Network pharmacology-based findings of the immunomodulatory activity of phytocompounds from Withania somnifera and Aloe barbadensis
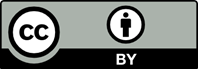
Immunomodulation constitutes a crucial part of individual organisms’ defense systems. Moreover, the utilization of plant-based natural products as herbal medicine for immunomodulation has garnered significant interest. Herein, we examined the immunomodulatory potentials of active phytocompounds extracted from Withania somnifera and Aloe barbadensis by employing ADMET screening, network pharmacology, and molecular docking techniques. This study follows the paradigm in drug discovery, which has shifted from a “one-target, one-drug” mode to a “network-target, multiple-component-therapeutics” mode. Phyto compounds sourced from W. somnifera and A. barbadensis were mined from online databases, including Dr. Duke’s Phytochemical Ethnobotanical Database. After screening these active compounds, their potential targets were predicted through in silico ADMET property prediction models. Network pharmacology was utilized to establish a “compound-protein/gene-disease” network and reveal the regulatory mechanism of small molecules in a high-throughput manner through STRING, Cytohubba plugin in Cytoscape, and the g: Profiler software. A molecular docking simulation was performed to examine the binding affinity between the selected hub targets and bioactives. The findings showed that phytocompounds derived from the W. somnifera and A. barbadensis exhibit immunomodulatory effects by inhibiting specific protein targets, notably AKT1, HCK, JAK2, PDPK1, KIT, and IL2. Molecular docking analysis further revealed the potential of withanolide G, somniferine, and somniferanolide as promising immunomodulatory compounds against HCK, JAK2, and PDPK1 proteins, which are involved in multiple myeloma pathways, encompassing the PI3K-Akt signaling pathway, NOD-like receptor signaling pathway, and Toll-like receptor signaling pathway. In conclusion, these compounds are recommended for further in vivo and in vitro investigations to ascertain their potential as treatments for multiple myeloma.
- Yagi A, Yu BP, 2021, Immune modulation of Aloe vera: Acemannan and gut microbiota modulator. J Gastroenterol Hepatol Res, 4: 1707–1721.
- Ayivi R, Ibrahim S, Colleran H, et al., 2021, COVID-19: Human immune response and the influence of food ingredients and active compounds. Bioactive Compounds Health Dis, 4: 100. https://doi.org/10.31989/bchd.v4i6.802
- Newman DJ, Cragg GM, 2020, Natural products as sources of new drugs over the nearly four decades from 01/1981 to 09/2019. J Nat Prod, 83: 770–803. https://doi.org/10.1021/acs.jnatprod.9b01285
- Tharakan A, Himanshu S, Irin RB, et al., 2021, Immunomodulatory effect of Withania somnifera (Ashwagandha) Extract--a randomized, double-blind, placebo controlled trial with an open label extension on healthy participants. J Clin Med, 10: 3644. https://doi.org/10.3390/jcm10163644
- Afewerky HK, Ayodeji AE, Tiamiyu BB, et al., 2021, Critical review of the Withania somnifera (L.) Dunal: Ethnobotany, pharmacological efficacy, and commercialization significance in Africa. Bull Natl Res Cent, 45: 176. https://doi.org/10.1186/s42269-021-00635-6
- Surjushe A, Vasani R, Saple DG, 2008, Aloe vera: A short review. Indian J Dermatol, 53: 163–166. https://doi.org/10.4103/0019-5154.44785
- Sánchez M, González-Burgos E, Iglesias I, et al., 2020, Pharmacological update properties of Aloe vera and its major active constituents. Molecules, 25: 1324. https://doi.org/10.3390/molecules25061324
- Rafei H, Haroun F, Tabbara IA, 2018, Novel immunotherapeutic agents for the treatment of multiple myeloma. Am J Clin Oncol, 42: 317–329. https://doi.org/10.1097/COC.0000000000000506
- Rajkumar SV, Landgren O, Mateos MV, 2015, Smoldering multiple myeloma. Blood, 125: 3069–3075. https://doi.org/10.1182/blood-2014-09-568899
- Guillerey C, Nakamura K, Vuckovic S, et al., 2016, Immune responses in multiple myeloma: Role of the natural immune surveillance and potential of immunotherapies. Cell Mol Life Sci, 73: 1569–1589. https://doi.org/10.1007/s00018-016-2135-z
- Leelananda SP, Lindert S, 2016, Computational methods in drug discovery. Beilstein J Org Chem, 12: 2694–2718. https://doi.org/10.3762/bjoc.12.267
- Huang C, 2014, Systems pharmacology in drug discovery and therapeutic insight for herbal medicines. Brief Bioinform, 15: 710–733. https://doi.org/10.1093/bib/bbt035
- Zhang R, Zhu X, Bai H, et al., 2019, Network pharmacology databases for traditional Chinese medicine: Review and assessment. Front Pharmacol, 10: 123. https://doi.org/10.3389/fphar.2019.00123
- Kibble M, Saarinen N, Tang J, et al., 2015, Network pharmacology applications to map the unexplored target space and therapeutic potential of natural products. Nat Prod Rep, 32: 1249–1266. https://doi.org/10.1039/c5np00005j
- Mohanraj K, Karthikeyan BS, Vivek-Ananth RP, et al., 2018, IMPPAT: A curated database of Indian medicinal plants, phytochemistry and therapeutics. Sci Rep, 8: 4329. https://doi.org/10.1038/s41598-018-22631-z
- Kim S, Chen J, Cheng T, et al., 2019, PubChem in 2021: New data content and improved web interfaces. Nucleic Acids Res, 49: D1388–D1395. https://doi.org/10.1093/nar/gkaa971
- Ru J, Li P, Wang J, et al., 2014, TCMSP: A database of systems pharmacology for drug discovery from herbal medicines. J Cheminform, 6: 13. https://doi.org/10.1186/1758-2946-6-13
- Aungst BJ, 2017, Optimizing oral bioavailability in drug discovery: An overview of design and testing strategies and formulation options. J Pharm Sci, 106: 921–929. https://doi.org/10.1016/j.xphs.2016.12.002
- Yue SJ, Xin LT, Fan YC, 2017, Herb pair Danggui-Honghua: Mechanisms underlying blood stasis syndrome by system pharmacology approach. Sci Rep, 7: 40318. https://doi.org/10.1038/srep40318
- Lipinski CA, Dominy BW, Feeney PJ, 2012, Experimental and computational approaches to estimate solubility and permeability in drug discovery and development settings. Adv Drug Deliv Rev, 64: 4–17. https://doi.org/10.1016/j.addr.2012.09.019
- Abdul-Hammed M, Adedotun IO, Falade VA, et al., 2021, Target-based drug discovery, ADMET profiling and bioactivity studies of antibiotics as potential inhibitors of SARS-CoV-2 main protease (Mpro). Virusdisease, 32: 642–656. https://doi.org/10.1007/s13337-021-00717-z
- Keiser MJ, Roth BL, Armbruster BN, et al., 2007, Relating protein pharmacology by ligand chemistry. Nat Biotechnol, 25: 197–206. https://doi.org/10.1038/nbt1284
- Daina A, Michielin O, Zoete V, 2019, SwissTargetPrediction: Updated data and new features for efficient prediction of protein targets of small molecules. Nucleic Acids Res, 47: W357–W364. https://doi.org/10.1093/nar/gkz382
- Wang X, Shen Y, Wang S, 2017, PharmMapper 2017 update: A web server for potential drug target identification with a comprehensive target pharmacophore database. Nucleic Acids Res, 45: W356–W360. https://doi.org/10.1093/nar/gkx374
- Gane PJ, Bateman A, Martin MJ, et al., 2014, UniProt: A hub for protein information. Nucleic Acids Res, 43: D204–D212. https://doi.org/10.1093/nar/gku989.
- Safran M, Dalah I, Alexander J, et al., 2018, GeneCards version 3: The human gene integrator. Database (Oxford), 2010: baq020. https://doi.org/10.1093/database/baq020
- Pinero J, Bravo A, Queralt-Rosinach N, et al., 2017, DisGeNET: A comprehensive platform integrating information on human disease-associated genes and variants. Nucleic Acids Res, 45: D833–D839. https://doi.org/10.1093/nar/gkw943
- Oliveros JC, 2007, VENNY. An Interactive Tool for Comparing Lists with Venn Diagrams. Available from: https://bioinfogp.cnb.csic.es/tools/venny/index.html [Last accessed on 2021 Jul 10].
- Szklarczyk D, Franceschini A, Wyder S, et al., 2015, STRING v10: Protein-protein interaction networks, integrated over the tree of life. Nucleic Acids Res, 43: D447–D452. https://doi.org/10.1093/nar/gku1003
- Shannon P, Markiel A, Ozier O, et al., 2003, Cytoscape: A software environment for integrated models of biomolecular interaction networks. Genome Res, 13: 2498–2504. https://doi.org/10.1101/gr.1239303
- Afolayan FID, Tarkaa CT, 2023, Network pharmacology-based assessment of anti-inflammatory action of phytocompounds derived from Nigella sativa and Moringa oleifera. Drug Discov, 17: e13dd1016.
- Barabasi AL, Oltvai ZN, 2004, Network biology: Understanding the cell’s functional organization. Nat Rev Genet, 5: 101–113. https://doi.org/10.1038/nrg1272
- Reimand J, Kull M, Peterson H, et al., 2007, g: Profiler--a web-based toolset for functional profiling of gene lists from large-scale experiments. Nucleic Acids Res, 35: W193–W200. https://doi.org/10.1093/nar/gkm226
- Swamydas M, Murphy EV, Ignatz-Hoover JJ, et al., 2022, Deciphering mechanisms of immune escape to inform immunotherapeutic strategies in multiple myeloma. J Hematol Oncol, 15: 17. https://doi.org/10.1186/s13045-022-01234-2
- Dallakyan S, Olson AJ, 2015, Small-molecule library screening by docking with PyRx. Methods Mol Biol, 1263: 243–250. https://doi.org/10.1007/978-1-4939-2269-7_19
- BIOVIA, 2022, BIOVIA Workbook, Release 2020: BIOVIA Pipeline Pilot, Release 2022. San Diego: Dassault Systèmes.
- Eberhardt J, Santos-Martins D, Tillack AF, et al., 2021, AutoDock vina 1.2.0: New docking methods, expanded force field, and python bindings. J Chem Inform Model, 61: 3891–3898. https://doi.org/10.1021/acs.jcim.1c00203
- Sahu N, Mishra S, Kesheri M, et al., 2022, Identification of Cyanobacteria-based natural inhibitors against SARS-CoV-2 druggable target ACE2 using molecular docking study, ADME and toxicity analysis. Indian J Clin Biochem, 38: 361–373. https://doi.org/10.1007/s12291-022-01056-6
- Cho DY, Kim YA, Przytycka TM, 2012, Chapter 5: Network biology approach to complex diseases. PLoS Comput Biol, 8: e1002820. https://doi.org/10.1371/journal.pcbi.1002820
- Gupta M, Sharma R, Kumar A, 2018, Docking techniques in pharmacology: How much promising? Comput Biol Chem, 76: 210–217. https://doi.org/10.1016/j.compbiolchem.2018.06.005
- Cohen OC, Shadmi E, Keinan-Boker L, et al., 2019, The association between patients’ perceived continuity of care and beliefs about oral anticancer treatment. Supportive Care Cancer, 27: 3545–3553. https://doi.org/10.1007/s00520-019-04668-6
- Medzhitov R, 2007, Recognition of microorganisms and activation of the immune response. Nature, 449: 819–826. https://doi.org/10.1038/nature06246
- Ramakrishnan V, Kumar S, 2018, PI3K/AKT/mTOR pathway in multiple myeloma: From basic biology to clinical promise. Leuk Lymphoma, 59: 2524–2534. https://doi.org/10.1080/10428194.2017.1421760
- McDonald SJ, Sharkey JM, Sun M, et al., 2020, Beyond the brain: Peripheral interactions after traumatic brain injury. J Neurotrauma, 37: 770–781. https://doi.org/10.1089/neu.2019.6885
- Kawai T, Akira S, 2007, Signaling to NF-κB by Toll-like receptors. Trends Mol Med, 13: 460–469. https://doi.org/10.1016/j.molmed.2007.09.002
- Napetschnig J, Wu H, 2013, Molecular basis of NF-κB signaling. Annu Rev Biophys, 42: 443–468. https://doi.org/10.1146/annurev-biophys-083012-130338
- O’Shea JJ, Plenge R, 2012, JAK and STAT signaling molecules in immunoregulation and immune-mediated disease. Immunity, 36: 542–550. https://doi.org/10.1016/j.immuni.2012.03.014
- Hu YS, Han X, Liu XH, 2019, STAT3: A potential drug target for tumor and inflammation. Curr Top Med Chem, 19: 1305–1317. https://doi.org/10.2174/1568026619666190620145052
- Ismail SI, Mahmoud IS, Msallam MM, 2010, Hotspot mutations of PIK3CA and AKT1 genes are absent in multiple myeloma. Leuk Res, 34: 824–826. https://doi.org/10.1016/j.leukres.2009.11.018
- Chang H, Qi XY, Claudio J, 2006, Analysis of PTEN deletions and mutations in multiple myeloma. Leuk Res, 30: 262–265. https://doi.org/10.1016/j.leukres.2005.07.008
- Peterson TR, Laplante M, Thoreen CC, 2009, DEPTOR is an mTOR inhibitor frequently overexpressed in multiple myeloma cells and required for their survival. Cell, 137: 873–886. https://doi.org/10.1016/j.cell.2009.03.046
- Yu CF, Liu ZX, Cantley LG, 2002, ERK negatively regulates the epidermal growth factor-mediated interaction of Gab1 and the phosphatidylinositol 3-kinase. J Biol Chem, 277: 19382–19388. https://doi.org/10.1074/jbc.M200732200
- Narayanankutty A, 2019, PI3K/Akt/mTOR pathway as a therapeutic target for colorectal cancer: A review of preclinical and clinical evidence. Curr Drug Targets, 20: 1217–1226. https://doi.org/10.2174/1389450120666190618123846
- Chang WJ, Gonzalez-Paz N, Price-Troska T, 2008, Clinical and biological significance of RAS mutations in multiple myeloma. Leukemia, 22: 2280–2284. https://doi.org/10.1038/leu.2008.142
- Rasmussen T, Kuehl M, Lodahl M, et al., 2005, Possible roles for activating RAS mutations in the MGUS to MM transition and in the intramedullary to extramedullary transition in some plasma cell tumors. Blood, 105: 317–323. https://doi. org/10.1182/blood-2004-03-0833
- White PT, Subramanian C, Motiwala HF, et al., 2016, Natural withanolides in the treatment of chronic diseases. Adv Exp Med Biol, 928: 329–373. https://doi.org/10.1007/978-3-319-41334-1_14
- Swarnalatha S, Puratchikody A, 2014, Cytokine mediated immunomodulatory properties of kaempferol-5-O-β-D-glucopyranoside from methanol extract of aerial parts of Indigofera aspalathoides Vahl ex DC. Int J Res Pharm Sci, 5: 73–78.
- Choi MJ, Park EJ, Min KJ, et al., 2011, Endoplasmic reticulum stress mediates withaferin A-induced apoptosis in human renal carcinoma cells. Toxicology In Vitro, 25: 692–698. https://doi.org/10.1016/j.tiv.2011.01.010
- Abramson HN, 2016, Kinase inhibitors as potential agents in the treatment of multiple myeloma. Oncotarget,7: 81926–81968. https://doi.org/10.18632/oncotarget.10745
- Manning G, Whyte DB, Martinez R, et al., 2002, The protein kinase complement of the human genome. Science, 298: 1912–1934. https://doi.org/10.1126/science.1075762