In silico evaluation of heat shock proteins reveals an interplay with polyamines as a survival strategy for the Plasmodium falciparum
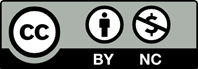
The current drugs available in the market are not effective due to growing numbers of resistance to the causative agent of malaria. There are various Plasmodium parasites, of which Plasmodium falciparum is the main cause of morbidity and mortality reported worldwide. Therefore, there is an urgent need to come up with an innovative and effective treatment for this disease. Polyamines play a major role in the parasite’s well-being and growth, while heat shock proteins keep the proteomics of the parasite in good shape. In this study, an in silico analysis of the interaction between putrescine, spermidine, spermine, and heat shock proteins was carried out to establish the binding site for drug discovery. Computational tools such as Bioedit, PROCHECK, KNIME Hub, and Schrodinger were used. The results revealed interactions between polyamines and heat shock proteins with glutamine and aspartic acid being common amino acids where interaction occurs between the chaperones and polyamines. Molecular dynamics showed a strong interaction between PfHsp70-1 and putrescine, but the best interaction is observed for PfHsp70-1 and spermidine. Based on these results, a follow-up study will be conducted to establish the synthesis of drugs that will be used as targets for both polyamines and heat shock proteins to eradicate malaria.
- WHO, 2020, World Malaria Report 2020: 20 Years of Global Progress and Challenges. Geneva: World Health Organization, p299.
- Varo R, Chaccour C, Bassat Q, 2020, Update on malaria. Med Clín (Barc), 155: 395–402. https://doi.org/10.1016/j.medcli.2020.05.010
- Tse EG, Korsik M, Todd MH, 2019, The past, present and future of anti-malarial medicines. Malar J, 18: 93. https://doi.org/10.1186/s12936-019-2724-z
- Antony HA, Parija SC, 2016, Antimalarial drug resistance: An overview. Trop Parasitol, 6: 30–41. https://doi.org/10.4103/2229-5070.175081
- Mbengue A, Bhattacharjee S, Pandharkar T, et al., 2015, A molecular mechanism of artemisinin resistance in Plasmodium falciparum malaria. Nature, 520: 683–687. https://doi.org/10.1038/nature14412
- Belete TM, 2020, Recent progress in the development of new antimalarial drugs with novel targets. Drug Des Devel Ther, 14: 3875–3889. https://doi.org/10.2147/DDDT.S265602
- Hart RJ, Ghaffar A, Abdalal S, et al., 2016, Plasmodium AdoMetDC/ODC bifunctional enzyme is essential for male sexual stage development and mosquito transmission. Biol Open, 5: 1022–1029. https://doi.org/10.1242/bio.016352
- Müller IB, Gupta RD, Lüersen K, et al., 2008, Assessing the polyamine metabolism of Plasmodium falciparum as chemotherapeutic target. Mol Biochem Parasitol, 160: 1–7. https://doi.org/10.1016/j.molbiopara.2008.03.008
- Pavithra SR, Kumar R, Tatu U, 2007, Systems analysis of chaperone networks in the malarial parasite Plasmodium falciparum. PLoS Comput Biol, 3: 1701–1715. https://doi.org/10.1371/journal.pcbi.0030168
- Jee H, 2016, Size dependent classification of heat shock proteins: A mini-review. J Exerc Rehabil, 12: 255–259. https://doi.org/10.12965/jer.1632642.321
- Miller DJ, Fort PE, 2018, Heat shock proteins regulatory role in neurodevelopment. Front Neurosci, 12: 821. https://doi.org/10.3389/fnins.2018.00821
- Ganea E, 2001, Chaperone-like activity of alpha-crystallin and other small heat shock proteins. Curr Protein Pept Sci, 2: 205–225. https://doi.org/10.2174/1389203013381107
- Wynn RM, Davie JR, Cox RP, et al., 1994, Molecular chaperones: Heat-shock proteins, foldases, and matchmakers. J Lab Clin Med, 124: 31–36.
- Hall TA, 1999, BioEdit: A user-friendly biological sequence alignment editor and analysis program for Windows 95/98/ NT. Nucleic Acids Symp Ser, 41: 95–98.
- Kelley LA, Mezulis S, Yates CM, et al., 2015, The Phyre2 web portal for protein modeling, prediction and analysis. Nat Protoc, 10: 845–858. https://doi.org/10.1038/nprot.2015.053
- Laskowski RA, Jabłońska J, Pravda L, et al., 2018, PDBsum: Structural summaries of PDB entries. Protein Sci, 27: 129–134. https://doi.org/10.1002/pro.3289
- Maestro S, 2020, Schrödinger Release 2020-3: Maestro. New York, USA: Schrödinger, LLC.
- Halgren T, 2007, New method for fast and accurate binding-site identification and analysis. Chem Biol Drug Des, 69: 146–148. https://doi.org/10.1111/j.1747-0285.2007.00483.x
- Halgren TA, 2009, Identifying and characterizing binding sites and assessing druggability. J Chem Inf Model, 49: 377–389. https://doi.org/10.1021/ci800324m
- Berthold MR, Cebron N, Dill F, et al., 2009, KNIME-the Konstanz information miner: Version 2.0 and beyond. AcM SIGKDD Explor Newslett, 11: 26–31. https://doi.org/10.1145/1656274.1656280
- LigPrep S, 2019, Schrödinger Release 2019-4. New York, USA: Schrödinger LLC.
- Wass MN, Kelley LA, Sternberg MJ, 2010, 3DLigandSite: Predicting ligand-binding sites using similar structures. Nucleic Acids Res, 38: W469–W473. https://doi.org/10.1093/nar/gkq406
- Duhovny D, Nussinov R, Wolfson HJ, 2002, Efficient unbound docking of rigid molecules. In: Algorithms in Bioinformatics: Second International Workshop, WABI 2002 Rome, Italy, September 17–21, 2002 Proceedings. Springer Berlin Heidelberg, p185–200.
- Bergdorf M, Robinson-Mosher A, Guo X, et al., 2021, Desmond/GPU Performance as of April 2021. DE Shaw Research, Technical Report DESRES/TR-2021-01.
- Aier I, Varadwaj PK, Raj U, 2016, Structural insights into conformational stability of both wild-type and mutant EZH2 receptor. Sci Rep, 6: 34984. https://doi.org/10.1038/srep34984
- Makhoba XH, 2021, A double line of defense: Heat shock proteins and polyamines act as contributing factors to drug resistance of some Plasmodium parasites. In: Plasmodium Species and Drug Resistance. London: Intechopen.
- Makhoba XH, Viegas C Jr., Mosa RA, et al., 2020, Potential impact of the multi-target drug approach in the treatment of some complex diseases. Drug Des Dev Ther, 14: 3235–3249. https://doi.org/10.2147/DDDT.S257494