Technology overview and investigation of the quality of a 3D-printed maraging steel demonstration part
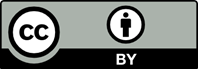
Additive manufacturing (AM) has gained significant traction in the production of high-performance metallic components, yet concerns persist regarding the consistency of powder materials and the mechanical properties of 3D-printed parts. This study addresses these challenges through a detailed analysis of a maraging steel part manufactured using laser powder bed fusion. The demonstration part was evaluated for geometric accuracy, surface roughness, chemical composition, microstructure, and mechanical properties, including hardness and density. The findings revealed that 3D-printed maraging steel components can achieve high levels of dimensional precision and mechanical integrity, making them suitable for demanding applications. Despite these promising results, the study highlighted the need for improved powder quality control and accurate composition measurement to ensure the consistent production of reliable parts. The non-destructive hardness testing method applied in this study proved effective for predicting tensile strength, offering a streamlined approach to quality assurance. These results contribute to a growing body of research and knowledge supporting the adoption of AM for producing critical mechanical components, while underscoring the need for further investigation into quality assurance and standardized non-destructive testing procedures for high-performance metal AM parts.
- Thompson MK, Moroni G, Vaneker T, et al. Design for additive manufacturing: Trends, opportunities, considerations, and constraints. CIRP Ann. 2016;65:737-760. doi: 10.1016/j.cirp.2016.05.004
- Attaran M. The rise of 3-D printing: The advantages of additive manufacturing over traditional manufacturing. Bus Horiz. 2017;60:677-688. doi: 10.1016/j.bushor.2017.05.011
- Grasso M, Colosimo BM. Process defects and in situ monitoring methods in metal powder bed fusion: A review. Meas Sci Technol. 2017;28:044005. doi: 10.1088/1361-6501/aa5c4f
- Lewandowski JJ, Seifi M. Metal additive manufacturing: A review of mechanical properties. Ann Rev Mater Res. 2016;46:151-186. doi: 10.1146/annurev-matsci-070115-0320245
- ASTM. Additive Manufacturing-General Principles- Fundamentals and Vocabulary (ISO/ASTM 52900:2021). United States: ASTM International; 2021. doi: 10.1520/F3177-21
- SLM Solutions Group AG. Available from: https://www.slm-solutions.com [Last accessed on 2021 Sep 16].
- EOS GmbH-Electro Optical Systems. Available from: https://www.eos.info [Last accessed on 2021 Sep 16].
- Concept Laser GmbH/GE Additive. Available from: https:// www.ge.com/additive/who-we-are/concept-laser [Last accessed on 2021 Sep 16].
- Trumpf GmbH & Co. KG. Available from: https://www. trumpf.com [Last accessed on 2021 Sep 16].
- DM3D Technology, LLC. Available from: https://dm3dtech. com [Last accessed on 2021 Sep 16].
- Optomec Inc. Available from: https://optomec.com [Last accessed on 2021 Sep 16].
- Herzog D, Seyda V, Wycisk E, Emmelmann C. Additive manufacturing of metals. Acta Mater. 2016;117:371-392. doi: 10.1016/j.actamat.2016.07.019
- Chua C, Liu Y, Williams RJ, Chua CK, Sing SL. In-process and post-process strategies for part quality assessment in metal powder bed fusion: A review. J Manuf Syst. 2024;73:75-105. doi: 10.1016/j.jmsy.2024.01.004
- Thiede T, Mishurova T, Evsevleev S, Serrano-Munoz I, Gollwitzer C, Bruno G. 3D shape analysis of powder for laser beam melting by synchrotron X-ray CT. Quantum Beam Sci. 2019;3:3. doi: 10.3390/qubs3010003
- Zapico P, Giganto S, Barreiro J, Martínez-Pellitero S. Characterisation of 17-4PH metallic powder recycling to optimise the performance of the selective laser melting process. J Mater Res Technol. 2020;9:1273-1285. doi: 10.1016/j.jmrt.2019.11.054
- Aboulkhair NT, Simonelli M, Parry L, Ashcroft I, Tuck C, Hague R. 3D printing of aluminium alloys: Additive manufacturing of aluminium alloys using selective laser melting. Prog Mater Sci. 2019;106:100578. doi: 10.1016/j.pmatsci.2019.100578
- Sutton AT, Kriewall CS, Leu MC, Newkirk JW. Powder characterisation techniques and effects of powder characteristics on part properties in powder-bed fusion processes. Virtual Phys Prototyp. 2017;12:3-29. doi: 10.1080/17452759.2016.1250605
- Tian Y, Gora WS, Cabo AP, et al. Material interactions in laser polishing powder bed additive manufactured Ti6Al4V components. Addit Manuf. 2018;20:11-22. doi: 10.1016/j.addma.2017.12.010
- Zhang B, Li Y, Bai Q. Defect formation mechanisms in selective laser melting: A review. Chin J Mech Eng. 2017;30:515-527. doi: 10.1007/s10033-017-0121-5
- Malekipour E, El-Mounayri H. Common defects and contributing parameters in powder bed fusion AM process and their classification for online monitoring and control: A review. Int J Adv Manuf Technol. 2018;95:527-550. doi: 10.1007/s00170-017-1172-6
- Murr LE, Martinez E, Hernandez J, et al. Microstructures and properties of 17-4 PH stainless steel fabricated by selective laser melting. J Mater Res Technol. 2012;1:167-177. doi: 10.1016/s2238-7854(12)70029-7
- Facchini L, Vicente N Jr., Lonardelli I, Magalini E, Robotti P, Molinari A. Metastable austenite in 17-4 precipitation-hardening stainless steel produced by selective laser melting. Adv Eng Mater. 2010;12:184-188. doi: 10.1002/adem.200900259
- LeBrun T, Nakamoto T, Horikawa K, Kobayashi H. Effect of retained austenite on subsequent thermal processing and resultant mechanical properties of selective laser melted 17-4 PH stainless steel. Mater Des. 2015;81:44-53. doi: 10.1016/j.matdes.2015.05.026
- Kempen K, Yasa E, Thijs L, Kruth JP, Humbeeck JV. Microstructure and mechanical properties of selective laser melted 18Ni-300 steel. Phys Procedia. 2011;12:255-263. doi: 10.1016/j.phpro.2011.03.033
- Jägle EA, Choi PP, van Humbeeck J, Raabe D. Precipitation and austenite reversion behavior of a maraging steel produced by selective laser melting. J Mater Res. 2014;29:2072-2079. doi: 10.1557/jmr.2014.204
- Krakhmalev P, Yadroitsava I, Fredriksson G, Yadroitsev I. In situ heat treatment in selective laser melted martensitic AISI420 stainless steels. Mater Des. 2015;87:380-385. doi: 10.1016/j.matdes.2015.08.045
- Niendorf T, Leuders S, Riemer A, Richard HA, Tröster T, Schwarze D. Highly anisotropic steel processed by selective laser melting. Metall Mater Trans B. 2013;44:794-796. doi: 10.1007/s11663-013-9875-z
- Zhao X, Song B, Zhang Y, Zhu X, Wei Q, Shi Y. Decarburization of stainless steel during selective laser melting and its influence on Young’s modulus, hardness and tensile strength. Mater Sci Eng A. 2015;647:58-61. doi: 10.1016/j.msea.2015.08.061
- Song B, Zhao X, Li S, et al. Differences in microstructure and properties between selective laser melting and traditional manufacturing for fabrication of metal parts: A review. Front Mech Eng. 2015;10:111-125. doi: 10.1007/s11465-015-0341-2
- Majumdar JD, Pinkerton A, Liu Z, Manna I, Li L. Microstructure characterization and process optimization of laser assisted rapid fabrication of 316L stainless steel. Appl Surf Sci. 2005;247:320-327. doi: 10.1016/j.apsusc.2005.01.039
- Abd-Elghany K, Bourell D. Property evaluation of 304L stainless steel fabricated by selective laser melting. Rapid Prototyp J. 2012;18:420-428. doi: 10.1108/13552541211250418
- Carlton HD, Haboub A, Gallegos GF, Parkinson DY, MacDowell AA. Damage evolution and failure mechanisms in additively manufactured stainless steel. Mater Sci Eng A. 2016;651:406-414. doi: 10.1016/j.msea.2015.10.073
- Xu W, Brandt M, Sun S, et al. Additive manufacturing of strong and ductile Ti-6Al-4V by selective laser melting via in situ martensite decomposition. Acta Mater. 2015;85:74-84. doi: 10.1016/j.actamat.2014.11.028
- Thijs L, Sistiaga MLM, Wauthle R, Xie Q, Kruth JP, Humbeeck JV. Strong morphological and crystallographic texture and resulting yield strength anisotropy in selective laser melted tantalum. Acta Mater. 2013;61:4657-4668. doi: 10.1016/j.actamat.2013.04.036
- Carroll BE, Palmer TA, Beese AM. Anisotropic tensile behavior of Ti-6Al-4V components fabricated with directed energy deposition additive manufacturing. Acta Mater. 2015;87:309-320. doi: 10.1016/j.actamat.2014.12.054
- Wu W, Wang X, Wang Q, et al. Microstructure and mechanical properties of maraging 18Ni-300 steel obtained by powder bed based selective laser melting process. Rapid Prototyp J. 2020;26:1379-1387. doi: 10.1108/rpj-08-2018-0189
- Yao Y, Wang K, Wang X, et al. Microstructural heterogeneity and mechanical anisotropy of 18Ni-330 maraging steel fabricated by selective laser melting: The effect of build orientation and height. J Mater Res. 2020;35:2065-2076. doi: 10.1557/jmr.2020.126
- Mooney B, Kourousis K. A review of factors affecting the mechanical properties of maraging steel 300 fabricated via laser powder bed fusion. Metals. 2020;10:1273. doi: 10.3390/met10091273
- Takata N, Nishida R, Suzuki A, Kobashi M, Kato M. Crystallographic features of microstructure in maraging steel fabricated by selective laser melting. Metals. 2018;8:440. doi: 10.3390/met8060440
- Song J, Tang Q, Feng Q, et al. Effect of heat treatment on microstructure and mechanical behaviours of 18Ni-300 maraging steel manufactured by selective laser melting. Opt Laser Technol. 2019;120:105725. doi: 10.1016/j.optlastec.2019.105725
- Kürnsteiner P, Barriobero-Vila P, Bajaj P, et al. Designing an Fe-Ni-Ti maraging steel tailor-made for laser additive manufacturing. Addit Manuf. 2023;73:103647. doi: 10.1016/j.addma.2023.103647
- Su J, Li Q, Teng J, et al. Programmable mechanical properties of additively manufactured novel steel. Int J Extrem Manuf. 2024;7:015001. doi: 10.1088/2631-7990/ad88bc
- ASM International Handbook Committee. Properties and Selection: Irons, Steels, and High-Performance Alloys. Vol. 1. United States: ASM International; 1990. doi: 10.31399/asm.hb.v01.9781627081610
- Wikipedia. Maraging Steel. The Free Encyclopedia; 2021. Available from: https://en.wikipedia.org/w/index. php?title=maraging%20steel&oldid=1031815960 [Last accessed on 2021 Sep 16].
- Hall A, Slunder C. The Metallurgy, Behavior, and Application of the 18 Percent Nickel Maraging Steels. Report SP-5051. National Aeronautics and Space Administration (NASA); 1968.
- NiDI. The 18 Percent Nickel Maraging Steels: Engineering Analysis. Publication No. 4419. Canada: Nickel Development Institute (NiDI); 1976.
- Protolabs. Maraging Steel 1.2709. Protolabs. Available from: https://www.protolabs.com/media/1022869/maraging-steel-1.pdf [Last accessed on 2025 Feb 26].
- ASM International Handbook Committee. Additive Manufacturing Processes. Vol. 24. United States: ASM International; 2020. doi: 10.31399/asm.hb.v24.9781627082907
- Pannitz O, Sehrt JT. Transferability of process parameters in laser powder bed fusion processes for an energy and cost efficient manufacturing. Sustainability. 2020;12:1565. doi: 10.3390/su12041565
- Yun H, Dong L, Wang W, Bing Z, Xiangyun L. Study on the flowability of TC4 alloy powder for 3D printing. IOP Conf Ser Mater Sci Eng. 2018;439:042006. doi: 10.1088/1757-899x/439/4/042006
- Pagác M, Hajnys J, Petru J, Zlámal T. Comparison of hardness of surface 316L stainless steel made by additive technology and cold rolling. Mater Sci Forum. 2018;919:84-91. doi: 10.4028/www.scientific.net/msf.919.84
- Liverani E, Toschi S, Ceschini L, Fortunato A. Effect of selective laser melting (SLM) process parameters on microstructure and mechanical properties of 316L austenitic stainless steel. J Mater Process Technol. 2017;249:255-263. doi: 10.1016/j.jmatprotec.2017.05.042
- Strano G, Hao L, Everson RM, Evans KE. Surface roughness analysis, modelling and prediction in selective laser melting. J Mater Process Technol. 2013;213:589-597. doi: 10.1016/j.jmatprotec.2012.11.011
- Wang D, Liu Y, Yang Y, Xiao D. Theoretical and experimental study on surface roughness of 316L stainless steel metal parts obtained through selective laser melting. Rapid Prototyp J. 2016;22:706-716. doi: 10.1108/RPJ-06-2015-0078
- Leary M. Surface roughness optimisation for selective laser melting (SLM): Accommodating relevant and irrelevant surfaces. In: Brandt M editor. Laser Additive Manufacturing: Materials, Design, Technologies, and Applications. Woodhead Publishing Series in Electronic and Optical Materials. Ch. 4. United Kingdom: Woodhead Publishing; 2017. p. 99-118. doi: 10.1016/B978-0-08-100433-3.00004-X
- Vayssette B, Saintier N, Brugger C, Elmay M, Pessard E. Surface roughness of Ti-6Al-4V parts obtained by SLM and EBM: Effect on the high cycle fatigue life. Procedia Eng. 2018;213:89-97. doi: 10.1016/j.proeng.2018.02.010
- Iron-Foundry. Rockwell Hardness (HRC, HRB) to Brinell Hardness (HB or BHN) Conversion; 2024. Available from: https://www.iron-foundry.com/hardness-hrc-hrb-hb.html [Last accessed on 2024 Aug 05].
- ASTM. Standard Test Methods and Definitions for Mechanical Testing of Steel Products (ASTM A370-24). United States: ASTM International; 2024. doi: 10.1520/A0370-24
- Pavlina E, Tyne CV. Correlation of yield strength and tensile strength with hardness for steels. J Mater Eng Perform. 2008;17:888-893. doi: 10.1007/s11665-008-9225-5