tailored for skin and nail infections. Similarly, NU2 poly-fluorophosphate-glycosides from Suregada multiflora roots ethanol extract exhibited strong inhibitory effects on XDR bacteria by targeting DNA topoisomerase I. Recently, many cyclic peptide antibiotics have been synthesized in vitro using computer-guided AI technologies to predict 3D drug-enzyme interactions and are currently undergoing clinical trials. Our ultimate goal is to combat XDR bacteria-associated deaths, which are predicted to escalate as we approach 2050.
- McArthur AG, Waglechner N, Nizam F, et al. The comprehensive antibiotic resistance database. Antimicrob Agents Chemother. 2013;57(7):3348-3357. doi: 10.1128/AAC.00419-13
- Woappi Y, Gabani P, Singh A, Singh OV. Antibiotrophs: The complexity of antibiotic-subsisting and antibiotic-resistant microorganisms. Crit Rev Microbiol. 2016;42(1):17-30. doi: 10.3109/1040841X.2013.875982
- Das B, Verma J, Kumar P, Ghosh A, Ramamurthy T. Antibiotic resistance in Vibrio cholerae: Understanding the ecology of resistance genes and mechanisms. Vaccine. 2020;38(Suppl 1):A83-A92. doi: 10.1016/j.vaccine.2019.06.031
- Chakraborty AK. High mode contamination of multi-drug resistant bacteria in Kolkata: Mechanism of gene activation and remedy by heterogeneous phyto-antibiotics. Indian J Biotechnol. 2015;14:149-159.
- Chakraborty AK. Ganga action plan, heterogeneous phyto-antibiotics and phage therapy are the best hope for India tackling superbug spread and control. Indian J Biol Sci. 2017;23:34-51.
- Moo CL, Yang SK, Yusoff K, et al. Mechanisms of Antimicrobial Resistance (AMR) and alternative approaches to overcome AMR. Curr Drug Discov Technol. 2020;17(4):430-447. doi: 10.2174/1570163816666190304122219
- Chakraborty AK. Current status and unusual mechanism of multiresistance in Mycobacterium tuberculosis. J Health Med Inform. 2019;10(1):328. doi: 10.4172/2157-7420.1000328
- Barbour A, Tagg J, Abou-Zied O, Philip K. New insights into the mode of action of the lantibiotic salivaricin B. Sci Rep. 2016;6:31749. doi: 10.1038/srep31749
- Barbour A, Wescombe PA, Simtj L. Evolution of lantibiotic salivaricins: New weapons to fight infectious diseases. Trends Microbiol. 2020;28(7):578-593. doi: 10.1016/j.tim.2020.03.001
- Miklasińska-Majdanik M. Mechanisms of resistance to macrolide antibiotics among Staphylococcus aureus. Antibiotics (Basel). 2021;10(11):1406. doi: 10.3390/antibiotics10111406
- Cowan MM. Plant products as antimicrobial agents. Clin Microbiol Rev. 1999;12:564-582. doi: 10.1128/CMR.12.4.564
- Ren Y, Yu J, Kinghorn AD. Development of anticancer agents from plant-derived sesquiterpene lactones. Curr Med Chem. 2016;23(23):2397-2420. doi: 10.2174/0929867323666160510123255
- Daglia M. Polyphenols as antimicrobial agents. Curr Opin Biotechnol. 2011;23:174-181. doi: 10.1016/j.copbio.2011.08.007
- Chakraborty AK. Multi-drug resistant bacteria from Kolkata Ganga River with heterogeneous MDR genes have four hallmarks of cancer cells but could be controlled by organic phyto-extracts. Biochem Biotechnol Res. 2017;5(1):11-23.
- Chakraborty AK, Saha S, Poria K, Samanta T, Gautam S, Mukhopadhyay J. A saponin-polybromophenol antibiotic (CU1) from Cassia fistula bark against multi-drug resistant bacteria targeting RNA polymerase. Curr Res Pharmacol Drug Discov. 2022;3:100090. doi: 10.1016/j.crphar.2022.100090
- Liu K, Huigens RW 3rd. Instructive advances in chemical microbiology inspired by nature’s diverse inventory of molecules. ACS Infect Dis. 2020;6(4):541-562. doi: 10.1021/acsinfecdis.9b00413
- Tan HM, Lall AC, Keppo J, Chen SL. Evaluation of a new antiresistic strategy to manage antibiotic resistance. J Glob Antimicrob Resist. 2023;33:368-375. doi: 10.1016/j.jgar.2023.03.006
- Maniatis T, Fritsch EF, Sambrook J. Molecular Cloning-A Laboratory Manual. Cold Spring Harbor, NY, USA: Cold Spring Harbor Laboratory Press; 1982.
- Koser SA. Correlation of Citrate utilization by members of the colon-aerogenes group with other differential characteristics and with habitat. J Bacterol. 1924;9:59-77. doi: 10.1128/jb.9.1.59-77.1924
- Thomson JJ. Cathode rays. Philos Mag. 1897;44(269):293-316. doi: 10.1080/14786449708621070
- Aston FW. LXXIV. A positive ray spectrograph. In: The London, Edinburgh, and Dublin Philosophical Magazine and Journal of Science. Vol. 38. United States: Creative Media Partners, LLC; 1919. p. 707-714. doi: 10.1080/14786441208636004
- Song Y, Cong Y, Wang B, Zhang N. Applications of Fourier transform infrared spectroscopy to pharmaceutical preparations. Expert Opin Drug Deliv. 2020;17(4):551-571. doi: 10.1080/17425247.2020.1737671
- Willams AD, Rousham E, Neal AL, et al. Impact of contrasting poultry exposures on human, poultry, and wastewater antibiotic resistomes in Bangladesh. Microbiol Spectr. 2023;11:e01763-23. doi: 10.1128/spectrum.01763-23
- Carvalho I, Chenouf NS, Carvalho JA, et al. Multidrug-resistant Klebsiella pneumoniae harboring extended spectrum β-lactamase encoding genes isolated from human septicemias. PLoS One. 2021;16(5):e0250525. doi: 10.1371/journal.pone.0250525
- Guclu AU, Gozen AG. Genetic diversity of OXA-like genes in multidrug-resistant Acinetobacter baumannii strains from ICUs. Clin Lab. 2020;66(10):20215-2019. doi: 10.7754/Clin.Lab.2020.200135
- Hotchkiss RD, Dubos RJ. Fractionation of the bactericidal agent from cultures of a soil Bacillus. J Biol Chem. 1940;132:791-792.
- Ganz T. Defensins: Antimicrobial peptides of innate immunity. Nat Rev Immunol. 2003;3:710-720. doi: 10.1038/nri1180
- Imran M, Abida, Alotaibi NM, et al. Computer-assisted discovery of safe and effective DprE1/aaRSs inhibitors against TB utilizing drug repurposing approach. J Infect Public Health. 2023;16(4):554-572. doi: 10.1016/j.jiph.2023.02.005
- Taira J, Nagano T, Kitamura M, Yamaguchi M, Sakamoto H, Aoki S. Structural modification of a novel inhibitor for mycobacterium enoyl-acyl carrier protein reductase assisted by in silico structure-based drug screening. Int J Mycobacteriol. 2020;9(1):12-17. doi: 10.4103/ijmy.ijmy_184_19
- Wayah SB, Philip K. Purification, characterization, mode of action, and enhanced production of salivaricin MMAYE1, a novel bacteriocin from Lactobacillus salivarius SPW1 of human gut origin. Electron J Biotechnol. 2018;35:39-47. doi: 10.1016/j.ejbt.2018.08.003
- Foreman KJ, Marquez N, Dolgert A, et al. Forecasting life expectancy, years of life lost, and all-cause and cause-specific mortality for 250 causes of death: Reference and alternative scenarios for 2016-40 for 195 countries and territories. Lancet. 2018;392(10159):2052-2090. doi: 10.1016/S0140-6736(18)31694-5
- Allel K, Day L, Hamilton A, et al. Global antimicrobial-resistance drivers: An ecological country-level study at the human-animal interface. Lancet Planet Health. 2023;7(4):e291-e303. doi: 10.1016/S2542-5196(23)00026-8
- Tanimura T, Jaramillo E, Weil D, Raviglione M, Lönnroth K. Financial burden for tuberculosis patients in low-and middle-income countries: A systematic review. Eur Respir J. 2014;43(6):1763-1775. doi: 10.1183/09031936.00193413
- Phan J, Nair D, Jain S, et al. Alterations in gut microbiome composition and function in irritable bowel syndrome and increased probiotic abundance with daily supplementation. mSystems. 2021;6(6):e01215-21. doi: 10.1128/mSystems.01215-21
- De Gaetano GV, Lentini G, Famà A, Coppolino F, Beninati C. Antimicrobial resistance: Two-component regulatory systems and multidrug efflux pumps. Antibiotics (Basel). 2023;12(6):965. doi: 10.3390/antibiotics12060965
- Alock BP, Raphenya AR, Lau TTY, et al. CARD 2020: Antibiotic resistome surveillance with the comprehensive antibiotic resistance database. Nucleic Acids Res. 2020;48:D517-D525. doi: 10.1093/nar/gkz935
- Barbour A, Smith L, Oveisi M, et al. Discovery of phosphorylated lantibiotics with proimmune activity that regulate the oral microbiome. Proc Natl Acad Sci U S A. 2023;120(22):e2219392120. doi: 10.1073/pnas.2219392120
- Pei ZF, Zhu L, Sarksian R, van der Donk WA, Nair SK. Class V Lanthipeptide cyclase directs the biosynthesis of a stapled peptide natural product. J Am Chem Soc. 2022;144(38):17549-17557. doi: 10.1021/jacs.2c06808
- Tovillas P, Navo CD, Oroz P, et al. Synthesis of β2,2-amino acids by stereoselective alkylation of isoserine derivatives followed by nucleophilic ring opening of quaternary sulfamidates. J Org Chem. 2022;87(13):8730-8743. doi: 10.1021/acs.joc.2c01034
- Bothwell IR, Caetano T, Sarksian R, Mendo S, van der Donk WA. Structural analysis of class I Lanthipeptides from Pedobacter lusitanus NL19 reveals an unusual ring pattern. ACS Chem Biol. 2021;16(6):1019-1029. doi: 10.1021/acschembio.1c00106
- Joaquin D, Lee MA, Kastner DW, et al. Impact of dehydroamino acids on the structure and stability of incipient 310-helical peptides. J Org Chem. 2020;85(3):1601-1613. doi: 10.1021/acs.joc.9b02747
- De Luca S, Digilio G, Verdoliva V, Tovillas P, Jiménez-Osés G, Peregrina JM. Lanthionine peptides by S-alkylation with substituted cyclic sulfamidates promoted by activated molecular sieves: Effects of the sulfamidate structure on the yield. J Org Chem. 2019;84(22):14957-14964. doi: 10.1021/acs.joc.9b02306
- Dickman R, Mitchell SA, Figueiredo AM, Hansen DF, Tabor AB. Molecular recognition of lipid II by Lantibiotics: Synthesis and conformational studies of analogues of nisin and mutacin rings A and B. J Org Chem. 2019;84(18):11493-11512. doi: 10.1021/acs.joc.9b01253
- Chen H, Zhang Y, Li QQ, Zhao YF, Chen YX, Li YM. De novo design to synthesize lanthipeptides involving cascade cysteine reactions: SapB Synthesis as an example. J Org Chem. 2018;83(14):7528-7533. doi: 10.1021/acs.joc.8b00259
- Ma C, Peng Y, Li H, Chen W. Organ-on-a-Chip: A new paradigm for drug development. Trends Pharmacol Sci. 2021;42(2):119-133. doi: 10.1016/j.tips.2020.11.009
- Najmi A, Javed SA, Al Bratty M, Alhazmi HA. Modern approaches in the discovery and development of plant-based natural products and their analogues as potential therapeutic agents. Molecules. 2022;27(2):349. doi: 10.3390/molecules27020349
- Sivadas N, Kaul G, Akhir A, et al. Naturally derived malabaricone B as a promising bactericidal candidate targeting multidrug-resistant Staphylococcus aureus also possess synergistic interactions with clinical antibiotics. Antibiotics (Basel). 2023;12(10):1483. doi: 10.3390/antibiotics12101483
- Torres MT, de la Fuente-Nunez C. Toward computer-made artificial antibiotics. Curr Opin Microbiol. 2019;51:30-38. doi: 10.1016/j.mib.2019.03.004
- Torres MDT, Cao J, Franco OL, Lu TK, de la Fuente- Nunez C. Synthetic biology and computer-based frameworks for antimicrobial peptide discovery. ACS Nano. 2021;15(2):2143-2164. doi: 10.1021/acsnano.0c09509
- Aronica PGA, Reid LM, Desai N, et al. Computational methods and tools in antimicrobial peptide research. J Chem Inf Model. 2021;61(7):3172-3196. doi: 10.1021/acs.jcim.1c00175
- Gray DA, Wenzel M. Multitarget approaches against multiresistant superbugs. ACS Infect Dis. 2020;6(6):1346-1365. doi: 10.1021/acsinfecdis.0c00001
- Fields FR, Lee SW, McConnell MJ. Using bacterial genomes and essential genes for the development of new antibiotics. Biochem Pharmacol. 2017;134:74-86. doi: 10.1016/j.bcp.2016.12.002
- Rana R, Awasthi R, Sharma B, Kulkarni GT. Nanoantibiotic formulations to combat antibiotic resistance - old wine in a new bottle. Recent Pat Drug Deliv Formul. 2019;13(3):174-183. doi: 10.2174/1872211313666190911124626
- Munir MU, Ahmed A, Usman M, Salman S. Recent advances in nanotechnology-aided materials in combating microbial resistance and functioning as antibiotics substitutes. Int J Nanomedicine. 2020;15:7329-7358. doi: 10.2147/IJN.S265934
- Manrique PD, López CA, Gnanakaran S, Rybenkov VV, Zgurskaya HI. New understanding of multidrug efflux and permeation in antibiotic resistance, persistence, and heteroresistance. Ann N Y Acad Sci. 2023;1519(1):46-62. doi: 10.1111/nyas.14921
- Mulat M, Pandita A, Khan F. Medicinal plant compounds for combating the multi-drug resistant pathogenic bacteria: A review. Curr Pharm Biotechnol. 2019;20(3):183-196. doi: 10.2174/1872210513666190308133429
- Tarín-Pelló A, Suay-García B, Pérez-Gracia MT. Antibiotic resistant bacteria: Current situation and treatment options to accelerate the development of a new antimicrobial arsenal. Expert Rev Anti Infect Ther. 2022;20(8):1095-1108. doi: 10.1080/14787210.2022.2078308
- Lv S, Wang Y, Jiang K, et al. Genetic engineering and biosynthesis technology: Keys to unlocking the chains of phage therapy. Viruses. 2023;15(8):1736. doi: 10.3390/v15081736
- Zeituni EM, Raterman EL. NIAID’s comprehensive support mechanisms for antibiotic development. ACS Infect Dis. 2020;6(6):1299-1301. doi: 10.1021/acsinfecdis.0c00099
- Lluka T, Stokes JM. Antibiotic discovery in the artificial intelligence era. Ann N Y Acad Sci. 2023;1519(1):74-93. doi: 10.1111/nyas.14930
- Xavier BB, Das AJ, Cochrane G, et al. Consolidating and exploring antibiotic resistance gene data resources. J Clin Microbiol. 2016;54(4):851-859. doi: 10.1128/JCM.02717-15