Antisense oligonucleotides targeting hepatic angiotensinogen reduce atherosclerosis and liver steatosis in hypercholesterolemic mice
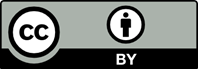
Hepatocyte-derived angiotensinogen (AGT) is the precursor of angiotensin II (AngII). We determined the effects of hepatocyte-specific (N-acetylgalactosamine-conjugated) antisense oligonucleotides targeting AGT (GalNAc AGT ASO) on AngII-mediated blood pressure (BP) regulation and atherosclerosis and compared its effects with losartan, an AngII type 1 (AT1) receptor blocker, in hypercholesterolemic mice. Eight-week-old male low-density lipoprotein (LDL) receptor deficient mice were administered vehicle or GalNAc AGT ASO (1, 2.5, or 5 mg/kg) subcutaneously beginning 2 weeks before the initiation of Western diet feeding. All mice were fed Western diet for 12 weeks. Their systolic BP was monitored by the tail-cuff technique, and the atherosclerotic lesion area was measured by an en face method. Although the effects of all 3 doses of GalNAc AGT ASO on plasma AGT concentrations were similar, GalNAc AGT ASO reduced BP and atherosclerotic lesion size in a dose-dependent manner. Subsequently, we compared the effects of GalNAc AGT ASO (5 mg/kg) with losartan (15 mg/kg/day). Compared to losartan, GalNAc AGT ASO led to more profound increases in plasma renin and reduction in BP but had similar effects on atherosclerosis. Remarkably, GalNAc AGT ASO also reduced liver steatosis, which was not observed in losartan-treated mice. In conclusion, the BP increase and atherosclerosis development in hypercholesterolemic mice are dependent on AngII generated from hepatic AGT. Deleting hepatic AGT improves diet-induced liver steatosis, and this occurs in an AT1 receptor-independent manner.
Lu H, Cassis LA, Vander Kooi CW, et al., 2016, Structure and functions of angiotensinogen. Hypertens Res, 39: 492–500. https://doi.org/10.1038/hr.2016.17
Wu C, Lu H, Cassis LA, et al., 2011, Molecular and pathophysiological features of angiotensinogen: A mini review. N Am J Med Sci (Boston), 4: 183–190. https://doi.org/10.7156/v4i4p183
Daugherty A, Rateri DL, Lu H, et al., 2004, Hypercholesterolemia stimulates angiotensin peptide synthesis and contributes to atherosclerosis through the AT1A receptor. Circulation, 110: 3849–3857. https://doi.org/10.1161/01.CIR.0000150540.54220.C4
Lu H, Rateri DL, Feldman DL, et al., 2008, Renin inhibition reduces hypercholesterolemia-induced atherosclerosis in mice. J Clin Invest, 118: 984–993. https://doi.org/10.1172/JCI32970
Lu H, Balakrishnan A, Howatt DA, et al., 2012, Comparative effects of different modes of renin angiotensin system inhibition on hypercholesterolaemia-induced atherosclerosis. Br J Pharmacol, 165: 2000–2008. https://doi.org/10.1111/j.1476-5381.2011.01712.x
Takata Y, Chu V, Collins AR, et al., 2005, Transcriptional repression of ATP-binding cassette transporter A1 gene in macrophages: A novel atherosclerotic effect of angiotensin II. Circ Res, 97: e88–e96. https://doi.org/10.1161/01.RES.0000190400.46267.7e
Fusco FB, Gomes DJ, Bispo KC, et al., 2017, Low-sodium diet induces atherogenesis regardless of lowering blood pressure in hypertensive hyperlipidemic mice. PLoS One, 12: e0177086. https://doi.org/10.1371/journal.pone.0177086
Lu H, Wu C, Howatt DA, et al., 2016, Angiotensinogen exerts effects independent of angiotensin II. Arterioscler Thromb Vasc Biol, 36: 256–265. https://doi.org/10.1161/ATVBAHA.115.306740
Dickson ME, Sigmund CD, 2006, Genetic basis of hypertension: Revisiting angiotensinogen. Hypertension, 48: 14–20. https://doi.org/10.1161/01.HYP.0000227932.13687.60
Yiannikouris F, Karounos M, Charnigo R, et al., 2012, Adipocyte-specific deficiency of angiotensinogen decreases plasma angiotensinogen concentration and systolic blood pressure in mice. Am J Physiol Regul Integr Comp Physiol, 302: R244–R251. https://doi.org/10.1152/ajpregu.00323.2011
Yiannikouris F, Gupte M, Putnam K, et al., 2012, Adipocyte deficiency of angiotensinogen prevents obesity-induced hypertension in male mice. Hypertension, 60: 1524–1530. https://doi.org/10.1161/HYPERTENSIONAHA.112.192690
Matsusaka T, Niimura F, Shimizu A, et al., 2012, Liver angiotensinogen is the primary source of renal angiotensin II. J Am Soc Nephrol, 23: 1181–1189. https://doi.org/10.1681/ASN.2011121159
Rong J, Tao X, Lin Y, et al., 2021, Loss of hepatic angiotensinogen attenuates sepsis-induced myocardial dysfunction. Circ Res, 129: 547–564. https://doi.org/10.1161/CIRCRESAHA.120.318075
Mullick AE, Yeh ST, Graham MJ, et al., 2017, Blood pressure lowering and safety improvements with liver angiotensinogen inhibition in models of hypertension and kidney injury. Hypertension, 70: 566–576. https://doi.org/10.1161/HYPERTENSIONAHA.117.09755
Daugherty A, Rateri D, Lu H, et al., 2009, Measuring blood pressure in mice using volume pressure recording, a tail-cuff method. J Vis Exp, 27: 1291. https://doi.org/10.3791/1291
Daugherty A, Tall AR, Daemen MJ, et al., 2017, Recommendation on design, execution, and reporting of animal atherosclerosis studies: A scientific statement from the American heart association. Arterioscler Thromb Vasc Biol, 37: e131–e157. https://doi.org/10.1161/ATV.0000000000000062
Chen H, Howatt DA, Franklin MK, et al., 2022, A mini-review on quantification of atherosclerosis in hypercholesterolemic mice. Glob Transl Med, 1: 72. https://doi.org/10.36922/gtm.v1i1.76
Kim S, Yang L, Kim S, et al., 2017, Targeting hepatic heparin-binding EGF-like growth factor (HB-EGF) induces anti-hyperlipidemia leading to reduction of angiotensin II-induced aneurysm development. PLoS One, 12: e0182566.https://doi.org/10.1371/journal.pone.0182566
Tao XR, Rong JB, Lu HS, et al., 2019, Angiotensinogen in hepatocytes contributes to Western diet-induced liver steatosis. J Lipid Res, 60: 1983–1995. https://doi.org/10.1194/jlr.M093252
Lu Z, Li Y, Li AJ, et al., 2022, Loss of GPR40 in LDL receptor-deficient mice exacerbates high-fat diet-induced hyperlipidemia and nonalcoholic steatohepatitis. PLoS One, 17: e0277251. https://doi.org/10.1371/journal.pone.0277251
Liu Y, Zhang Y, Zhu H, et al., 2022, Aucubin administration suppresses STING signaling and mitigated high-fat diet-induced atherosclerosis and steatohepatosis in LDL receptor deficient mice. Food Chem Toxicol, 169: 113422. https://doi.org/10.1016/j.fct.2022.113422
de Jong LM, Zhang Z, den Hartog Y, et al., 2022, PRMT3 inhibitor SGC707 reduces triglyceride levels and induces pruritus in Western-type diet-fed LDL receptor knockout mice. Sci Rep, 12: 483. https://doi.org/10.1038/s41598-021-04524-w
Lu H, Cassis LA, Daugherty A, 2007, Atherosclerosis and arterial blood pressure in mice. Curr Drug Targets, 8: 1181–1189. https://doi.org/10.2174/138945007782403829
Wu C, Xu Y, Lu H, et al., 2015, Cys18-Cys137 disulfide bond in mouse angiotensinogen does not affect AngII-dependent functions in vivo. Hypertension, 65: 800–805. https://doi.org/10.1161/HYPERTENSIONAHA.115.05166
Wu CH, Wu C, Howatt DA, et al., 2020, Two amino acids proximate to the renin cleavage site of human angiotensinogen do not affect blood pressure and atherosclerosis in mice. Arterioscler Thromb Vasc Biol, 40: 2108–2113. https://doi.org/10.1161/ATVBAHA.120.314048
Kukida M, Cai L, Ye D, et al., 2021, Renal angiotensinogen is predominantly liver derived in nonhuman primates. Arterioscler Thromb Vasc Biol, 41: 2851–2853. https://doi.org/10.1161/ATVBAHA.121.316590
Wang CH, Liu HM, Chang ZY, et al., 2021, Losartan prevents hepatic steatosis and macrophage polarization by inhibiting HIF-1α in a murine model of NAFLD. Int J Mol Sci, 22: 7841. https://doi.org/10.3390/ijms22157841
Stucchi P, Cano V, Ruiz-Gayo M, et al., 2009, Aliskiren reduces body-weight gain, adiposity and plasma leptin during diet-induced obesity. Br J Pharmacol, 158: 771–778. https://doi.org/10.1111/j.1476-5381.2009.00355.x
Premaratna SD, Manickam E, Begg DP, et al., 2012, Angiotensin-converting enzyme inhibition reverses diet-induced obesity, insulin resistance and inflammation in C57BL/6J mice. Int J Obes (Lond), 36: 233–243. https://doi.org/10.1038/ijo.2011.95
Cruz-López EO, Ren L, Uijl E, et al., 2023, Blood pressure-independent renoprotective effects of small interference RNA targeting liver angiotensinogen in experimental diabetes. Br J Pharmacol, 180: 80–93. https://doi.org/10.1111/bph.15955