Hepatocyte-specific angiotensinogen deficiency inhibits Western diet-induced liver steatosis with suppression of cell division in mice
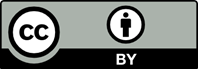
Liver steatosis is a common cause of chronic liver disease. To investigate the molecular basis of hepatic steatosis, low-density lipoprotein receptor-deficient (LDLR -/-) mice were fed a Western diet (WD, 42% of calories from fat) for 5, 14, or 42 days and evaluated against mice fed a normal laboratory diet. Histological analyses revealed that steatosis was detected as early as 14 days of WD feeding. Bulk RNA sequencing demonstrated that WD feeding altered liver transcriptomes related to inflammation and cell adhesion consistent with the progression of liver steatosis. Previous studies determined that hepatocyte-specific deficiency of angiotensinogen (AGT), the unique substrate of the renin-angiotensin system (RAS), alleviates WD-induced hepatic steatosis in mice. However, the effects of hepatic AGT deficiency were not mimicked by pharmacological inhibition of the RAS, and the molecular mechanisms by which AGT deficiency protects against WD-induced steatosis is unknown. Therefore, liver transcriptomes were compared between hepatocyte-specific AGT-deficient mice (hepAGT -/-) and their wild-type littermates (hepAGT +/+) after 14 days of WD feeding. Gene ontology analyses showed that upregulated genes in hepAGT -/- mice were enriched for metabolic processes and downregulated genes were enriched for cell division pathways. The integration analysis of the two RNA sequencing data identified 5 key genes, Smpd3, Dtl, Cdc6, Mki67, and Top2a, which were primarily associated with cell division processes in hepAGT +/+ mice and were suppressed in hepAGT -/- mice. In conclusion, hepatic AGT deficiency downregulated genes related to cell division during the progression of liver steatosis.
- Younossi ZM, Koenig AB, Abdelatif D, Fazel Y, Henry L, Wymer M. Global epidemiology of nonalcoholic fatty liver disease-Meta-analytic assessment of prevalence, incidence, and outcomes. Hepatology. 2016;64:73-84. doi: 10.1002/hep.28431
- Cotter TG, Rinella M. Nonalcoholic fatty liver disease 2020: The state of the disease. Gastroenterology. 2020;158:1851-1864. doi: 10.1053/j.gastro.2020.01.052
- Loomba R, Sanyal AJ. The global NAFLD epidemic. Nat Rev Gastroenterol Hepatol. 2013;10:686-690. doi: 10.1038/nrgastro.2013.171
- Teng ML, Tan DJH, Ng CH, Huang DQ. Hepatocellular carcinoma surveillance in non-alcoholic fatty liver disease-who and how? Clin Mol Hepatol. 2023;29:404-407. doi: 10.3350/cmh.2023.0069
- Gwaltney-Brant SM. In: Gupta RC, editor. Nutraceuticals in Renal Diseases. Ch. 8. United States: Academic Press; 2016. p. 101-108.
- Ma W, Wu W, Wen W, et al. Association of NAFLD with cardiovascular disease and all-cause mortality: A large-scale prospective cohort study based on UK Biobank. Ther Adv Chronic Dis. 2022;13:20406223221122478. doi: 10.1177/20406223221122478
- Engin A. Non-alcoholic fatty liver disease. Adv Exp Med Biol. 2017;960:443-467. doi: 10.1007/978-3-319-48382-5_19
- Yki-Jarvinen H, Luukkonen PK, Hodson L, Moore JB. Dietary carbohydrates and fats in nonalcoholic fatty liver disease. Nat Rev Gastroenterol Hepatol. 2021;18:770-786. doi: 10.1038/s41575-021-00472-y
- Stephenson K, Kennedy L, Hargrove L, et al. Updates on dietary models of nonalcoholic fatty liver disease: Current studies and insights. Gene Expr. 2018;18:5-17. doi: 10.3727/105221617X15093707969658
- Matsusaka T, Niimura F, Shimizu A, et al. Liver angiotensinogen is the primary source of renal angiotensin II. J Am Soc Nephrol. 2012;23:1181-1189. doi: 10.1681/ASN.2011121159
- Lu H, Wu C, Howatt DA, et al. Angiotensinogen exerts effects independent of Angiotensin II. Arterioscler Thromb Vasc Biol. 2016;36:256-265. doi: 10.1161/ATVBAHA.115.306740
- Kukida M, Cai L, Ye D, et al. Renal angiotensinogen is predominantly liver derived in nonhuman primates. Arterioscler Thromb Vasc Biol. 2021;41:2851-2853. doi: 10.1161/atvbaha.121.316590
- Daugherty A, Sawada H, Sheppard MB, Lu HS. Angiotensinogen as a therapeutic target for cardiovascular and metabolic diseases. Arterioscler Thromb Vasc Biol. 2024;44:1021-1030. doi: 10.1161/ATVBAHA.124.318374
- Tao XR, Rong JB, Lu HS, et al. Angiotensinogen in hepatocytes contributes to Western diet-induced liver steatosis. J Lipid Res. 2019;60:1983-1995. doi: 10.1194/jlr.M093252
- Ye D, Wu C, Cai L, et al. Antisense oligonucleotides targeting hepatic angiotensinogen reduce atherosclerosis and liver steatosis in hypercholesterolemic mice. Glob Transl Med. 2023;2:288. doi: 10.36922/gtm.288
- Dutta S, Sengupta P. Men and mice: Relating their ages. Life Sci. 2016;152:244-248. doi: 10.1016/j.lfs.2015.10.025
- Temple JL, Cordero P, Li J, Nguyen V, Oben JA. A guide to non-alcoholic fatty liver disease in childhood and adolescence. Int J Mol Sci. 2016;17:947. doi: 10.3390/ijms17060947
- Boyraz M, Hatipoglu N, Sari E, et al. Non-alcoholic fatty liver disease in obese children and the relationship between metabolic syndrome criteria. Obes Res Clin Pract. 2014;8:e356-363. doi: 10.1016/j.orcp.2013.08.003
- Berardis S, Sokal E. Pediatric non-alcoholic fatty liver disease: An increasing public health issue. Eur J Pediatr. 2014;173:131-139. doi: 10.1007/s00431-013-2157-6
- Ratziu V, Bellentani S, Cortez-Pinto H, Day C, Marchesini G. A position statement on NAFLD/NASH based on the EASL 2009 special conference. J Hepatol. 2010;53:372-384. doi: 10.1016/j.jhep.2010.04.008
- Team RC. R: A language and environment for statistical computing. R Foundation for Statistical Computing; 2021. Available from : https://www.r-project.org [Last accessed on 2022 Dec 16].
- Robinson MD, McCarthy DJ, Smyth GK. edgeR: A Bioconductor package for differential expression analysis of digital gene expression data. Bioinformatics. 2010;26:139-140. doi: 10.1093/bioinformatics/btp616
- Carlson M. Genome wide annotation for Mouse. R package version 3.14.0; 2021. Available from: https://bioconductor.org/packages/release/data/annotation/html/org.Mm.eg. db.html [Last accessed on 2021 Jun 15].
- Wu T, Hu E, Xu S, et al. clusterProfiler 4.0: A universal enrichment tool for interpreting omics data. Innovation (Camb). 2021;2:100141. doi: 10.1016/j.xinn.2021.100141
- Yu G, Wang LG, Han Y, He QY. clusterProfiler: An R package for comparing biological themes among gene clusters. OMICS. 2012;16:284-287. doi: 10.1089/omi.2011.0118
- Tang D, Chen M, Huang X, et al. SRplot: A free online platform for data visualization and graphing. PLoS One. 2023;18:e0294236. doi: 10.1371/journal.pone.0294236
- Gaemers IC, Stallen JM, Kunne C, et al. Lipotoxicity and steatohepatitis in an overfed mouse model for non-alcoholic fatty liver disease. Biochim Biophys Acta. 2011;1812:447-458. doi: 10.1016/j.bbadis.2011.01.003
- Spooner MH, Garcia-Jaramillo M, Apperson KD, Lohr CV, Jump DB. Time course of western diet (WD) induced nonalcoholic steatohepatitis (NASH) in female and male Ldlr-/-mice. PLoS One. 2023;18:e0292432. doi: 10.1371/journal.pone.0292432
- Rinella ME, Neuschwander-Tetri BA, Siddiqui MS, et al. AASLD Practice Guidance on the clinical assessment and management of nonalcoholic fatty liver disease. Hepatology. 2023;77:1797-1835. doi: 10.1097/HEP.0000000000000323
- Milic S, Stimac D. Nonalcoholic fatty liver disease/ steatohepatitis: Epidemiology, pathogenesis, clinical presentation and treatment. Dig Dis. 2012;30:158-162. doi: 10.1159/000336669
- Basaranoglu M, Neuschwander-Tetri BA. Nonalcoholic fatty liver disease: Clinical features and pathogenesis. Gastroenterol Hepatol (N Y). 2006;2:282-291.
- Brunt EM, Tiniakos DG. Histopathology of nonalcoholic fatty liver disease. World J Gastroenterol. 2010;16:5286-5296. doi: 10.3748/wjg.v16.i42.5286
- Khoonsari M, Mohammad Hosseini Azar M, Ghavam R, et al. Clinical manifestations and diagnosis of nonalcoholic fatty liver disease. Iran J Pathol. 2017;12:99-105.
- Li X, Wang Z, Klaunig JE. Modulation of xenobiotic nuclear receptors in high-fat diet induced non-alcoholic fatty liver disease. Toxicology. 2018;410:199-213. doi: 10.1016/j.tox.2018.08.007
- Park WJ, Song JH, Kim GT, Park TS. Ceramide and sphingosine 1-phosphate in liver diseases. Mol Cells. 2020;43:419-430. doi: 10.14348/molcells.2020.0054
- Al-Rashed F, Arefanian H, Madhoun AA, et al. Neutral sphingomyelinase 2 inhibition limits hepatic steatosis and inflammation. Cells. 2024;13:463. doi: 10.3390/cells13050463
- Nikolova-Karakashian M, Karakashian A, Rutkute K. Role of neutral sphingomyelinases in aging and inflammation. Subcell Biochem. 2008;49:469-486. doi: 10.1007/978-1-4020-8831-5_18
- Summers SA. Ceramides: Nutrient signals that drive hepatosteatosis. J Lipid Atheroscler. 2020;9:50-65. doi: 10.12997/jla.2020.9.1.50
- El-Amouri S, Karakashian A, Bieberich E, Nikolova- Karakashian M. Regulated translocation of neutral sphingomyelinase-2 to the plasma membrane drives insulin resistance in steatotic hepatocytes. J Lipid Res. 2023;64:100435. doi: 10.1016/j.jlr.2023.100435
- Geilen CC, Wieder T, Orfanos CE. Ceramide signalling: regulatory role in cell proliferation, differentiation and apoptosis in human epidermis. Arch Dermatol Res. 1997;289:559-566. doi: 10.1007/s004030050240
- Hajduch E, Lachkar F, Ferre P, Foufelle F. Roles of ceramides in non-alcoholic fatty liver disease. J Clin Med. 2021;10:792. doi: 10.3390/jcm10040792
- Raichur S, Brunner B, Bielohuby M, et al. The role of C16:0 ceramide in the development of obesity and type 2 diabetes: CerS6 inhibition as a novel therapeutic approach. Mol Metab. 2019;21:36-50. doi: 10.1016/j.molmet.2018.12.008
- Saddoughi SA, Ogretmen B. Diverse functions of ceramide in cancer cell death and proliferation. Adv Cancer Res. 2013;117:37-58. doi: 10.1016/B978-0-12-394274-6.00002-9
- Rossi M, Duan S, Jeong YT, et al. Regulation of the CRL4(Cdt2) ubiquitin ligase and cell-cycle exit by the SCF(Fbxo11) ubiquitin ligase. Mol Cell. 2013;49:1159-1166. doi: 10.1016/j.molcel.2013.02.004
- Huh J, Piwnica-Worms H. CRL4(CDT2) targets CHK1 for PCNA-independent destruction. Mol Cell Biol. 2013;33: 213-226. doi: 10.1128/MCB.00847-12
- Liu Z, Yang G, Yi X, et al. Osteopontin regulates the growth and invasion of liver cancer cells via DTL. Oncol Lett. 2023;26:476. doi: 10.3892/ol.2023.14064
- Chen ZX, Mu MY, Yang G, et al. Hypoxia-induced DTL promotes the proliferation, metastasis, and sorafenib resistance of hepatocellular carcinoma through ubiquitin-mediated degradation of SLTM and subsequent Notch pathway activation. Cell Death Dis. 2024;15:734. doi: 10.1038/s41419-024-07089-4
- Dong R, Zhang D, Han B, et al. DTL is a novel downstream gene of E2F1 that promotes the progression of hepatocellular carcinoma. Curr Cancer Drug Targets. 2023;23:817-828. doi: 10.2174/1568009623666230511100246
- Borlado LR, Mendez J. CDC6: From DNA replication to cell cycle checkpoints and oncogenesis. Carcinogenesis. 2008;29:237-243. doi: 10.1093/carcin/bgm268
- Shi Y, Yan F, Wang F, Pan L. MiR-128-3p suppresses tumor proliferation and metastasis via targeting CDC6 in hepatocellular carcinoma cells. Tissue Cell. 2021;72:101534. doi: 10.1016/j.tice.2021.101534
- Uuskula-Reimand L, Wilson MD. Untangling the roles of TOP2A and TOP2B in transcription and cancer. Sci Adv. 2022;8:eadd4920. doi: 10.1126/sciadv.add4920
- Wang T, Lu J, Wang R, Cao W, Xu J. TOP2A promotes proliferation and metastasis of hepatocellular carcinoma regulated by miR-144-3p. J Cancer. 2022;13:589-601. doi: 10.7150/jca.64017
- Assy N, Minuk GY. Liver regeneration: methods for monitoring and their applications. J Hepatol. 1997; 26:945-952. doi: 10.1016/s0168-8278(97)80266-8
- Huang Z, Zhou P, Li S, Li K. Prediction of the Ki-67 marker index in hepatocellular carcinoma based on dynamic contrast-enhanced ultrasonography with sonazoid. Insights Imaging. 2022;13:199. doi: 10.1186/s13244-022-01320-6
- Trauner M, Fuchs CD. Novel therapeutic targets for cholestatic and fatty liver disease. Gut. 2022;71:194-209. doi: 10.1136/gutjnl-2021-324305
- Cast A, Kumbaji M, D’Souza A, et al. Liver proliferation is an essential driver of fibrosis in mouse models of nonalcoholic fatty liver disease. Hepatol Commun. 2019;3:1036-1049. doi: 10.1002/hep4.1381
- Sun X, Kaufman PD. Ki-67: More than a proliferation marker. Chromosoma. 2018;127:175-186. doi: 10.1007/s00412-018-0659-8
- Mrouj K, Andres-Sanchez N, Dubra G, et al. Ki-67 regulates global gene expression and promotes sequential stages of carcinogenesis. Proc Natl Acad Sci U S A. 2021;118:e2026507118. doi: 10.1073/pnas.2026507118
- Li Z, Wang R, Qiu C, et al. Role of DTL in hepatocellular carcinoma and its impact on the tumor microenvironment. Front Immunol. 2022;13:834606. doi: 10.3389/fimmu.2022.834606
- Chen YC, Chen IS, Huang GJ, et al. Targeting DTL induces cell cycle arrest and senescence and suppresses cell growth and colony formation through TPX2 inhibition in human hepatocellular carcinoma cells. Onco Targets Ther. 2018;11:1601-1616. doi: 10.2147/OTT.S147453
- Jia W, Liu X, Zhang Z. Role of TOP2A and CDC6 in liver cancer. Medicine (Baltimore). 2023;102:e35604. doi: 10.1097/MD.0000000000035604
- Wang K, Jiang X, Jiang Y, et al. EZH2-H3K27me3-mediated silencing of mir-139-5p inhibits cellular senescence in hepatocellular carcinoma by activating TOP2A. J Exp Clin Cancer Res. 2023;42:320. doi: 10.1186/s13046-023-02855-2
- Ramos-Santillan V, Oshi M, Nelson E, Endo I, Takabe K. High Ki67 gene expression is associated with aggressive phenotype in hepatocellular carcinoma. World J Oncol. 2024;15:257-267. doi: 10.14740/wjon1751
- Jin J, Valanejad L, Nguyen TP, et al. Activation of CDK4 triggers development of non-alcoholic fatty liver disease. Cell Rep. 2016;16:744-756. doi: 10.1016/j.celrep.2016.06.019
- Denechaud PD, Lopez-Mejia IC, Giralt A, et al. E2F1 mediates sustained lipogenesis and contributes to hepatic steatosis. J Clin Invest. 2016;126:137-150. doi: 10.1172/JCI81542
- Chen T, Sun Y, Ji P, Kopetz S, Zhang W. Topoisomerase IIalpha in chromosome instability and personalized cancer therapy. Oncogene. 2015;34:4019-4031. doi: 10.1038/onc.2014.332
- El Dika M, Dudka D, Kloc M, Kubiak JZ. CDC6 as a key inhibitory regulator of CDK1 activation dynamics and the timing of mitotic entry and progression. Biology (Basel). 2023;12:855. doi: 10.3390/biology12060855
- Fesler A, Zhang N, Ju J. The expanding regulatory universe of p53 in gastrointestinal cancer. F1000Res. 2016;5:756. doi: 10.12688/f1000research.8363.1
- Song B, Wang Y, Titmus MA, et al. Molecular mechanism of chemoresistance by miR-215 in osteosarcoma and colon cancer cells. Mol Cancer. 2010;9:96. doi: 10.1186/1476-4598-9-96
- Revill K, Wang T, Lachenmayer A, et al. Genome-wide methylation analysis and epigenetic unmasking identify tumor suppressor genes in hepatocellular carcinoma. Gastroenterology. 2013;145:1424-1435.e1421-1425. doi: 10.1053/j.gastro.2013.08.055
- Jovic D, Liang X, Zeng H, Lin L, Xu F, Luo Y. Single-cell RNA sequencing technologies and applications: A brief overview. Clin Transl Med. 2022;12:e694. doi: 10.1002/ctm2.694
- Tobar Leitao SA, Soares DDS, Carvas Junior N, Zimmer R, Ludwig NF, Andrades M. Study of anesthetics for euthanasia in rats and mice: A systematic review and meta-analysis on the impact upon biological outcomes (SAFE-RM). Life Sci. 2021;284:119916. doi: 10.1016/j.lfs.2021.119916