Targeting glioblastoma invasion and therapy resistance: Emerging trends and molecular pathways
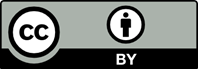
Glioblastoma (GBM) is the most common and aggressive primary central nervous system malignancy. Significant resistance to therapeutic intervention is a core feature of GBM that drives tumor recurrence and underlies the remarkably poor clinical outcomes associated with this disease. This review explores the therapeutic strategies and molecular pathways involved in GBM. Therapy resistance in GBM depends on multiple interconnected macrostructural and biomolecular mechanisms, including aggressive and diffuse invasion, tumor microtube network formation, stem-like cell enrichment, selective neurovascular permeability, an immunosuppressive microenvironment, and a high degree of inter- and intra-tumoral heterogeneity. Collectively, these pathobiological features insulate specific tumor compartments and maintain GBM viability despite significant treatment-induced cellular stress. While there is enthusiasm for addressing GBM therapeutic resistance, the scale of the challenge remains immense. The identified resistance mechanisms extensively interact and can compensate for single-target assaults. Emerging overlaps between neuro-oncology and developmental neurobiology additionally suggest that GBM may exploit therapeutic resistance mechanisms yet to be identified, functioning beyond the current scientific understanding. Thus, the scope and diversity of this problem demand a comprehensive therapeutic approach capable of targeting multiple interacting mechanisms of therapeutic resistance.
- Siegel RL, Miller KD, Jemal A. Cancer statistics, 2019. CA Cancer J Clin. 2019;69(1):7-34. doi: 10.3322/caac.21551
- Ostrom QT, Cioffi G, Waite K, Kruchko C, Barnholtz-Sloan JS. CBTRUS statistical report: Primary brain and other central nervous system tumors diagnosed in the United States in 2014-2018. Neuro Oncol. 2021;23(12 Suppl 2):iii1-iii105. doi: 10.1093/neuonc/noab200
- Hsu S, Quattrone M, Ostrom Q, Ryken TC, Sloan AE, Barnholtz-Sloan JS. Incidence patterns for primary malignant spinal cord gliomas: A surveillance, epidemiology, and end results study. J Neurosurg Spine. 2011;14(6):742-747. doi: 10.3171/2011.1.SPINE10351
- SEER*Explorer: An Interactive Website for SEER Cancer Statistics. Surveillance Research Program, National Cancer Institute. Data Source(s): SEER Incidence Data, November 2023 Submission (1975-2021), SEER 22 Registries; 2024. Available from: https://seer.cancer.gov/statistics-network/ explorer Last accessed on 2025 Apr 10].
- Stupp R, Mason WP, van den Bent MJ, et al. Radiotherapy plus concomitant and adjuvant temozolomide for glioblastoma. N Engl J Med. 2005;352(10):987-996. doi: 10.1056/NEJMoa043330
- Bauman GS, Gaspar LE, Fisher BJ, Halperin EC, Macdonald DR, Cairncross JG. A prospective study of short-course radiotherapy in poor prognosis glioblastoma multiforme. Int J Radiat Oncol Biol Phys. 1994;29(4):835-839. doi: 10.1016/0360-3016(94)90573-8
- Whittle IR, Denholm SW, Gregor A. Management of patients aged over 60 years with supratentorial glioma: Lessons from an audit. Surg Neurol. 1991;36(2):106-111. doi: 10.1016/0090-3019(91)90227-z
- Parsons DW, Jones S, Zhang X, et al. An integrated genomic analysis of human glioblastoma multiforme. Science. 2008;321(5897):1807-1812. doi: 10.1126/science.1164382
- Cancer Genome Atlas Research Network. Comprehensive genomic characterization defines human glioblastoma genes and core pathways. Nature. 2008;455(7216):1061-1068. doi: 10.1038/nature07385
- Neftel C, Laffy J, Filbin MG, et al. An integrative model of cellular states, plasticity, and genetics for glioblastoma. Cell. 2019;178(4):835-849.e21. doi: 10.1016/j.cell.2019.06.024
- U.S. Department of Health and Human Services - Rare Cancers. U.S. Department of Health and Human Services. 2022. Available from: https://rarediseases.info.nih.gov/diseases/ diseases-by-category/1/rare-cancers [Last accessed on 2022 Jan 30].
- Ohgaki H, Dessen P, Jourde B, et al. Genetic pathways to glioblastoma: A population-based study. Cancer Res. 2004;64(19):6892-6899. doi: 10.1158/0008-5472.Can-04-1337
- Ostrom QT, Egan KM, Nabors LB, et al. Glioma risk associated with extent of estimated European genetic ancestry in African Americans and Hispanics. Int J Cancer. 2020;146(3):739-748. doi: 10.1002/ijc.32318
- Porter AB, Lachance DH, Johnson DR. Socioeconomic status and glioblastoma risk: A population-based analysis. Cancer Causes Control. 2015;26(2):179-185. doi: 10.1007/s10552-014-0496-x
- Braganza MZ, Kitahara CM, Berrington de González A, Inskip PD, Johnson KJ, Rajaraman P. Ionizing radiation and the risk of brain and central nervous system tumors: A systematic review. Neuro Oncol. 2012;14(11):1316-1324. doi: 10.1093/neuonc/nos208
- Ostrom QT, Adel Fahmideh M, Cote DJ, et al. Risk factors for childhood and adult primary brain tumors. Neuro Oncol. 2019;21(11):1357-1375. doi: 10.1093/neuonc/noz123
- Kinnersley B, Mitchell JS, Gousias K, et al. Quantifying the heritability of glioma using genome-wide complex trait analysis. Sci Rep. 2015;5:17267. doi: 10.1038/srep17267
- Wrensch M, Lee M, Miike R, et al. Familial and personal medical history of cancer and nervous system conditions among adults with glioma and controls. Am J Epidemiol. 1997;145(7):581-593. doi: 10.1093/oxfordjournals.aje.a009154
- Kumar VA, Abbas AK, Aster JC. Neoplasia. In: Robbins and Cotran Pathological Basis of Disease. 9th ed., Ch. 7. Amsterdam, Netherlands: Elsevier Health Sciences; 2014. p. 265-340.
- Pedersen PH, Rucklidge GJ, Mørk SJ, et al. Leptomeningeal tissue: A barrier against brain tumor cell invasion. J Natl Cancer Inst. 1994;86(21):1593-1599. doi: 10.1093/jnci/86.21.1593
- Louveau A, Herz J, Alme MN, et al. CNS lymphatic drainage and neuroinflammation are regulated by meningeal lymphatic vasculature. Nat Neurosci. 2018;21(10):1380-1391. doi: 10.1038/s41593-018-0227-9
- Houston SC, Crocker IR, Brat DJ, Olson JJ. Extraneural metastatic glioblastoma after interstitial brachytherapy. Int J Radiat Oncol Biol Phys. 2000;48(3):831-836. doi: 10.1016/s0360-3016(00)00662-3
- WHO International Agency for Research on Cancer. World Health Organization Classification of Tumours of the Central Nervous System. 5th ed. Lyon, France: WHO International Agency for Research on Cancer; 2021.
- Wesseling P, Kros JM, Jeuken JWM. The pathological diagnosis of diffuse gliomas: Towards a smart synthesis of microscopic and molecular information in a multidisciplinary context. Diagn Histopathol. 2011;17(11):486-494. doi: 10.1016/j.mpdhp.2011.08.005
- Yachnis, AT, Rivera-Zengotita, ML. Diffuse gliomas: Astrocytic. In Neuropathology. United States: Elsevier Sanders; 2014. p. 72-83.
- Kumar V, Abbas, AK, Aster JC. Robbins and Cotran Pathologic Basis of Disease. 10th ed. Amsterdam, Netherlands: Elsevier- OHCE; 2020.
- WHO International Agency for Research on Cancer. World Health Organization Classification of Tumours of the Central Nervous System. 4th ed. Lyon, France: WHO International Agency for Research on Cancer; 2016.
- Horbinski C, Ligon KL, Brastianos P, et al. The medical necessity of advanced molecular testing in the diagnosis and treatment of brain tumor patients. Neuro Oncol. 2019;21(12):1498-1508. doi: 10.1093/neuonc/noz119
- Louis DN, Aldape K, Brat DJ, et al. Announcing cIMPACT-NOW: The consortium to inform molecular and practical approaches to CNS tumor taxonomy. Acta Neuropathol. 2017;133(1):1-3. doi: 10.1007/s00401-016-1646-x
- Weller M, van den Bent M, Preusser M, et al. EANO guidelines on the diagnosis and treatment of diffuse gliomas of adulthood. Nat Rev Clin Oncol. 2021;18(3):170-186. doi: 10.1038/s41571-020-00447-z
- Ohgaki H, Kleihues P. The definition of primary and secondary glioblastoma. Clin Cancer Res. 2013;19(4):764-772. doi: 10.1158/1078-0432.Ccr-12-3002
- Guo C, Pirozzi CJ, Lopez GY, Yan H. Isocitrate dehydrogenase mutations in gliomas: Mechanisms, biomarkers and therapeutic target. Curr Opin Neurol. 2011;24(6):648-652. doi: 10.1097/WCO.0b013e32834cd415
- Hegi ME, Diserens AC, Gorlia T, et al. MGMT gene silencing and benefit from temozolomide in glioblastoma. N Engl J Med. 2005;352(10):997-1003. doi: 10.1056/NEJMoa043331
- Alexander BM, Cloughesy TF. Adult glioblastoma. J Clin Oncol. 2017;35(21):2402-2409. doi: 10.1200/jco.2017.73.0119
- Chaichana KL, Parker SL, Olivi A, Quiñones-Hinojosa A. Long-term seizure outcomes in adult patients undergoing primary resection of malignant brain astrocytomas. Clinical article. J Neurosurg. 2009;111(2):282-292. doi: 10.3171/2009.2.JNS081132
- Bohman LE, Swanson KR, Moore JL, et al. Magnetic resonance imaging characteristics of glioblastoma multiforme: Implications for understanding glioma ontogeny. Neurosurgery. 2010;67(5):1319-1328. doi: 10.1227/NEU.0b013e3181f556ab
- Brown TJ, Brennan MC, Li M, et al. Association of the extent of resection with survival in glioblastoma: A systematic review and meta-analysis. JAMA Oncol. 2016;2(11):1460-1469. doi: 10.1001/jamaoncol.2016.1373
- Grabowski MM, Recinos PF, Nowacki AS, et al. Residual tumor volume versus extent of resection: Predictors of survival after surgery for glioblastoma. J Neurosurg. 2014;121(5):1115-1123. doi: 10.3171/2014.7.Jns132449
- Ciric I, Ammirati M, Vick N, Mikhael M. Supratentorial gliomas: Surgical considerations and immediate postoperative results. Gross total resection versus partial resection. Neurosurgery. 1987;21(1):21-26. doi: 10.1227/00006123-198707000-00005
- Simpson JR, Horton J, Scott C, et al. Influence of location and extent of surgical resection on survival of patients with glioblastoma multiforme: Results of three consecutive Radiation Therapy Oncology Group (RTOG) clinical trials. Int J Radiat Oncol Biol Phys. 1993;26(2):239-244. doi: 10.1016/0360-3016(93)90203-8
- Chambless LB, Kistka HM, Parker SL, Hassam-Malani L, McGirt MJ, Thompson RC. The relative value of postoperative versus preoperative Karnofsky Performance Scale scores as a predictor of survival after surgical resection of glioblastoma multiforme. J Neurooncol. 2015;121(2):359-364. doi: 10.1007/s11060-014-1640-x
- Newlands ES, Stevens MF, Wedge SR, Wheelhouse RT, Brock C. Temozolomide: A review of its discovery, chemical properties, pre-clinical development and clinical trials. Cancer Treat Rev. 1997;23(1):35-61. doi: 10.1016/s0305-7372(97)90019-0
- Stupp R, Hegi ME, Mason WP, et al. Effects of radiotherapy with concomitant and adjuvant temozolomide versus radiotherapy alone on survival in glioblastoma in a randomised phase III study: 5-year analysis of the EORTC-NCIC trial. Lancet Oncol. 2009;10(5):459-466. doi: 10.1016/s1470-2045(09)70025-7
- Giladi M, Schneiderman RS, Voloshin T, et al. Mitotic spindle disruption by alternating electric fields leads to improper chromosome segregation and mitotic catastrophe in cancer cells. Sci Rep. 2015;5:18046. doi: 10.1038/srep18046
- Stupp R, Taillibert S, Kanner A, et al. Effect of tumor-treating fields plus maintenance temozolomide vs maintenance temozolomide alone on survival in patients with glioblastoma: A randomized clinical trial. JAMA. 2017;318(23):2306-2316. doi: 10.1001/jama.2017.18718
- Taphoorn MJB, Dirven L, Kanner AA, et al. Influence of treatment with tumor-treating fields on health-related quality of life of patients with newly diagnosed glioblastoma: A secondary analysis of a randomized clinical Trial. JAMA Oncol. 2018;4(4):495-504. doi: 10.1001/jamaoncol.2017.5082
- Konishi Y, Muragaki Y, Iseki H, Mitsuhashi N, Okada Y. Patterns of intracranial glioblastoma recurrence after aggressive surgical resection and adjuvant management: Retrospective analysis of 43 cases. Neurol Med Chir (Tokyo). 2012;52(8):577-586. doi: 10.2176/nmc.52.577
- Rapp M, Baernreuther J, Turowski B, Steiger HJ, Sabel M, Kamp MA. Recurrence pattern analysis of primary glioblastoma. World Neurosurg. 2017;103:733-740. doi: 10.1016/j.wneu.2017.04.053
- Lombardi G, Pambuku A, Bellu L, et al. Effectiveness of antiangiogenic drugs in glioblastoma patients: A systematic review and meta-analysis of randomized clinical trials. Crit Rev Oncol Hematol. 2017;111:94-102. doi: 10.1016/j.critrevonc.2017.01.018
- Keunen O, Johansson M, Oudin A, et al. Anti-VEGF treatment reduces blood supply and increases tumor cell invasion in glioblastoma. Proc Natl Acad Sci U S A. 2011;108(9):3749-3754. doi: 10.1073/pnas.1014480108
- Sizoo EM, Braam L, Postma TJ, et al. Symptoms and problems in the end-of-life phase of high-grade glioma patients. Neuro Oncol. 2010;12(11):1162-1166. doi: 10.1093/neuonc/nop045
- Thier K, Calabek B, Tinchon A, Grisold W, Oberndorfer S. The last 10 days of patients with glioblastoma: Assessment of clinical signs and symptoms as well as treatment. Am J Hosp Palliat Care. 2016;33(10):985-988. doi: 10.1177/1049909115609295
- Sizoo EM, Koekkoek JA, Postma TJ, et al. Seizures in patients with high-grade glioma: A serious challenge in the end-of-life phase. BMJ Support Palliat Care. 2014;4(1):77-80. doi: 10.1136/bmjspcare-2013-000456
- Sizoo EM, Pasman HR, Dirven L, et al. The end-of-life phase of high-grade glioma patients: A systematic review. Support Care Cancer. 2014;22(3):847-857. doi: 10.1007/s00520-013-2088-9
- Edelstein K, Coate L, Massey C, Jewitt NC, Mason WP, Devins GM. Illness intrusiveness and subjective well-being in patients with glioblastoma. J Neurooncol. 2016;126(1):127-135. doi: 10.1007/s11060-015-1943-6
- Bergo E, Lombardi G, Guglieri I, Capovilla E, Pambuku A, Zagone V. Neurocognitive functions and health-related quality of life in glioblastoma patients: A concise review of the literature. Eur J Cancer Care (Engl). 2019;28(1):e12410. doi: 10.1111/ecc.12410
- Silbergeld DL, Rostomily RC, Alvord EC Jr. The cause of death in patients with glioblastoma is multifactorial: Clinical factors and autopsy findings in 117 cases of supratentorial glioblastoma in adults. J Neurooncol. 1991;10(2):179-185. doi: 10.1007/BF00146880
- Bausewein C, Hau P, Borasio GD, Voltz R. How do patients with primary brain tumours die? Palliat Med. 2003;17(6):558-559. doi: 10.1177/026921630301700615
- Galkin VE, VanLoock MS, Orlova A, Egelman EH. A new internal mode in F-actin helps explain the remarkable evolutionary conservation of actin’s sequence and structure. Curr Biol. 2002;12(7):570-575. doi: 10.1016/s0960-9822(02)00742-x
- Holmes KC, Popp D, Gebhard W, Kabsch W. Atomic model of the actin filament. Nature. 1990;347(6288):44-49. doi: 10.1038/347044a0
- Svitkina TM. The cytoskeletal organization of epithelial cells in culture. [Organizatsiia tsitoskeleta épitelial’nykh kletok v kul’ture]. Tsitologiia. 1989;31(12):1435-1440.
- Erickson HP. Microtubule surface lattice and subunit structure and observations on reassembly. J Cell Biol. 1974;60(1):153-167. doi: 10.1083/jcb.60.1.153
- Goodson HV, Jonasson EM. Microtubules and microtubule-associated proteins. Cold Spring Harb Perspect Biol. 2018;10(6):a022608. doi: 10.1101/cshperspect.a022608
- Hayden JH, Bowser SS, Rieder CL. Kinetochores capture astral microtubules during chromosome attachment to the mitotic spindle: Direct visualization in live newt lung cells. J Cell Biol. 1990;111(3):1039-1045. doi: 10.1083/jcb.111.3.1039
- Barlan K, Gelfand VI. Microtubule-based transport and the distribution, tethering, and organization of organelles. Cold Spring Harbor Perspect Biol. 2017;9(5):a025817. doi: 10.1101/cshperspect.a025817
- Etienne-Manneville S. Microtubules in cell migration. Annu Rev Cell Dev Biol. 2013;29:471-499. doi: 10.1146/annurev-cellbio-101011-155711
- Sharma P, Alsharif S, Fallatah A, Chung BM. Intermediate filaments as effectors of cancer development and metastasis: A focus on Keratins, vimentin, and nestin. Cells. 2019;8(5):497. doi: 10.3390/cells8050497
- Ishikawa H, Bischoff R, Holtzer H. Mitosis and intermediate-sized filaments in developing skeletal muscle. J Cell Biol. 1968;38(3):538-555. doi: 10.1083/jcb.38.3.538
- Herrmann H, Aebi U. Intermediate filaments: Structure and assembly. Cold Spring Harb Perspect Biol. 2016;8(11):a018242. doi: 10.1101/cshperspect.a018242
- Pruss RM, Mirsky R, Raff MC, Thorpe R, Dowding AJ, Anderton BH. All classes of intermediate filaments share a common antigenic determinant defined by a monoclonal antibody. Cell. 1981;27(3 Pt 2):419-428. doi: 10.1016/0092-8674(81)90383-4
- Xie R, Kessler T, Grosch J, et al. Tumor cell network integration in glioma represents a stemness feature. Neuro Oncol. 2021;23(5):757-769. doi: 10.1093/neuonc/noaa275
- Scherer HJ. Structural development in gliomas. Am J Cancer. 1938;34(3):333. doi: 10.1158/ajc.1938.333
- Brooks LJ, Clements MP, Burden JJ, et al. The white matter is a pro-differentiative niche for glioblastoma. Nat Commun. 2021;12(1):2184. doi: 10.1038/s41467-021-22225-w
- Diksin M, Smith SJ, Rahman R. The molecular and phenotypic basis of the glioma invasive perivascular niche. Int J Mol Sci. 2017;18(11):2184. doi: 10.3390/ijms18112342
- Tilling T, Engelbertz C, Decker S, Korte D, Hüwel S, Galla HJ. Expression and adhesive properties of basement membrane proteins in cerebral capillary endothelial cell cultures. Cell Tissue Res. 2002;310(1):19-29. doi: 10.1007/s00441-002-0604-1
- Yu Q, Xue Y, Liu J, Xi Z, Li Z, Liu Y. Fibronectin promotes the malignancy of glioma stem-like cells via modulation of cell adhesion, differentiation, proliferation and chemoresistance. Front Mol Neurosci. 2018;11:130. doi: 10.3389/fnmol.2018.00130
- Burnside ER, Bradbury EJ. Manipulating the extracellular matrix and its role in brain and spinal cord plasticity and repair. Neuropathol Appl Neurobiol. 2014;40(1):26-59. doi: 10.1111/nan.12114
- Thorne RG, Nicholson C. In vivo diffusion analysis with quantum dots and dextrans predicts the width of brain extracellular space. Proc Natl Acad Sci U S A. 2006;103(14):5567-5572. doi: 10.1073/pnas.0509425103
- Quesnel A, Karagiannis GS, Filippou PS. Extracellular proteolysis in glioblastoma progression and therapeutics. Biochim Biophys Acta Rev Cancer. 2020;1874(2):188428. doi: 10.1016/j.bbcan.2020.188428
- Gritsenko PG, Atlasy N, Dieteren CEJ, et al. p120-catenin-dependent collective brain infiltration by glioma cell networks. Nat Cell Biol. 2020;22(1):97-107. doi: 10.1038/s41556-019-0443-x
- Beadle C, Assanah MC, Monzo P, Vallee R, Rosenfeld SS, Canoll P. The role of myosin II in glioma invasion of the brain. Mol Biol Cell. 2008;19(8):3357-3368. doi: 10.1091/mbc.e08-03-0319
- Cuddapah VA, Robel S, Watkins S, Sontheimer H. A neurocentric perspective on glioma invasion. Nat Rev Neurosci. 2014;15(7):455-465. doi: 10.1038/nrn3765
- Zottel A, Jovcevska I, Samec N, Komel R. Cytoskeletal proteins as glioblastoma biomarkers and targets for therapy: A systematic review. Crit Rev Oncol Hematol. 2021;160:103283. doi: 10.1016/j.critrevonc.2021.103283
- Arden JD, Lavik KI, Rubinic KA, et al. Small-molecule agonists of mammalian Diaphanous-related (mDia) formins reveal an effective glioblastoma anti-invasion strategy. Mol Biol Cell. 2015;26(21):3704-3718. doi: 10.1091/mbc.E14-11-1502
- Yamana N, Arakawa Y, Nishino T, et al. The Rho-mDia1 pathway regulates cell polarity and focal adhesion turnover in migrating cells through mobilizing Apc and c-Src. Mol Cell Biol. 2006;26(18):6844-6858. doi: 10.1128/mcb.00283-06
- Li Z, Xu Y, Zhang C, Liu X, Jiang L, Chen F. Mammalian diaphanous-related formin 1 is required for motility and invadopodia formation in human U87 glioblastoma cells. Int J Mol Med. 2014;33(2):383-391. doi: 10.3892/ijmm.2013.1577
- Heuser VD, Kiviniemi A, Lehtinen L, et al. Multiple formin proteins participate in glioblastoma migration. BMC Cancer. 2020;20(1):710. doi: 10.1186/s12885-020-07211-7
- Higa N, Shinsato Y, Kamil M, et al. Formin-like 1 (FMNL1) is associated with glioblastoma multiforme mesenchymal subtype and independently predicts poor prognosis. Int J Mol Sci. 2019;20(24):6355. doi: 10.3390/ijms20246355
- Monzo P, Chong YK, Guetta-Terrier C, et al. Mechanical confinement triggers glioma linear migration dependent on formin FHOD3. Mol Biol Cell. 2016;27(8):1246-1261. doi: 10.1091/mbc.E15-08-0565
- Katsetos CD, Reginato MJ, Baas PW, et al. Emerging microtubule targets in glioma therapy. Semin Pediatr Neurol. 2015;22(1):49-72. doi: 10.1016/j.spen.2015.03.009
- Pettee KM, Becker KN, Alberts AS, Reinard KA, Schroeder JL, Eisenmann KM. Targeting the mdia formin-assembled cytoskeleton is an effective anti-invasion strategy in adult high-grade glioma patient-derived neurospheres. Cancers (Basel). 2019;11(3):392. doi: 10.3390/cancers11030392
- Horne EA, Diaz P, Cimino PJ, et al. A brain-penetrant microtubule-targeting agent that disrupts hallmarks of glioma tumorigenesis. Neurooncol Adv. 2021;3(1):vdaa165. doi: 10.1093/noajnl/vdaa165
- Al-Koussa H, Atat OE, Jaafar L, Tashjian H, El-Sibai M. The role of Rho GTPases in motility and invasion of glioblastoma cells. Anal Cell Pathol (Amst). 2020;2020:9274016. doi: 10.1155/2020/9274016
- Hirata E, Yukinaga H, Kamioka Y, et al. In vivo fluorescence resonance energy transfer imaging reveals differential activation of Rho-family GTPases in glioblastoma cell invasion. J Cell Sci. 2012;125(Pt 4):858-868. doi: 10.1242/jcs.089995
- Xu J, Simonelli F, Li X, et al. Molecular mechanisms of the blockage of glioblastoma motility. J Chem Inf Model. 2021;61(6):2967-2980. doi: 10.1021/acs.jcim.1c00279
- Okura H, Golbourn BJ, Shahzad U, et al. A role for activated Cdc42 in glioblastoma multiforme invasion. Oncotarget. 2016;7(35):56958-56975. doi: 10.18632/oncotarget.10925
- Xu J, Galvanetto N, Nie J, Yang Y, Torre V. Rac1 promotes cell motility by controlling cell mechanics in human glioblastoma. Cancers (Basel). 2020;12(6):1667. doi: 10.3390/cancers12061667
- Forget MA, Desrosiers RR, Del M, et al. The expression of rho proteins decreases with human brain tumor progression: Potential tumor markers. Clin Exp Metastasis. 2002;19(1):9-15. doi: 10.1023/a:1013884426692
- SenGupta S, Parent CA, Bear JE. The principles of directed cell migration. Nat Rev Mol Cell Biol. 2021;22(8):529-547. doi: 10.1038/s41580-021-00366-6
- Annabi B, Bouzeghrane M, Moumdjian R, Moghrabi A, Béliveau R. Probing the infiltrating character of brain tumors: Inhibition of RhoA/ROK-mediated CD44 cell surface shedding from glioma cells by the green tea catechin EGCg. J Neurochem. 2005;94(4):906-916. doi: 10.1111/j.1471-4159.2005.03256.x
- Ulrich TA, de Juan Pardo EM, Kumar S. The mechanical rigidity of the extracellular matrix regulates the structure, motility, and proliferation of glioma cells. Cancer Res. 2009;69(10):4167-4174. doi: 10.1158/0008-5472.Can-08-4859
- Chen L, Zhu M, Yu S, et al. Arg kinase mediates CXCL12/ CXCR4-induced invadopodia formation and invasion of glioma cells. Exp Cell Res. 2020;389(1):111893. doi: 10.1016/j.yexcr.2020.111893
- van Lith SA, Navis AC, Verrijp K, et al. Glutamate as chemotactic fuel for diffuse glioma cells: Are they glutamate suckers? Biochim Biophys Acta. 2014;1846(1):66-74. doi: 10.1016/j.bbcan.2014.04.004
- Qin EY, Cooper DD, Abbott KL, et al. Neural precursor-derived pleiotrophin mediates subventricular zone invasion by glioma. Cell. 2017;170(5):845-859.e19. doi: 10.1016/j.cell.2017.07.016
- Catacuzzeno L, Franciolini F. Role of KCa3.1 channels in Modulating Ca2+ oscillations during glioblastoma cell migration and invasion. Int J Mol Sci. 2018;19(10):2970. doi: 10.3390/ijms19102970
- Venkatesh HS, Morishita W, Geraghty AC, et al. Electrical and synaptic integration of glioma into neural circuits. Nature. 2019;573(7775):539-545. doi: 10.1038/s41586-019-1563-y
- Venkataramani V, Tanev DI, Strahle C, et al. Glutamatergic synaptic input to glioma cells drives brain tumour progression. Nature. 2019;573(7775):532-538. doi: 10.1038/s41586-019-1564-x
- Castrillon DH, Wasserman SA. Diaphanous is required for cytokinesis in Drosophila and shares domains of similarity with the products of the limb deformity gene. Development. 1994;120(12):3367-3377. doi: 10.1242/dev.120.12.3367
- Fabregat A, Sidiropoulos K, Viteri G, et al. Reactome diagram viewer: Data structures and strategies to boost performance. Bioinformatics. 2018;34(7):1208-1214. doi: 10.1093/bioinformatics/btx752
- Pimm ML, Henty-Ridilla JL. New twists in actin-microtubule interactions. Mol Biol Cell. 2021;32(3):211-217. doi: 10.1091/mbc.E19-09-0491
- Palazzo AF, Cook TA, Alberts AS, Gundersen GG. mDia mediates Rho-regulated formation and orientation of stable microtubules. Nat Cell Biol. 2001;3(8):723-729. doi: 10.1038/35087035
- Bartolini F, Moseley JB, Schmoranzer J, Cassimeris L, Goode BL, Gundersen GG. The formin mDia2 stabilizes microtubules independently of its actin nucleation activity. J Cell Biol. 2008;181(3):523-536. doi: 10.1083/jcb.200709029
- Ercan-Sencicek AG, Jambi S, Franjic D, et al. Homozygous loss of DIAPH1 is a novel cause of microcephaly in humans. Eur J Hum Genet. 2015;23(2):165-172. doi: 10.1038/ejhg.2014.82
- Arakawa Y, Bito H, Furuyashiki T, et al. Control of axon elongation via an SDF-1alpha/Rho/mDia pathway in cultured cerebellar granule neurons. J Cell Biol. 2003;161(2):381-391. doi: 10.1083/jcb.200210149
- Herzog D, Loetscher P, van Hengel J, et al. The small GTPase RhoA is required to maintain spinal cord neuroepithelium organization and the neural stem cell pool. J Neurosci. 2011;31(13):5120-5130. doi: 10.1523/jneurosci.4807-10.2011
- Shinohara R, Thumkeo D, Kamijo H, et al. A role for mDia, a Rho-regulated actin nucleator, in tangential migration of interneuron precursors. Nat Neurosci. 2012;15(3): 373-380, S1-S2. doi: 10.1038/nn.3020
- Ohshima Y, Kubo T, Koyama R, Ueno M, Nakagawa M, Yamashita T. Regulation of axonal elongation and pathfinding from the entorhinal cortex to the dentate gyrus in the hippocampus by the chemokine stromal cell-derived factor 1 alpha. J Neurosci. 2008;28(33):8344-8353. doi: 10.1523/jneurosci.1670-08.2008
- Hong EH, Kim JY, Kim JH, Lim DS, Kim M, Kim JY. BIG2-ARF1-RhoA-mDia1 signaling regulates dendritic golgi polarization in hippocampal neurons. Mol Neurobiol 2018;55(10):7701-7716. doi: 10.1007/s12035-018-0954-7
- Qu X, Yuan FN, Corona C, et al. Stabilization of dynamic microtubules by mDia1 drives Tau-dependent Aβ1-42 synaptotoxicity. J Cell Biol. 2017;216(10):3161-3178. doi: 10.1083/jcb.201701045
- Zhang C, Wang L, Chen J, et al. Knockdown of Diaph1 expression inhibits migration and decreases the expression of MMP2 and MMP9 in human glioma cells. Biomed Pharmacother. 2017;96:596-602. doi: 10.1016/j.biopha.2017.10.031
- Gargini R, Escoll M, García E, García-Escudero R, Wandosell F, Antón IM. WIP drives tumor progression through YAP/TAZ-dependent autonomous cell growth. Cell Rep. 2016;17(8):1962-1977. doi: 10.1016/j.celrep.2016.10.064
- Monzo P, Crestani M, Chong YK, et al. Adaptive mechanoproperties mediated by the formin FMN1 characterize glioblastoma fitness for invasion. Dev Cell. 2021;56(20):2841-2855.e8. doi: 10.1016/j.devcel.2021.09.007
- Dandy WE. Removal of right cerebral hemisphere for certain tumors with hemiplegia: Preliminary report. J Am Med Assoc. 1928;90(11):823-825. doi: 10.1001/jama.1928.02690380007003
- Sahm F, Capper D, Jeibmann A, et al. Addressing diffuse glioma as a systemic brain disease with single-cell analysis. Arch Neurol. 2012;69(4):523-526. doi: 10.1001/archneurol.2011.2910
- Watkins S, Robel S, Kimbrough IF, Robert SM, Ellis-Davies G, Sontheimer H. Disruption of astrocyte-vascular coupling and the blood-brain barrier by invading glioma cells. Nat Commun. 2014;5:4196. doi: 10.1038/ncomms5196
- Nagano N, Sasaki H, Aoyagi M, Hirakawa K. Invasion of experimental rat brain tumor: Early morphological changes following microinjection of C6 glioma cells. Acta Neuropathol. 1993;86(2):117-125. doi: 10.1007/bf00334878
- Berens ME, Giese A. “.those left behind.” Biology and oncology of invasive glioma cells. Neoplasia. 1999;1(3):208-219. doi: 10.1038/sj.neo.7900034
- Giese A, Loo MA, Tran N, Haskett D, Coons SW, Berens ME. Dichotomy of astrocytoma migration and proliferation. Int J Cancer. 1996;67(2):275-282. doi: 10.1002/(SICI)1097-0215(19960717)67:2<275:AID-IJC20>3.0.CO;2-9
- Giese A, Bjerkvig R, Berens ME, Westphal M. Cost of migration: Invasion of malignant gliomas and implications for treatment. J Clin Oncol. 2003;21(8):1624-1636. doi: 10.1200/jco.2003.05.063
- Ogawa D, Ansari K, Nowicki MO, Salinska E, Bronisz A, Godlewski J. MicroRNA-451 inhibits migration of glioblastoma while making it more susceptible to conventional therapy. Noncoding RNA. 2019;5(1):25. doi: 10.3390/ncrna5010025
- Ye ZC, Sontheimer H. Glioma cells release excitotoxic concentrations of glutamate. Cancer Res. 1999;59(17): 4383-4391.
- Buckingham SC, Campbell SL, Haas BR, et al. Glutamate release by primary brain tumors induces epileptic activity. Nat Med. 2011;17(10):1269-1274. doi: 10.1038/nm.2453
- Wild-Bode C, Weller M, Rimner A, Dichgans J, Wick W. Sublethal irradiation promotes migration and invasiveness of glioma cells: Implications for radiotherapy of human glioblastoma. Cancer Res. 2001;61(6):2744-2750.
- Dong Z, Zhou L, Han N, Zhang M, Lyu X. Wnt/beta-catenin pathway involvement in ionizing radiation-induced invasion of U87 glioblastoma cells. Strahlenther Onkol. 2015;191(8):672-680. doi: 10.1007/s00066-015-0858-7
- Phillips HS, Kharbanda S, Chen R, et al. Molecular subclasses of high-grade glioma predict prognosis, delineate a pattern of disease progression, and resemble stages in neurogenesis. Cancer Cell. 2006;9(3):157-173. doi: 10.1016/j.ccr.2006.02.019
- Osswald M, Jung E, Sahm F, et al. Brain tumour cells interconnect to a functional and resistant network. Nature. 2015;528(7580):93-98. doi: 10.1038/nature16071
- Jung E, Osswald M, Blaes J, et al. Tweety-homolog 1 drives brain colonization of gliomas. J Neurosci. 2017;37(29): 6837-6850. doi: 10.1523/jneurosci.3532-16.2017
- Pinto G, Saenz-de-Santa-Maria I, Chastagner P, et al. Patient-derived glioblastoma stem cells transfer mitochondria through tunneling nanotubes in tumor organoids. Biochem J. 2021;478(1):21-39. doi: 10.1042/BCJ20200710
- Jung E, Alfonso J, Osswald M, Monyer H, Wick W, Winkler F. Emerging intersections between neuroscience and glioma biology. Nat Neurosci. 2019;22:1951-1960. doi: 10.1038/s41593-019-0540-y
- Portela M, Venkataramani V, Fahey-Lozano N, et al. Glioblastoma cells vampirize WNT from neurons and trigger a JNK/MMP signaling loop that enhances glioblastoma progression and neurodegeneration. PLoS Biol. 2019;17(12):e3000545. doi: 10.1371/journal. pbio .3000545
- Joseph JV, Magaut CR, Storevik S, et al. TGF-β promotes microtube formation in glioblastoma through thrombospondin 1. Neuro Oncol. 2021;24:541-553. doi: 10.1093/neuonc/noab212
- Roehlecke C, Schmidt MHH. Tunneling nanotubes and tumor microtubes in cancer. Cancers (Basel). 2020;12(4):857. doi: 10.3390/cancers12040857
- Venkataramani V, Tanev DI, Kuner T, Wick W, Winkler F. Synaptic input to brain tumors: Clinical implications. Neuro Oncol. 2021;23(1):23-33. doi: 10.1093/neuonc/noaa158
- Dunn-Pirio AM, Woodring S, Lipp E, et al. Adjunctive perampanel for glioma-associated epilepsy. Epilepsy Behav Case Rep. 2018;10:114-117. doi: 10.1016/j.ebcr.2018.09.003
- Grossman SA, Ye X, Chamberlain M, et al. Talampanel with standard radiation and temozolomide in patients with newly diagnosed glioblastoma: A multicenter phase II trial. J Clin Oncol. 2009;27(25):4155-4161. doi: 10.1200/JCO.2008.21.6895
- Izumoto S, Miyauchi M, Tasaki T, et al. Seizures and tumor progression in glioma patients with uncontrollable epilepsy treated with perampanel. Anticancer Res. 2018;38(7): 4361-4366. doi: 10.21873/anticanres.12737
- Weil S, Osswald M, Solecki G, et al. Tumor microtubes convey resistance to surgical lesions and chemotherapy in gliomas. Neuro Oncol. 2017;19(10):1316-1326. doi: 10.1093/neuonc/nox070
- Tombal B, Denmeade SR, Gillis JM, Isaacs JT. A supramicromolar elevation of intracellular free calcium ([Ca(2+)](i)) is consistently required to induce the execution phase of apoptosis. Cell Death Differ. 2002;9(5):561-573. doi: 10.1038/sj.cdd.4400999
- Brightman MW, Reese TS. Junctions between intimately apposed cell membranes in the vertebrate brain. J Cell Biol. 1969;40(3):648-677. doi: 10.1083/jcb.40.3.648
- Sarkaria JN, Hu LS, Parney IF, et al. Is the blood-brain barrier really disrupted in all glioblastomas? A critical assessment of existing clinical data. Neuro Oncol. 2018;20(2):184-191. doi: 10.1093/neuonc/nox175
- Daneman R, Prat A. The blood-brain barrier. Cold Spring Harbor Perspect Biol. 2015;7(1):a020412. doi: 10.1101/cshperspect.a020412
- Löscher W, Potschka H. Blood-brain barrier active efflux transporters: ATP-binding cassette gene family. NeuroRx. 2005;2(1):86-98. doi: 10.1602/neurorx.2.1.86
- Armulik A, Genové G, Betsholtz C. Pericytes: Developmental, physiological, and pathological perspectives, problems, and promises. Dev Cell. 2011;21(2):193-215. doi: 10.1016/j.devcel.2011.07.001
- Hall CN, Reynell C, Gesslein B, et al. Capillary pericytes regulate cerebral blood flow in health and disease. Nature. 2014;508(7494):55-60. doi: 10.1038/nature13165
- Abbott NJ, Rönnbäck L, Hansson E. Astrocyte-endothelial interactions at the blood-brain barrier. Nat Rev Neurosci. 2006;7(1):41-53. doi: 10.1038/nrn1824
- Quail DF, Joyce JA. The microenvironmental landscape of brain tumors. Cancer Cell. 2017;31(3):326-341. doi: 10.1016/j.ccell.2017.02.009
- Minchinton AI, Tannock IF. Drug penetration in solid tumours. Nat Rev Cancer. 2006;6(8):583-592. doi: 10.1038/nrc1893
- Sampson JH, Gunn MD, Fecci PE, Ashley DM. Brain immunology and immunotherapy in brain tumours. Nat Rev Cancer. 2020;20(1):12-25. doi: 10.1038/s41568-019-0224-7
- Ousman SS, Kubes P. Immune surveillance in the central nervous system. Nat Neurosci. 2012;15(8):1096-1101. doi: 10.1038/nn.3161
- Vitkovic L, Maeda S, Sternberg E. Anti-inflammatory cytokines: Expression and action in the brain. Neuroimmunomodulation. 2001;9(6):295-312. doi: 10.1159/000059387
- Graeber MB, Scheithauer BW, Kreutzberg GW. Microglia in brain tumors. Glia. 2002;40(2):252-259. doi: 10.1002/glia.10147
- Hussain SF, Yang D, Suki D, Aldape K, Grimm E, Heimberger AB. The role of human glioma-infiltrating microglia/macrophages in mediating antitumor immune responses. Neuro Oncol. 2006;8(3):261-279. doi: 10.1215/15228517-2006-008
- Zhang I, Alizadeh D, Liang J, et al. Characterization of arginase expression in glioma-associated microglia and macrophages. PLoS One. 2016;11(12):e0165118. doi: 10.1371/journal.pone.0165118
- Wainwright DA, Balyasnikova IV, Chang AL, et al. IDO expression in brain tumors increases the recruitment of regulatory T cells and negatively impacts survival. Clin Cancer Res. 2012;18(22):6110-6121. doi: 10.1158/1078-0432.Ccr-12-2130
- Uyttenhove C, Pilotte L, Théate I, et al. Evidence for a tumoral immune resistance mechanism based on tryptophan degradation by indoleamine 2,3-dioxygenase. Nat Med. 2003;9(10):1269-1274. doi: 10.1038/nm934
- Woroniecka K, Fecci PE. T-cell exhaustion in glioblastoma. Oncotarget. 2018;9(82):35287-35288. doi: 10.18632/oncotarget.26228
- Chongsathidkiet P, Jackson C, Koyama S, et al. Sequestration of T cells in bone marrow in the setting of glioblastoma and other intracranial tumors. Nat Med. 2018;24(9):1459-1468. doi: 10.1038/s41591-018-0135-2
- Fecci PE, Mitchell DA, Whitesides JF, et al. Increased regulatory T-cell fraction amidst a diminished CD4 compartment explains cellular immune defects in patients with malignant glioma. Cancer Res. 2006;66(6):3294-3302. doi: 10.1158/0008-5472.Can-05-3773
- Patel AP, Tirosh I, Trombetta JJ, et al. Single-cell RNA-seq highlights intratumoral heterogeneity in primary glioblastoma. Science. 2014;344(6190):1396-1401. doi: 10.1126/science.1254257
- Wang Q, Hu B, Hu X, et al. Tumor evolution of glioma-intrinsic gene expression subtypes associates with immunological changes in the microenvironment. Cancer Cell. 2017;32(1):42-56.e6. doi: 10.1016/j.ccell.2017.06.003
- Wang J, Cazzato E, Ladewig E, et al. Clonal evolution of glioblastoma under therapy. Nat Genet. 2016;48(7):768-776. doi: 10.1038/ng.3590
- Touat M, Li YY, Boynton AN, et al. Mechanisms and therapeutic implications of hypermutation in gliomas. Nature. 2020;580(7804):517-523. doi: 10.1038/s41586-020-2209-9
- Kim H, Zheng S, Amini SS, et al. Whole-genome and multisector exome sequencing of primary and post-treatment glioblastoma reveals patterns of tumor evolution. Genome Res. 2015;25(3):316-327. doi: 10.1101/gr.180612.114
- Segerman A, Niklasson M, Haglund C, et al. Clonal variation in drug and radiation response among glioma-initiating cells is linked to proneural-mesenchymal transition. Cell Rep. 2016;17(11):2994-3009. doi: 10.1016/j.celrep.2016.11.056
- Chen J, Li Y, Yu TS, et al. A restricted cell population propagates glioblastoma growth after chemotherapy. Nature. 2012;488(7412):522-526. doi: 10.1038/nature11287
- Singh SK, Clarke ID, Terasaki M, et al. Identification of a cancer stem cell in human brain tumors. Cancer Res. 2003;63(18):5821-5828.
- Singh SK, Hawkins C, Clarke ID, et al. Identification of human brain tumour initiating cells. Nature. 2004;432(7015):396-401. doi: 10.1038/nature03128
- Bao S, Wu Q, McLendon RE, et al. Glioma stem cells promote radioresistance by preferential activation of the DNA damage response. Nature. 2006;444(7120):756-760. doi: 10.1038/nature05236
- Prager BC, Bhargava S, Mahadev V, Hubert CG, Rich JN. Glioblastoma stem cells: Driving resilience through chaos. Trends Cancer. 2020;6(3):223-235. doi: 10.1016/j.trecan.2020.01.009
- Lan X, Jörg DJ, Cavalli FMG, et al. Fate mapping of human glioblastoma reveals an invariant stem cell hierarchy. Nature. 2017;549(7671):227-232. doi: 10.1038/nature23666
- Piccirillo SGM, Colman S, Potter NE, et al. Genetic and functional diversity of propagating cells in glioblastoma. Stem Cell Reports. 2015;4(1):7-15. doi: 10.1016/j.stemcr.2014.11.003
- Lomonaco SL, Finniss S, Xiang C, et al. The induction of autophagy by gamma-radiation contributes to the radioresistance of glioma stem cells. Int J Cancer. 2009;125(3):717-722. doi: 10.1002/ijc.24402
- Bleau AM, Hambardzumyan D, Ozawa T, et al. PTEN/PI3K/ Akt pathway regulates the side population phenotype and ABCG2 activity in glioma tumor stem-like cells. Cell Stem Cell. 2009;4(3):226-235. doi: 10.1016/j.stem.2009.01.007
- Lee JH, Lee JE, Kahng JY, et al. Human glioblastoma arises from subventricular zone cells with low-level driver mutations. Nature. 2018;560(7717):243-247. doi: 10.1038/s41586-018-0389-3
- Barami K, Sloan AE, Rojiani A, Schell MJ, Staller A, Brem S. Relationship of gliomas to the ventricular walls. J Clin Neurosci. 2009;16(2):195-201. doi: 10.1016/j.jocn.2008.03.006
- Stiles CD, Rowitch DH. Glioma stem cells: A midterm exam. Neuron. 2008;58(6):832-846. doi: 10.1016/j.neuron.2008.05.031
- de Groot J, Sontheimer H. Glutamate and the biology of gliomas. Glia. 2011;59(8):1181-1189. doi: 10.1002/glia.21113
- Smits A, Jin Z, Elsir T, et al. GABA-A channel subunit expression in human glioma correlates with tumor histology and clinical outcome. PLoS One. 2012;7(5):e37041. doi: 10.1371/journal.pone.0037041
- Aldape K, Brindle KM, Chesler L, et al. Challenges to curing primary brain tumours. Nat Rev Clin Oncol. 2019;16(8): 509-520. doi: 10.1038/s41571-019-0177-5
- Monje M, Borniger JC, D’Silva NJ, et al. Roadmap for the emerging field of cancer neuroscience. Cell. 2020;181(2): 219-222. doi: 10.1016/j.cell.2020.03.034