Immune modulation and epigenetic therapies for enhanced outcome of treatment in triple-negative breast cancer
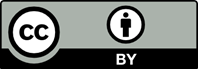
Triple-negative breast cancer (TNBC) is a subtype of breast cancer characterized by the absence of human epidermal growth factor receptor 2, estrogen receptor, and progesterone receptor expression. While traditional TNBC treatment primarily relies on systemic chemotherapy, epigenetic modification has an important role in immune evasion and tumor progression. Recent classifications of TNBC into “hot” and “cold” tumors, based on immune activity and mutation burden, have revealed that poor response to immune checkpoint inhibitors (ICIs) in these tumors is due to low tumor-infiltrating lymphocytes and the presence of immunosuppressive cells. Emerging treatments such as ICIs and poly(ADP-ribose) polymerase inhibitors offer promising alternatives. The tumor microenvironment (TME) shapes immune responses in TNBC, and a limited subset of patients has shown encouraging responses to ICIs in treating TNBC at both early and advanced stages. However, combination therapies face challenges, including medication interactions and side effects. Epigenetic modulators, such as histone deacetylase and DNA methyltransferase inhibitors, also show promise in reversing epigenetic changes by enhancing anti-tumor immunity in TNBC. The frequent expression of indoleamine 2,3-dioxygenase 1 (IDO1) in TNBC, which catalyzes the conversion of tryptophan to kynurenine, contributes to immune suppression in TNBC patients. High IDO1 levels impair the tumoricidal activity of natural killer cells and correlate with poor responses to anti-PD-L1 therapy, which can be improved using IDO1 inhibitors. Targeting myeloid-derived suppressor cells, regulatory T-cells, and tumor-associated macrophage, along with utilizing epigenetic mechanisms, show promise in modulating the immunosuppressive TME in TNBC. Combining these approaches with standard therapies, along with biomarker-guided patient selection, can enhance treatment efficacy. Clinical trials and preclinical models are essential for optimizing these strategies. This study emphasizes the importance of understanding the mechanisms of modulation, tumor-immune interactions, and the potential of epigenetic therapies in improving treatment outcomes in TNBC.
- Wolff AC, Hammond MEH, Hicks DG, Dowsett M, McShane LM, Allison KH. Recommendations for human epidermal growth factor receptor 2 testing in breast cancer. J Clin Oncol. 2013;31(31):3997-4013. doi: 10.5858/arpa.2018-0902-SA
- Yin L, Duan JJ, Bian XW, Yu SC. Triple-negative breast cancer molecular subtyping and treatment progress. Breast Cancer Res. 2020;22:61. doi: 10.1186/s13058-020-01296-5
- Lin NU, Vanderplas A, Hughes ME, Theriault RL, Edge SB, Wong YN. Clinicopathologic features, patterns of recurrence, and survival among women with triple-negative breast cancer in the National Comprehensive Cancer Network. Cancer. 2012;118(22):5463-5472. doi: 10.1002/cncr.27581
- Cortes J, Rugo HS, Cescon DW, Im SA, Yusof MM, Gallardo C. Pembrolizumab plus chemotherapy in advanced triple-negative breast cancer. N Engl J Med. 2022;387(3):217-226. doi: 10.1056/NEJMoa2202809
- Bardia A, Hurvitz SA, Tolaney SM, Loirat D, Punie K, Oliveira M. Sacituzumab govitecan in metastatic triple-negative breast cancer. N Engl J Med. 2021;384(16):1529-1541. doi: 10.1056/NEJMoa2028485
- Swann JB, Smyth MJ. Immune surveillance of tumors. J Clin Invest. 2007;117(4):1137-1146. doi: 10.1172/JCI31405
- Kim R. Cancer immunoediting: From immune surveillance to immune escape. Cancer Immunother. 2007;1:1-279. doi: 10.1111/j.1365-2567.2007.02587.x
- Ignacio J, Manughian-Peter A, Matthew P, O’Day S, Hoon DSB, Marzese DM. Mechanisms of immune evasion in triple-negative breast cancer patients. J Clin Oncol. 2018;36:1096. doi: 10.1200/JCO.2018.36.15_suppl.1096
- Llinàs-Arias P, Íñiguez-Muñoz S, McCann K, Voorwerk L, Orozco JIJ, Ensenyat-Mendez M. Epigenetic regulation of immunotherapy response in triple-negative breast cancer. Cancers (Basel). 2021;13(16):4139. doi: 10.3390/cancers13164139
- Yang J, Xu J, Wang W, Zhang B, Yu X, Shi S. Epigenetic regulation in the tumor microenvironment: Molecular mechanisms and therapeutic targets. Signal Transduct Target Ther. 2023;8:210. doi: 10.1038/s41392-023-01480-x
- Jung G, Hernández-Illán E, Moreira L, Balaguer F, Goel A. Epigenetics of colorectal cancer: Biomarker and therapeutic potential. Nat Rev Gastroenterol Hepatol. 2020;17:111-130. doi: 10.1038/s41575-019-0230-y
- Dawson MA, Kouzarides T. Cancer epigenetics: From mechanism to therapy. Cell. 2012;150:12-27. doi: 10.1016/j.cell.2012.06.013
- Schmid P, Cortes J, Pusztai L, McArthur H, Kümmel S, Bergh J. Pembrolizumab for early triple-negative breast cancer. N Engl J Med. 2020;382(9):810-821. doi: 10.1056/NEJMoa1910549
- Mittendorf EA, Zhang H, Barrios CH, Saji S, Jung KH, Hegg R. Neoadjuvant atezolizumab in combination with sequential nab-paclitaxel and anthracycline-based chemotherapy versus placebo and chemotherapy in patients with early-stage triple-negative breast cancer (IMpassion031): A randomized, double-blind, phase 3 trial. Lancet. 2020;396(10257):1090-1100. doi: 10.1016/S0140-6736(20)31953-X
- Zhang B, Wu Q, Li B, Wang D, Wang L, Zhou YL. M6A regulator-mediated methylation modification patterns and tumor microenvironment infiltration characterization in gastric cancer. Mol Cancer. 2020;19(1):53. doi: 10.1186/s12943-020-01170-0
- Duan Q, Zhang H, Zheng J, Zhang L. Turning cold into hot: Firing up the tumor microenvironment. Trends Cancer. 2020;6:605-618. doi: 10.1016/j.trecan.2020.03.002
- Zheng H, Siddharth S, Parida S, Wu X, Sharma D. Tumor microenvironment: Key players in triple-negative breast cancer immunomodulation. Cancers (Basel). 2021;13:3357. doi: 10.3390/cancers13163457
- Bai X, Ni J, Beretov J, Graham P, Li Y. Immunotherapy for triple-negative breast cancer: A molecular insight into the microenvironment, treatment, and resistance. J Natl Cancer Cent. 2021;1(3):75-87. doi: 10.1016/j.jncc.2021.06.001
- Wang H, Li S, Wang Q, et al. Tumor immunological phenotype signature-based high-throughput screening for the discovery of combination immunotherapy compounds. Sci Adv. 2021;7(4):eabd7851. doi: 10.1126/sciadv.abd7851
- Petrosyan V, Dobrolecki LE, LaPlante EL, et al. Immunologically “cold” triple negative breast cancers engraft at a higher rate in patient derived xenografts. NPJ Breast Cancer. 2022;8:104. doi: 10.1038/s41523-022-00476-0
- Perdiguero EG, Geissmann F. Identifying the infiltrators. Science. 2014;344:801-802. doi: 10.1126/science.1255117
- Huertas-Caro CA, Ramírez MA, Rey-Vargas L, et al. Tumor infiltrating lymphocytes (TILs) are a prognosis biomarker in Colombian patients with triple negative breast cancer. Sci Rep. 2023;13(1):21324. doi: 10.1038/s41598-023- 48300-4
- Dieci MV, Radosevic-Robin N, Fineberg S, et al. Update on tumor-infiltrating lymphocytes (TILs) in breast cancer, including recommendations to assess TILs in residual disease after neoadjuvant therapy and in carcinoma in situ: A report of the International Immuno-Oncology Biomarker Working Group on Breast Cancer. Semin Cancer Biol. 2018;52:16-25. doi: 10.1016/j.semcancer.2017.10.003
- Demaria S, Volm MD, Shapiro RL, et al. Development of tumor-infiltrating lymphocytes in breast cancer after neoadjuvant paclitaxel chemotherapy. Clin Cancer Res. 2001;7(10):3025-3032. doi: 10.1158/1078-0432.CCR-07-1004
- Liu S, Duan X, Xu L, et al. Optimal threshold for stromal tumor-infiltrating lymphocytes: Its predictive and prognostic value in HER2-positive breast cancer treated with trastuzumab-based neoadjuvant chemotherapy. Breast Cancer Res Treat. 2015;154(2):239-249. doi: 10.1007/s10549-015-3617-7
- West NR, Milne K, Truong PT, Macpherson N, Nelson BH, Watson PH. Tumor-infiltrating lymphocytes predict response to anthracycline-based chemotherapy in estrogen receptor-negative breast cancer. Breast Cancer Res. 2011;13(6):R125. doi: 10.1186/bcr3072
- Gao G, Wang Z, Qu X, Zhang Z. Prognostic value of tumor-infiltrating lymphocytes in patients with triple-negative breast cancer: A systematic review and meta-analysis. BMC Cancer. 2020;20(1):179. doi: 10.1186/s12885-020-6668-z
- Mao Y, Qu Q, Chen X, Huang O, Wu J, Shen K. The prognostic value of tumor-infiltrating lymphocytes in breast cancer: A systematic review and meta-analysis. PLoS One. 2016;11(4):e0152500. doi: 10.1371/journal.pone.0152500
- Wu Y, Yi M, Niu M, Mei Q, Wu K. Myeloid-derived suppressor cells: An emerging target for anticancer immunotherapy. Mol Cancer. 2022;21:184. doi: 10.1186/s12943-022-01657-y
- Ostrand-Rosenberg S, Sinha P. Myeloid-derived suppressor cells: Linking inflammation and cancer. J Immunol. 2009;182(8):4499-4506. doi: 10.4049/jimmunol.0802740
- Gomez S, Tabernacki T, Kobyra J, Roberts P, Chiappinelli KB. Combining epigenetic and immune therapy to overcome cancer resistance. Semin Cancer Biol. 2020;65:99-113. doi: 10.1016/j.semcancer.2019.05.008
- Bird L. MDSC metabolite stuns T cells. Nat Rev Immunol. 2020;20:352-353. doi: 10.1038/s41577-020-0336-z
- Lu Z, Zou J, Li S, et al. Epigenetic therapy inhibits metastases by disrupting premetastatic niches. Nature. 2020;579(7798):284-290. doi: 10.1038/s41586-020-2054-x
- Ge Y, Cheng D, Jia Q, Xiong H, Zhang J. Mechanisms underlying the role of myeloid-derived suppressor cells in clinical diseases: Good or bad. Immune Netw. 2021;21:e21 doi: 10.4110/in.2021.21.e21
- Xu Z, Ji J, Xu J, et al. MiR-30a increases MDSC differentiation and immunosuppressive function by targeting SOCS3 in mice with B-cell lymphoma. FEBS J. 2017;284(15):2410-2424. doi: 10.1111/febs.14133
- Kajihara N, Kobayashi T, Otsuka R, et al. Tumor-derived interleukin-34 creates an immunosuppressive and chemoresistant tumor microenvironment by modulating myeloid-derived suppressor cells in triple-negative breast cancer. Cancer Immunol Immunother. 2023;72(4):851-864. doi: 10.1007/s00262-022-03293-3
- Mehdizadeh R, Shariatpanahi SP, Goliaei B, Rüegg C. Targeting myeloid-derived suppressor cells in combination with tumor cell vaccination predicts anti-tumor immunity and breast cancer dormancy: An in silico experiment. Sci Rep. 2023;13(1):5875. doi: 10.1038/s41598-023-32554-z
- Hao Z, Li R, Wang Y, Li S, Hong Z, Han Z. Landscape of myeloid-derived suppressor cell in tumor immunotherapy. Biomark Res. 2021;9(1):77. doi: 10.1186/s40364-021-00333-5
- Kumar S, Wilkes DW, Samuel N, et al. Δnp63-driven recruitment of myeloid-derived suppressor cells promotes metastasis in triple-negative breast cancer. J Clin Invest. 2018;128(11):5095-5109. doi: 10.1172/JCI99673
- Nefedova Y, Fishman M, Sherman S, Wang X, Beg AA, Gabrilovich DI. Mechanism of all-trans retinoic acid effect on tumor-associated myeloid-derived suppressor cells. Cancer Res. 2007;67(22):11021-11028. doi: 10.1158/0008-5472.CAN-07-2593
- Paroni G, Zanetti A, Barzago MM, et al. Retinoic acid sensitivity of triple-negative breast cancer cells characterized by constitutive activation of the notch1 pathway: The role of rarβ. Cancers (Basel). 2020;12(10):3027. doi: 10.3390/cancers12103027
- Lim C, Hwang D, Yazdimamaghani M, et al. High-dose paclitaxel and its combination with CSF1R inhibitor in polymeric micelles for chemoimmunotherapy of triple-negative breast cancer. Nano Today. 2023;51:101884. doi: 10.1016/j.nantod.2023.101884
- Connolly RM, Rudek MA, Piekarz R. Entinostat: A promising treatment option for patients with advanced breast cancer. Future Oncol. 2017;13(13):1137-1148. doi: 10.2217/fon-2016-0526
- Fuso P, Muratore M, D’angelo T, et al. PI3K inhibitors in advanced breast cancer: The past, the present, new challenges and future perspectives. Cancers (Basel). 2022;14:2161. doi: 10.3390/cancers14092161
- Cinier J, Hubert M, Besson L, et al. Recruitment and expansion of Tregs cells in the tumor environment-how to target them? Cancers (Basel). 2021;13(8):1850. doi: 10.3390/cancers13081850
- Plitas G, Konopacki C, Wu K, et al. Regulatory T cells exhibit distinct features in human breast cancer. Immunity. 2016;45(5):1122-1134. doi: 10.1016/j.immuni.2016.10.032
- Liu F, Lang R, Zhao J, et al. CD8+ cytotoxic T cell and FOXP3+ regulatory T cell infiltration in relation to breast cancer survival and molecular subtypes. Breast Cancer Res Treat. 2011;130(2):645-655. doi: 10.1007/s10549-011-1647-3
- Yeong J, Thike AA, Lim JCT, et al. Higher densities of Foxp3+ regulatory T cells are associated with better prognosis in triple-negative breast cancer. Breast Cancer Res Treat. 2017;163(1):21-35. doi: 10.1007/s10549-017-4161-4
- Letendre P, Monga V, Milhem M, Zakharia Y. Ipilimumab: From preclinical development to future clinical perspectives in melanoma. Future Oncol. 2017;13:625-636. doi: 10.2217/fon-2016-0385
- Sobhani N, Tardiel-Cyril DR, Davtyan A, Generali D, Roudi R, Li Y. CTLA-4 in regulatory T cells for cancer immunotherapy. Cancers (Basel). 2021;13:1440. doi: 10.3390/cancers13061440
- Howard FM, Pearson AT, Nanda R. Clinical trials of immunotherapy in triple-negative breast cancer. Breast Cancer Res Treat. 2022;195:1-15. doi: 10.1007/s10549-022-06665-6
- Takahashi M, Cortés J, Dent R, et al. Pembrolizumab plus chemotherapy followed by pembrolizumab in patients with early triple-negative breast cancer: A secondary analysis of a randomized clinical trial. JAMA Netw Open. 2023;6(11):E2342107. doi: 10.1001/jamanetworkopen.2023.42107.
- Fattori S, Roy A Le, Houacine J, et al. CD25high effector regulatory T cells hamper responses to PD-1 blockade in triple-negative breast cancer. Cancer Res. 2023;83(18):3026-3044. doi: 10.1158/0008-5472.CAN-23-0613
- Strauss J, Heery CR, Schlom J, et al. Phase I trial of M7824 (MSB0011359C), a bifunctional fusion protein targeting PD-L1 and TGFβ, in advanced solid tumors. Clin Cancer Res. 2018;24(6):1287-1295. doi: 10.1158/1078-0432.CCR-17-2653
- Bhola NE, Balko JM, Dugger TC, et al. TGF-β inhibition enhances chemotherapy action against triple-negative breast cancer. J Clin Invest. 2013;123(3):1348-1358. doi: 10.1172/JCI65416
- Hu C, Liu X, Zeng Y, Liu J, Wu F. DNA methyltransferase inhibitors combination therapy for the treatment of solid tumors: mechanism and clinical application. Clin Epigenetics. 2021;13:166. doi: 10.1186/s13148-021-01154-x
- Terranova-Barberio M, Thomas S, Ali N, et al. HDAC inhibition potentiates immunotherapy in triple-negative breast cancer. Oncotarget. 2017;8(29):48223-48235. doi: 10.18632/oncotarget.23169
- Zahran AM, El-Badawy O, Kamel LM, Rayan A, Rezk K, Abdel-Rahim MH. Accumulation of regulatory T cells in triple-negative breast cancer can boost immune disruption. Cancer Manag Res. 2021;13:6019-6029. doi: 10.2147/CMAR.S285128
- Oshi M, Asaoka M, Tokumaru Y, et al. Abundance of regulatory T cell (Treg) as a predictive biomarker for neoadjuvant chemotherapy in triple-negative breast cancer. Cancers (Basel). 2020;12(10):3038. doi: 10.3390/cancers12103038
- Qin D, Zhang Y, Shu P, Lei Y, Li X, Wang Y. Targeting tumor-infiltrating Tregs for improved antitumor responses. Front Immunol. 2024;15:1325946. doi: 10.3389/fimmu.2024.1325946
- Qiu X, Zhao T, Luo R, Qiu R, Li Z. Tumor-associated macrophages: Key players in triple-negative breast cancer. Front Oncol. 2022;12:772615. doi: 10.3389/fonc.2022.772615
- Bottai G, Raschioni C, Székely B, et al. AXL-associated tumor inflammation as a poor prognostic signature in chemotherapy-treated triple-negative breast cancer patients. NPJ Breast Cancer. 2016;2(1):16033. doi: 10.1038/npjbcancer.2016.33
- Noy R, Pollard JW. Tumor-associated macrophages: From mechanisms to therapy. Immunity. 2014;41:49-61. doi: 10.1016/j.immuni.2014.06.010
- Hollmén M, Karaman S, Schwager S, et al. G-CSF regulates macrophage phenotype and associates with poor overall survival in human triple-negative breast cancer. Oncoimmunology. 2016;5(3):e1115177. doi: 10.1080/2162402X.2015.1115177
- Jamiyan T, Kuroda H, Yamaguchi R, Abe A, Hayashi M. CD68- and CD163-positive tumor-associated macrophages in triple negative cancer of the breast. Virchows Arch. 2020;477:767-775. doi: 10.1007/s00428-020-02855-z
- Wang J, Chen H, Chen X, Lin H. Expression of tumor-related macrophages and cytokines after surgery of triple-negative breast cancer patients and its implications. Med Sci Monit. 2016;22:115-120. doi: 10.12659/msm.895386
- Niu M, Valdes S, Naguib YW, Hursting SD, Cui Z. Tumor-associated macrophage-mediated targeted therapy of triple-negative breast cancer. Mol Pharm. 2016;13(6):1833-1842. doi: 10.1021/acs.molpharmaceut.5b00987
- Rogers TL, Holen I. Tumour macrophages as potential targets of bisphosphonates. J Transl Med. 2011;9:177. doi: 10.1186/1479-5876-9-177
- Yang Q, Guo N, Zhou Y, Chen J, Wei Q, Han M. The role of tumor-associated macrophages (TAMs) in tumor progression and relevant advance in targeted therapy. Acta Pharm Sin B. 2020;10:2156-2170. doi: 10.1016/j.apsb.2020.04.004
- Panjarian S, Issa JPJ. The roles of DNA demethylases in triple-negative breast cancer. Pharmaceuticals (Basel). 2021;14:628. doi: 10.3390/ph14070628
- Malpeli G, Innamorati G, Decimo I, et al. Methylation dynamics of RASSF1A and its impact on cancer. Cancers (Basel). 2019;11:959. doi: 10.3390/cancers11070959
- Saelee P, Pongtheerat T. APC promoter hypermethylation as a prognostic marker in breast cancer patients. Asian Pac J Cancer Prev. 2020;21(12):3627-3632. doi: 10.31557/APJCP.2020.21.12.3627
- Chien YC, Liu LC, Ye HY, Wu JY, Yu YL. EZH2 promotes migration and invasion of triple-negative breast cancer cells via regulating TIMP2-MMP-2/-9 pathway. Am J Cancer Res. 2018;8:422-434.
- Yang H, Salz T, Zajac-Kaye M, Liao D, Huang S, Qiu Y. Overexpression of histone deacetylases in cancer cells is controlled by interplay of transcription factors and epigenetic modulators. FASEB J. 2014;28(10):4265-4279. doi: 10.1096/fj.14-250654
- Title AC, Silva PN, Godbersen S, Hasenöhrl L, Stoffel M. The miR-200-Zeb1 axis regulates key aspects of β-cell function and survival in vivo. Mol Metab. 2021;53:101267. doi: 10.1016/j.molmet.2021.101267
- Miller TE, Ghoshal K, Ramaswamy B, et al. MicroRNA-221/222 confers tamoxifen resistance in breast cancer by targeting p27Kip1. J Biol Chem. 2008;283(44):29897-29903. doi: 10.1074/jbc.M804612200
- Zhang Z, Zhou C, Li X, et al. Loss of CHD1 promotes heterogeneous mechanisms of resistance to AR-targeted therapy via chromatin dysregulation. Cancer Cell. 2020;37(4):584-598.e11. doi: 10.1016/j.ccell.2020.03.001
- Cortazar P, Zhang L, Untch M, et al. Pathological complete response and long-term clinical benefit in breast cancer: The CTNeoBC pooled analysis. Lancet. 2014;384(9938):164-172. doi: 10.1016/S0140-6736(13)62422-8
- Kagihara JA, Andress M, Diamond JR. Nab-paclitaxel and atezolizumab for the treatment of PD-L1-positive, metastatic triple-negative breast cancer: Review and future directions. Expert Rev Precis Med Drug Dev. 2020;5(2):59. doi: 10.1080/23808993.2020.1730694
- Adams S, Schmid P, Rugo HS, et al. Pembrolizumab monotherapy for previously treated metastatic triple-negative breast cancer: Cohort A of the phase II KEYNOTE-086 study. Ann Oncol. 2019;30(3):397-404. doi: 10.1093/annonc/mdy517
- Ozaki Y, Matsumoto K, Takahashi M, et al. Phase II study of a combination therapy of nivolumab, bevacizumab and paclitaxel in patients with HER2-negative metastatic breast cancer as a first-line treatment (WJOG9917B, NEWBEAT trial). J Clin Oncol. 2018;36(15_suppl):TPS1110. doi: 10.1016/j.ejca.2022.05.014
- Chen F, Chen N, Gao Y, Jia L, Lyu Z, Cui J. Clinical progress of PD-1/L1 inhibitors in breast cancer immunotherapy. Front Oncol. 2022;11:724424. doi: 10.3389/fonc.2021.724424
- Li Y, Zhang H, Merkher Y, et al. Recent advances in therapeutic strategies for triple-negative breast cancer. J Hematol Oncol. 2022;15:121. doi: 10.1186/s13045-022-01341-0
- Dirix LY, Takacs I, Jerusalem G, et al. Avelumab, an anti- PD-L1 antibody, in patients with locally advanced or metastatic breast cancer: A phase 1b JAVELIN solid tumor study. Breast Cancer Res Treat. 2018;167(3):671-686. doi: 10.1007/s10549-017-4537-5
- Xie Y, Hu Y, Zhou N, et al. CAR T-cell therapy for triple-negative breast cancer: Where we are. Cancer Lett. 2020;491:121-131. doi: 10.1016/j.canlet.2020.07.044
- Simó-Riudalbas L, Esteller M. Targeting the histone orthography of cancer: Drugs for writers, erasers and readers. Br J Pharmacol. 2014;172(11):2716-2732. doi: 10.1111/bph.12844
- Skvortsova K, Stirzaker C, Taberlay P. The DNA methylation landscape in cancer. Essays Biochem. 2019;63:797-811. doi: 10.1042/EBC20190037
- Shukla A, Cloutier M, Santharam MA, Ramanathan S, Ilangumaran S. The MHC class-I transactivator NLRC5: Implications to cancer immunology and potential applications to cancer immunotherapy. Int J Mol Sci. 2021;22:1964. doi: 10.3390/ijms22041964
- Zeiner PS, Zinke J, Kowalewski DJ, et al. CD74 regulates complexity of tumor cell HLA class II peptidome in brain metastasis and is a positive prognostic marker for patient survival. Acta Neuropathol Commun. 2018;6(1):18. doi: 10.1186/s40478-018-0521-5
- Park SY, Park JW, Chun YS. Jumonji histone demethylases as emerging therapeutic targets. Pharmacol Res. 2016;105:146-151. doi: 10.1016/j.phrs.2016.01.026
- Guo L, Sang M, Liu Q, Fan X, Zhang X, Shan B. The expression and clinical significance of melanoma-associated antigen-A1, -A3 and -A11 in glioma. Oncol Lett. 2013;6(1):55-62. doi: 10.3892/ol.2013.1304
- Won JK, Bakhoum SF. The cytosolic DNA-sensing cGAS-STING pathway in cancer. Cancer Discov. 2020;10:26-39. doi: 10.1158/2159-8290.CD-19-0761
- Andres F, Yufeng L, Dongquan C, et al. Expression of the MHC class II pathway in triple-negative breast cancer tumor cells is associated with a good prognosis and infiltrating lymphocytes. Cancer Immunol Res. 2016;4(5):390-399. doi: 10.1158/2326-6066.CIR-15-0243
- Stewart RL, Matynia AP, Factor RE, Varley KE. Spatially-resolved quantification of proteins in triple-negative breast cancers reveals differences in the immune microenvironment associated with prognosis. Sci Rep. 2020;10(1):6598. doi: 10.1038/s41598-020-63539-x
- Luo N, Nixon MJ, Gonzalez-Ericsson PI, et al. DNA methyltransferase inhibition upregulates MHC-I to potentiate cytotoxic T lymphocyte responses in breast cancer. Nat Commun. 2018;9(1):248. doi: 10.1038/s41467-017-02630-w
- Kakimi K, Karasaki T, Matsushita H, Sugie T. Advances in personalized cancer immunotherapy. Breast Cancer. 2017;24(1):16-24. doi: 10.1007/s12282-016-0688-1
- Sasidharan Nair V, El Salhat H, Taha RZ, John A, Ali BR, Elkord E. DNA methylation and repressive H3K9 and H3K27 trimethylation in the promoter regions of PD-1, CTLA-4, TIM-3, LAG-3, TIGIT, and PD-L1 genes in human primary breast cancer. Clin Epigenetics. 2018;10(1):78. doi: 10.1186/s13148-018-0512-1
- Li YC, Zhou Q, Song QK, et al. Overexpression of an immune checkpoint (CD155) in breast cancer associated with prognostic significance and exhausted tumor-infiltrating lymphocytes: A cohort study. J Immunol Res. 2020;2020:3948928. doi: 10.1155/2020/3948928
- Huang W, Zhu Q, Shi Z, et al. Dual inhibitors of DNMT and HDAC induce viral mimicry to induce antitumor immunity in breast cancer. Cell Death Discov. 2024;10(1):143. doi: 10.1038/s41420-024-01895-7
- Sheikh TN, Chen X, Xu X, et al. Growth inhibition and induction of innate immune signaling of chondrosarcomas with epigenetic inhibitors. Mol Cancer Ther. 2021;20(12):2362-2371. doi: 10.1158/1535-7163.MCT-21-0066
- Kiyozumi Y, Baba Y, Okadome K, et al. Indoleamine 2,3-dioxygenase 1 promoter hypomethylation is associated with poor prognosis in patients with esophageal cancer. Cancer Sci. 2019;110(6):1863-1871. doi: 10.1111/cas.14028
- Zhai L, Bell A, Ladomersky E, et al. Immunosuppressive IDO in cancer: Mechanisms of action, animal models, and targeting strategies. Front Immunol. 2020;11:1185. doi: 10.3389/fimmu.2020.01185
- Hosseini A, Gharibi T, Marofi F, Babaloo Z, Baradaran B. CTLA-4: From mechanism to autoimmune therapy. Int Immunopharmacol. 2020;80:106221. doi: 10.1016/j.intimp.2020.106221
- Colamatteo A, Carbone F, Bruzzaniti S, et al. Molecular mechanisms controlling Foxp3 expression in health and autoimmunity: From epigenetic to post-translational regulation. Front Immunol. 2020;10:3136.doi: 10.3389/fimmu.2019.03136
- Wang X, Brea LT, Yu J. Immune modulatory functions of EZH2 in the tumor microenvironment: Implications in cancer immunotherapy. Am J Clin Exp Urol. 2019;7:85-91.
- Wong KK. DNMT1: A key drug target in triple-negative breast cancer. Semin Cancer Biol. 2021;72:198-213. doi: 10.1016/j.semcancer.2020.05.010
- Ropero S, Esteller M. The role of histone deacetylases (HDACs) in human cancer. Mol Oncol. 2007;1:19-25. doi: 10.1016/j.molonc.2007.02.001
- Morand S, Stanbery L, Walter A, Rocconi RP, Nemunaitis J. BRCA1/2 mutation status impact on autophagy and immune response: Unheralded target. JNCI Cancer Spectrum. 2021;4(6):pkaa077. doi: 10.1093/jncics/pkaa077
- Kim S, Park S, Cho MS, Lim W, Moon BI, Sung SH. Strong correlation of indoleamine 2,3-dioxygenase 1 expression with basal-like phenotype and increased lymphocytic infiltration in triple-negative breast cancer. J Cancer. 2017;8(1):124-130. doi: 10.7150/jca.17437
- Dill EA, Dillon PM, Bullock TN, Mills AM. IDO expression in breast cancer: An assessment of 281 primary and metastatic cases with comparison to PD-L1. Mod Pathol. 2018;31(10):1513-1522. doi: 10.1038/s41379-018-0061-3
- Asghar K, Loya A, Rana IA, et al. Association between cyclooxygenase-2 and indoleamine 2,3-dioxygenase expression in breast cancer patients from Pakistan. Asian Pac J Cancer Prev. 2019;20(11):3521-3525. doi: 10.31557/APJCP.2019.20.11.3521
- Costa RLB, Czerniecki BJ. Clinical development of immunotherapies for HER2+ breast cancer: A review of HER2-directed monoclonal antibodies and beyond. NPJ Breast Cancer. 2020;6:10. doi: 10.1038/s41523-020-0153-3
- Jing R, Bai S, Zhang P, et al. IDO-1 impairs antitumor immunity of natural killer cells in triple-negative breast cancer via up-regulation of HLA-G. Breast Cancer. 2024;31(1):135-147. doi: 10.1007/s12282-023-01522-w
- Carter JM, Polley MYC, Leon-Ferre RA, et al. Characteristics and spatially defined immune (micro) landscapes of early-stage PD-L1-positive triple-negative breast cancer. Clin Cancer Res. 2021;27(20):5628-5637. doi: 10.1158/1078-0432.CCR-21-0343
- Keenan TE, Tolaney SM. Role of immunotherapy in triple-negative breast cancer. J Natl Compr Canc Netw. 2020;18:479-489. doi: 10.6004/jnccn.2020.7554
- Jacquemier J, Bertucci F, Finetti P, et al. High expression of indoleamine 2,3-dioxygenase in the tumour is associated with medullary features and favourable outcome in basal-like breast carcinoma. Int J Cancer. 2012;130(1):96-104. doi: 10.1002/ijc.25979
- Noonepalle SK, Gu F, Lee EJ, et al. Promoter methylation modulates indoleamine 2,3-dioxygenase 1 induction by activated T cells in human breast cancers. Cancer Immunol Res. 2017;5(4):330-344. doi: 10.1158/2326-6066.CIR-16-0182
- Spranger S, Spaapen RM, Zha Y, et al. Up-regulation of PD-L1, IDO, and T(regs) in the melanoma tumor microenvironment is driven by CD8(+) T cells. Sci Transl Med. 2013;5:200ra116. doi: 10.1126/scitranslmed.3006504
- Song X, Si Q, Qi R, et al. Indoleamine 2,3-dioxygenase 1: A promising therapeutic target in malignant tumor. Front Immunol. 2021;12:800630. doi: 10.3389/fimmu.2021.800630.
- Zhai L, Spranger S, Binder DC, et al. Molecular pathways: Targeting IDO1 and other tryptophan dioxygenases for cancer immunotherapy. Clin Cancer Res. 2015;21:5427-5433. doi: 10.1158/1078-0432.CCR-15-0420
- Sun L. Advances in the discovery and development of selective heme-displacing IDO1 inhibitors. Expert Opin Drug Discov. 2020;15:1223-1232. doi: 10.1080/17460441.2020.1781811
- Pallotta MT, Rossini S, Suvieri C, et al. Indoleamine 2,3-dioxygenase 1 (IDO1): An up-to-date overview of an eclectic immunoregulatory enzyme. FEBS J. 2022;289(20):6099-6118. doi: 10.1111/febs.16086
- Tomek P, Palmer BD, Flanagan JU, Sun C, Raven EL, Ching LM. Discovery and evaluation of inhibitors to the immunosuppressive enzyme indoleamine 2,3-dioxygenase 1 (IDO1): Probing the active site-inhibitor interactions. Eur J Med Chem. 2017;126:983-996. doi: 10.1016/j.ejmech.2016.12.029
- Munn DH, Mellor AL. IDO in the tumor microenvironment: Inflammation, counter-regulation, and tolerance. Trends Immunol. 2016;37:193-207. doi: 10.1016/j.it.2016.01.002