Succinate metabolism in cardiovascular diseases
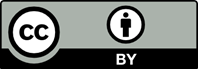
Cardiovascular disease (CVD) refers to a class of diseases related to the heart or blood vessels that have high global incidence. Succinate is generally considered an important intermediate product of the tricarboxylic acid cycle. Recent studies have shown that succinate is related to the pathophysiology of CVD, such as atherosclerosis, acute aortic dissection, hypertension, myocardial ischemia-reperfusion injury, and heart failure. It may represent a potential target or biomarker for CVD. It has been demonstrated that succinate not only participates in various energy metabolic pathways but also plays an important role in various pathophysiological activities as a signaling molecule. Given the significance of metabolism in CVD, it is important to focus on the metabolic regulation mechanism of succinate in CVD. This review outlines the latest evidence pointing to the potential role of succinate in CVD, along with its mechanisms, and updates the current understanding on the role of succinate in CVD. Further studies may focus on identifying succinate, its receptor, and its downstream signaling molecules as new targets for the prevention and treatment of CVD.
GBD 2016 Disease and Injury Incidence and Prevalence Collaborators. 2017, Global, regional, and national incidence, prevalence, and years lived with disability for 328 diseases and injuries for 195 countries, 1990-2016: A systematic analysis for the Global Burden of Disease Study 2016. Lancet, 390: 1211–1259. https://doi.org/10.1016/S0140-6736(17)32154-2
Ren J, Bi Y, Sowers JR, et al., 2021, Endoplasmic reticulum stress and unfolded protein response in cardiovascular diseases. Nat Rev Cardiol, 18: 499–521. https://doi.org/10.1038/s41569-021-00511-w
Nishida K, Otsu K, 2017, Inflammation and metabolic cardiomyopathy. Cardiovasc Res, 113: 389–398. https://doi.org/10.1093/cvr/cvx012
Lussey-Lepoutre C, Hollinshead KE, Ludwig C. et al., 2015, Loss of succinate dehydrogenase activity results in dependency on pyruvate carboxylation for cellular anabolism. Nat Commun, 6: 8784. https://doi.org/10.1038/ncomms9784
Burch JS, Marcero JR, Maschek JA, et al., 2018, Glutamine via alpha-ketoglutarate dehydrogenase provides succinyl- CoA for heme synthesis during erythropoiesis. Blood, 132: 987–998. https://doi.org/10.1182/blood-2018-01-829036
Winther S, Trauelsen M, Schwartz TW, 2021, Protective succinate-SUCNR1 metabolic stress signaling gone bad. Cell Metab, 33: 1276–1278. https://doi.org/10.1016/j.cmet.2021.06.009
Martinez-Reyes I, Chandel NS, 2020, Mitochondrial TCA cycle metabolites control physiology and disease. Nat Commun, 11: 102. https://doi.org/10.1038/s41467-019-13668-3
Markevich NI, Galimova MH, Markevich LN, 2020, Hysteresis and bistability in the succinate-CoQ reductase activity and reactive oxygen species production in the mitochondrial respiratory complex II. Redox Biol, 37: 101630. https://doi.org/10.1016/j.redox.2020.101630
Wagner M, Bertero E, Nickel A, et al., 2020, Selective NADH communication from alpha-ketoglutarate dehydrogenase to mitochondrial transhydrogenase prevents reactive oxygen species formation under reducing conditions in the heart. Basic Res Cardiol, 115: 53. https://doi.org/10.1007/s00395-020-0815-1
Liu PS, Wang H, Li X, et al., 2017, Alpha-ketoglutarate orchestrates macrophage activation through metabolic and epigenetic reprogramming. Nat Immunol, 18: 985–994. https://doi.org/10.1038/ni.3796
Alston CL, Davison JE, Meloni F, et al., 2012, Recessive germline SDHA and SDHB mutations causing leukodystrophy and isolated mitochondrial complex II deficiency. J Med Genet, 49: 569–577. https://doi.org/10.1136/jmedgenet-2012-101146
Acin-Perez R, Carrascoso I, Baixauli F, et al., 2014, ROS-triggered phosphorylation of complex II by Fgr kinase regulates cellular adaptation to fuel use. Cell Metab, 19: 1020–1033. https://doi.org/10.1016/j.cmet.2014.04.015
Kim SC, Sprung R, Chen Y, et al., 2006, Substrate and functional diversity of lysine acetylation revealed by a proteomics survey. Mol Cell, 23: 607–618. https://doi.org/10.1016/j.molcel.2006.06.026
Du J, Zhou Y, Su X, et al., 2011, Sirt5 is a NAD-dependent protein lysine demalonylase and desuccinylase. Science, 334: 806–809. https://doi.org/10.1126/science.1207861
Faith JJ, Ahern PP, Ridaura VK, et al., 2014, Identifying gut microbe-host phenotype relationships using combinatorial communities in gnotobiotic mice. Sci Transl Med, 6: 220ra211. https://doi.org/10.1126/scitranslmed.3008051
Macy JM, Ljungdahl LG, Gottschalk G, 1978, Pathway of succinate and propionate formation in Bacteroides fragilis. J Bacteriol, 134: 84–91. https://doi.org/10.1128/jb.134.1.84-91.1978
De Vadder F, Kovatcheva-Datchary P, Zitoun C, et al., 2016, Microbiota-produced succinate improves glucose homeostasis via intestinal gluconeogenesis. Cell Metab, 24: 151–157. https://doi.org/10.1016/j.cmet.2016.06.013
De Vadder F, Kovatcheva-Datchary P, Goncalves D, et al., 2014, Microbiota-generated metabolites promote metabolic benefits via gut-brain neural circuits. Cell, 156: 84–96. https://doi.org/10.1016/j.cell.2013.12.016
Watanabe Y, Nagai F, Morotomi M, 2012, Characterization of phascolarctobacterium succinatutens sp. nov., an asaccharolytic, succinate-utilizing bacterium isolated from human feces. Appl Environ Microbiol, 78: 511–518. https://doi.org/10.1128/AEM.06035-11
Fernandez-Veledo S, Vendrell J, 2019, Gut microbiota-derived succinate: Friend or foe in human metabolic diseases? Rev Endocr Metab Disord, 20: 439–447. https://doi.org/10.1007/s11154-019-09513-z
Connors J, Dawe N, Van Limbergen J, 2018, The role of succinate in the regulation of intestinal inflammation. Nutrients, 11: 25. https://doi.org/10.3390/nu11010025
Ariake K, Ohkusa T, Sakurazawa T, et al., 2000, Roles of mucosal bacteria and succinic acid in colitis caused by dextran sulfate sodium in mice. J Med Dent Sci, 47: 233–241.
Tannahill GM, Curtis AM, Adamik J, et al., 2013, Succinate is an inflammatory signal that induces IL-1beta through HIF-1alpha. Nature, 496: 238–242. https://doi.org/10.1038/nature11986
Chouchani ET, Pell VR, Gaude E, et al., 2014, Ischaemic accumulation of succinate controls reperfusion injury through mitochondrial ROS. Nature, 515: 431–435. https://doi.org/10.1038/nature13909
Mills E, O’Neill LA, 2014, Succinate: A metabolic signal in inflammation. Trends Cell Biol, 24: 313–320. https://doi.org/10.1016/j.tcb.2013.11.008
Reddy A, Bozi LH, Yaghi OK, et al., 2020, pH-gated succinate secretion regulates muscle remodeling in response to exercise. Cell, 183: 62–75 e17. https://doi.org/10.1016/j.cell.2020.08.039
An YA, Chen S, Deng Y, et al., 2021, The mitochondrial dicarboxylate carrier prevents hepatic lipotoxicity by inhibiting white adipocyte lipolysis. J Hepatol, 75: 387–399. https://doi.org/10.1016/j.jhep.2021.03.006
Prag HA, Gruszczyk AV, Huang MM, et al., 2021, Mechanism of succinate efflux upon reperfusion of the ischaemic heart. Cardiovasc Res, 117: 1188–1201. https://doi.org/10.1093/cvr/cvaa148
Bisbach CM, Hass DT, Thomas ED, et al., 2022, Monocarboxylate transporter 1 (MCT1) mediates succinate export in the retina. Invest Ophthalmol Vis Sci, 63: 1. https://doi.org/10.1167/iovs.63.4.1
He W, Miao FJ, Lin DC, et al., 2004, Citric acid cycle intermediates as ligands for orphan G-protein-coupled receptors. Nature, 429: 188–193. https://doi.org/10.1038/nature02488
Haffke M, Fehlmann D, Rummel G, et al., 2019, Structural basis of species-selective antagonist binding to the succinate receptor. Nature, 574: 581–585. https://doi.org/10.1038/s41586-019-1663-8
Macaulay IC, Tijssen MR, Thijssen-Timmer DC, et al., 2007, Comparative gene expression profiling of in vitro differentiated megakaryocytes and erythroblasts identifies novel activatory and inhibitory platelet membrane proteins. Blood, 109: 3260–3269. https://doi.org/10.1182/blood-2006-07-036269
Rubic T, Lametschwandtner G, Jost S, et al., 2008, Triggering the succinate receptor GPR91 on dendritic cells enhances immunity. Nat Immunol, 9: 1261–1269. https://doi.org/10.1038/ni.1657
Saraiva AL, Veras FP, Peres RS, et al., 2018, Succinate receptor deficiency attenuates arthritis by reducing dendritic cell traffic and expansion of Th17 cells in the lymph nodes. FASEB J, 32: 6550–6558. https://doi.org/10.1096/fj.201800285
Trauelsen M, Rexen Ulven E, Hjorth SA, et al., 2017, Receptor structure-based discovery of non-metabolite agonists for the succinate receptor GPR91. Mol Metab, 6: 1585–1596. https://doi.org/10.1016/j.molmet.2017.09.005
Littlewood-Evans A, Sarret S, Apfel V, et al., 2016, GPR91 senses extracellular succinate released from inflammatory macrophages and exacerbates rheumatoid arthritis. J Exp Med, 213: 1655–1662. https://doi.org/10.1084/jem.20160061
van Diepen JA, Robben JH, Hooiveld GJ, et al., 2017, SUCNR1-mediated chemotaxis of macrophages aggravates obesity-induced inflammation and diabetes. Diabetologia, 60: 1304–1313. https://doi.org/10.1007/s00125-017-4261-z
Harber KJ, de Goede KE, Verberk SG, et al., 2020, Succinate is an inflammation-induced immunoregulatory metabolite in macrophages. Metabolites, 10: 372. https://doi.org/10.3390/metabo10090372
Fernandez-Veledo S, Ceperuelo-Mallafre V, Vendrell J, 2021, Rethinking succinate: An unexpected hormone-like metabolite in energy homeostasis. Trends Endocrinol Metab, 32: 680–692. https://doi.org/10.1016/j.tem.2021.06.003
Xu J, Zheng Y, Zhao Y, et al., 2022, Succinate/IL-1beta signaling axis promotes the inflammatory progression of endothelial and exacerbates atherosclerosis. Front Immunol, 13: 817572. https://doi.org/10.3389/fimmu.2022.817572
Chen J, Zhang J, Wu J, et al., 2021, Low shear stress induced vascular endothelial cell pyroptosis by TET2/SDHB/ROS pathway. Free Radic Biol Med, 162: 582–591. https://doi.org/10.1016/j.freeradbiomed.2020.11.017
Wu P, Chen J, Chen J, et al., 2020, Trimethylamine N-oxide promotes apoE(-/-) mice atherosclerosis by inducing vascular endothelial cell pyroptosis via the SDHB/ROS pathway. J Cell Physiol, 235: 6582–6591. https://doi.org/10.1002/jcp.29518
Mills EL, Kelly B, Logan A, et al., 2016, Succinate dehydrogenase supports metabolic repurposing of mitochondria to drive inflammatory macrophages. Cell, 167: 457–470 e413. https://doi.org/10.1016/j.cell.2016.08.064
Ming-Chin Lee K, Achuthan AA, De Souza DP, et al., 2022, Type I interferon antagonism of the JMJD3-IRF4 pathway modulates macrophage activation and polarization. Cell Rep, 39: 110719. https://doi.org/10.1016/j.celrep.2022.110719
Wu JY, Huang TW, Hsieh YT, et al., 2020, Cancer-derived succinate promotes macrophage polarization and cancer metastasis via succinate receptor. Mol Cell, 77: 213–227 e215. https://doi.org/10.1016/j.molcel.2019.10.023
Sampson UK, Norman PE, Fowkes FG, et al., 2014, Global and regional burden of aortic dissection and aneurysms: Mortality trends in 21 world regions, 1990 to 2010. Glob Heart, 9: 171–180 e110. https://doi.org/10.1016/j.gheart.2013.12.010
Cui H, Chen Y, Li K, et al., 2021, Untargeted metabolomics identifies succinate as a biomarker and therapeutic target in aortic aneurysm and dissection. Eur Heart J, 42: 4373–4385. https://doi.org/10.1093/eurheartj/ehab605
Toma I, Kang JJ, Sipos A, et al., 2008, Succinate receptor GPR91 provides a direct link between high glucose levels and renin release in murine and rabbit kidney. J Clin Invest, 118: 2526–2534. https://doi.org/10.1172/JCI33293
Vargas SL, Toma I, Kang JJ, et al., 2009, Activation of the succinate receptor GPR91 in macula densa cells causes renin release. J Am Soc Nephrol, 20: 1002–1011. https://doi.org/10.1681/ASN.2008070740
Robben JH, Fenton RA, Vargas SL, et al., 2009, Localization of the succinate receptor in the distal nephron and its signaling in polarized MDCK cells. Kidney Int, 76: 1258–1267. https://doi.org/10.1038/ki.2009.360
Sadagopan N, Li W, Roberds SL, et al., 2007, Circulating succinate is elevated in rodent models of hypertension and metabolic disease. Am J Hypertens, 20: 1209–1215. https://doi.org/10.1016/j.amjhyper.2007.05.010
Khamaysi A, Anbtawee-Jomaa S, Fremder M, et al., 2019, Systemic succinate homeostasis and local succinate signaling affect blood pressure and modify risks for calcium oxalate lithogenesis. J Am Soc Nephrol, 30: 381–392. https://doi.org/10.1681/ASN.2018030277
Milliken AS, Nadtochiy SM, Brookes PS, 2022, Inhibiting succinate release worsens cardiac reperfusion injury by enhancing mitochondrial reactive oxygen species generation. J Am Heart Assoc, 11: e026135. https://doi.org/10.1161/JAHA.122.026135
Lu YT, Li LZ, Yang YL, et al., 2018, Succinate induces aberrant mitochondrial fission in cardiomyocytes through GPR91 signaling. Cell Death Dis, 9: 672. https://doi.org/10.1038/s41419-018-0708-5
Pell VR, Chouchani ET, Frezza C, et al., 2016, Succinate metabolism: A new therapeutic target for myocardial reperfusion injury. Cardiovasc Res, 111: 134–141. https://doi.org/10.1093/cvr/cvw100
Bae J, Salamon RJ, Brandt EB, et al., 2021, Malonate promotes adult cardiomyocyte proliferation and heart regeneration. Circulation, 143: 1973–1986. https://doi.org/10.1161/CIRCULATIONAHA.120.049952
Valls-Lacalle L, Barba I, Miro-Casas E, et al., 2018, Selective inhibition of succinate dehydrogenase in reperfused myocardium with intracoronary malonate reduces infarct size. Sci Rep, 8: 2442. https://doi.org/10.1038/s41598-018-20866-4
Xu J, Pan H, Xie X, et al., 2018, Inhibiting succinate dehydrogenase by dimethyl malonate alleviates brain damage in a rat model of cardiac arrest. Neuroscience, 393: 24–32. https://doi.org/10.1016/j.neuroscience.2018.09.041
Martin JL, Costa AS, Gruszczyk AV, et al., 2009, Succinate accumulation drives ischaemia-reperfusion injury during organ transplantation. Nat Metab, 1: 966–974. https://doi.org/10.1038/s42255-019-0115-y
Wyss RK, Mendez Carmona N, Arnold M, et al., 2021, Hypothermic, oxygenated perfusion (HOPE) provides cardioprotection via succinate oxidation prior to normothermic perfusion in a rat model of donation after circulatory death (DCD). Am J Transplant, 21: 1003–1011. https://doi.org/10.1111/ajt.16258
Kolwicz SC Jr., Purohit S, Tian R, 2013, Cardiac metabolism and its interactions with contraction, growth, and survival of cardiomyocytes. Circ Res, 113: 603–616. https://doi.org/10.1161/CIRCRESAHA.113.302095
Aguiar CJ, Rocha-Franco JA, Sousa PA, et al., 2014, Succinate causes pathological cardiomyocyte hypertrophy through GPR91 activation. Cell Commun Signal, 12: 78. https://doi.org/10.1186/s12964-014-0078-2
Yang L, Yu D, Mo R, et al., 2016, The succinate receptor GPR91 is involved in pressure overload-induced ventricular hypertrophy. PLoS One, 11: e0147597. https://doi.org/10.1371/journal.pone.0147597
Davili Z, Johar S, Hughes C, et al., 2007, Succinate dehydrogenase deficiency associated with dilated cardiomyopathy and ventricular noncompaction. Eur J Pediatr, 166: 867–870. https://doi.org/10.1007/s00431-006-0310-1
Aguiar CJ, Andrade VL, Gomes ER, et al., 2010, Succinate modulates Ca(2+) transient and cardiomyocyte viability through PKA-dependent pathway. Cell Calcium, 47: 37–46. https://doi.org/10.1016/j.ceca.2009.11.003
Banerjee P, Bandyopadhyay A, 2014, Cytosolic dynamics of annexin A6 trigger feedback regulation of hypertrophy via atrial natriuretic peptide in cardiomyocytes. J Biol Chem, 289: 5371–5385. https://doi.org/10.1074/jbc.M113.514810
Palomer X, Salvado L, Barroso E, et al., 2013, An overview of the crosstalk between inflammatory processes and metabolic dysregulation during diabetic cardiomyopathy. Int J Cardiol, 168: 3160–3172. https://doi.org/10.1016/j.ijcard.2013.07.150
Singh T, Khan H, Gamble DT, et al., 2022, Takotsubo syndrome: Pathophysiology, emerging concepts, and clinical implications. Circulation, 145: 1002–1019. https://doi.org/10.1161/CIRCULATIONAHA.121.055854
Keiran N, Ceperuelo-Mallafre V, Calvo E, et al., 2019, SUCNR1 controls an anti-inflammatory program in macrophages to regulate the metabolic response to obesity. Nat Immunol, 20: 581–592. https://doi.org/10.1038/s41590-019-0372-7
Ceperuelo-Mallafre V, Llaurado G, Keiran N, et al., 2019, Preoperative circulating succinate levels as a biomarker for diabetes remission after bariatric surgery. Diabetes Care, 42: 1956–1965. https://doi.org/10.2337/dc19-0114
Mills EL, Pierce KA, Jedrychowski MP, et al., 2018, Accumulation of succinate controls activation of adipose tissue thermogenesis. Nature, 560: 102–106. https://doi.org/10.1038/s41586-018-0353-2
Mills EL, Harmon C, Jedrychowski MP, et al., 2021, UCP1 governs liver extracellular succinate and inflammatory pathogenesis. Nat Metab, 3: 604–617. https://doi.org/10.1038/s42255-021-00389-5
Liu X, Chen Y, Zhao L, et al., 2022, Dietary succinate supplementation to maternal mice improves fetal brown adipose tissue development and thermogenesis of female offspring. J Nutr Biochem, 100: 108908. https://doi.org/10.1016/j.jnutbio.2021.108908
Liu K, Lin L, Li Q, et al., 2020, Scd1 controls de novo beige fat biogenesis through succinate-dependent regulation of mitochondrial complex II. Proc Natl Acad Sci U S A, 117: 2462–2472. https://doi.org/10.1073/pnas.1914553117
Regard JB, Sato IT, Coughlin SR, 2008, Anatomical profiling of G protein-coupled receptor expression. Cell, 135: 561–571. https://doi.org/10.1016/j.cell.2008.08.040
McCreath KJ, Espada S, Galvez BG, et al., 2015, Targeted disruption of the SUCNR1 metabolic receptor leads to dichotomous effects on obesity. Diabetes, 64: 1154–1167. https://doi.org/10.2337/db14-0346
Rexen Ulven E, Trauelsen M, Brvar M, et al., 2018, Structure-activity investigations and optimisations of non-metabolite agonists for the succinate receptor 1. Sci Rep, 8: 10010. https://doi.org/10.1038/s41598-018-28263-7
Geubelle P, Gilissen J, Dilly S, et al., 2017, Identification and pharmacological characterization of succinate receptor agonists. Br J Pharmacol, 174: 796–808. https://doi.org/10.1111/bph.13738
Bhuniya D, Umrani D, Dave B, et al., 2011, Discovery of a potent and selective small molecule hGPR91 antagonist. Bioorg Med Chem Lett, 21: 3596–3602. https://doi.org/10.1016/j.bmcl.2011.04.091
Sakai M, Sumiyoshi T, Aoyama T, et al., 2020, GPR91 antagonist and TGF-beta inhibitor suppressed collagen production of high glucose and succinate induced HSC activation. Biochem Biophys Res Commun, 530: 362–366. https://doi.org/10.1016/j.bbrc.2020.07.141
Le CT, Nguyen G, Park SY, et al., 2018, LY2405319, an analog of fibroblast growth factor 21 ameliorates alpha-smooth muscle actin production through inhibition of the succinate-G-protein couple receptor 91 (GPR91) pathway in mice. PLoS One, 13: e0192146. https://doi.org/10.1371/journal.pone.0192146
Nguyen G, Park SY, Le CT, et al., 2018, Metformin ameliorates activation of hepatic stellate cells and hepatic fibrosis by succinate and GPR91 inhibition. Biochem Biophys Res Commun, 495: 2649–2656. https://doi.org/10.1016/j.bbrc.2017.12.143
Zhang H, Zheng J, Lin J, et al., 2018, miR-758 mediates oxLDL-dependent vascular endothelial cell damage by suppressing the succinate receptor SUCNR1. Gene, 663: 1–8. https://doi.org/10.1016/j.gene.2018.04.029
Li T, Hu J, Gao F, et al., 2017, Transcription factors regulate GPR91-mediated expression of VEGF in hypoxia-induced retinopathy. Sci Rep, 7: 45807. https://doi.org/10.1038/srep45807
Osuna-Prieto FJ, Martinez-Tellez B, Ortiz-Alvarez L, et al., 2021, Elevated plasma succinate levels are linked to higher cardiovascular disease risk factors in young adults. Cardiovasc Diabetol, 20: 151. https://doi.org/10.1186/s12933-021-01333-3
Serena C, Ceperuelo-Mallafre V, Keiran N, et al., 2018, Elevated circulating levels of succinate in human obesity are linked to specific gut microbiota. ISME J, 12: 1642–1657. https://doi.org/10.1038/s41396-018-0068-2