Epigenetic perspective on atherosclerotic cardiovascular diseases: The holistic principle of systems biology and epigenetic reasoning
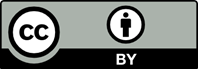
Atherosclerosis and coronary artery disease are the main causes of impairment and cardiac death, placing a significant burden on our health-care system. This review focuses on elucidating the involvement of various epigenetic mechanisms in the genesis and progression of cardiovascular diseases (CVDs), particularly chronic CVDs. The deregulation of epigenetic mechanisms plays a crucial role in the progression of CVDs, prompting exploration into novel preventive approaches. Advancements in molecular procedures, network-based approaches, and data analysis have identified new targets in CVDs, permitting the utilization of individualized epigenetic factors for personalized diagnosis and treatment. While promising for improving diagnostic and prognostic assessments, the clinical implementation of epigenetic biomarkers lags behind. Multicenter clinical documentation in a large sample population is crucial to confidently ascertain the clinical utility of specific epigenetic biomarkers. Of particular interest is the interplay between epigenetics and the conflict between the gene-based reductionist theory and the holistic principle of systems biology (SB). The holistic principle analyzes the structural organization and regulation of biological networks, influencing the genesis and progression of complex cardiac diseases like CVDs. This review emphasizes the complexity of CVDs, elucidates the interrelationship between disease networks and epigenetic mechanisms, and highlights the importance of the holistic principle of SB, coupled with artificial intelligence, in clarifying this interrelationship. The constant and uninterrupted epigenetic impact holds immense potential for advancing our understanding of disease progression and treatment across cells and tissues. Despite these advancements, the full integration of the epigenetic impact into medical practice remains incomplete, with limited utilization in clinical applications. Nevertheless, it is likely that in the near future, the epigenetic regulation of gene expression, with its lifelong and extended effects on health, will become an integral part of everyday clinical practice.
- Cox D, 2023, The Mystery of the Human Genome’s Dark Matter. United Kingdom: Future-BBC.
- Fasolo F, Di Gregoli K, Maegdefessel L, et al., 2019, Non-coding RNAs in cardiovascular cell biology and atherosclerosis. Cardiovasc Res, 115: 1732–1756. https://doi.org/10.1093/cvr/cvz203
- Rizzacasa B, Amati F, Romeo F, et al., 2019, Epigenetic modification in coronary atherosclerosis: JACC review topic of the week. J Am Coll Cardiol, 74: 1352–1365. https://doi.org/10.1016/j.jacc.2019.07.043
- Alghamdi BA, Aljohani IM, Alotaibi BG, et al., 2022, Studying epigenetics of cardiovascular diseases on chip guide. Cardiogenetics, 12: 218–234. https://doi.org/10.3390/cardiogenetics12030021
- Koch L, 2023, Spatial epigenomics in single cells. Nat Rev Genet, 24: 1. https://doi.org/10.1038/s41576-022-00552-y
- Kliegman RM, St Geme J, 2020, In Nelson Textbook of Pediatrics. 21st ed. Available from: https://eu.elsevierhealth.com [Last accessed on 2023 May 20].
- Napoli C, Coscioni E, de Nigris F, et al., 2021, Emergent expansion of clinical epigenetics in patients with cardiovascular diseases. Curr Opin Cardiol, 36: 295–300. https://doi.org/10.1097/HCO.0000000000000843
- Prasher D, Greenway SC, Singh RB, 2020, The impact of epigenetics on cardiovascular disease. Biochem Cell Biol, 98: 12–22. https://doi.org/10.1139/bcb-2019-0045
- van der Harst P, de Windt LJ, Chambers JC, 2017, Translational perspective on epigenetics in cardiovascular disease. J Am Coll Cardiol, 70: 590–606. https://doi.org/10.1016/j.jacc.2017.05.067
- Grimaldi V, Vietri MT, Schiano C, et al., 2015, Epigenetic reprogramming in atherosclerosis. Curr Atheroscler Rep, 17: 476. https://doi.org/10.1007/s11883-014-0476-3
- Perrino C, Barabási AL, Condorelli G, et al., 2017, Epigenomic and transcriptomic approaches in the post-genomic era: Path to novel targets for diagnosis and therapy of the ischaemic heart? Position Paper of the European Society of Cardiology Working Group on Cellular Biology of the Heart. Cardiovasc Res, 113: 725–736. https://doi.org/10.1093/cvr/cvx070
- Lourida KG, Louridas GE, 2022, Clinical phenotypes of cardiovascular and heart failure diseases can be reversed? The Holistic principle of systems biology in multifaceted heart diseases. Cardiogenetics, 12: 142–169. https://doi.org/10.3390/cardiogenetics12020015
- Schiano C, Benincasa G, Franzese M, et al., 2020, Epigenetic-sensitive pathways in personalized therapy of major cardiovascular diseases. Pharmacol Ther, 210: 107514. https://doi.org/10.1016/j.pharmthera.2020.107514
- Waddington CH, 2012, The epigenotype, 1942. Int J Epidemiol, 41: 10–13. https://doi.org/10.1093/ije/dyr184
- Russo VE, Martienssen RA, Riggs AD, 1996, Epigenetic Mechanisms of Gene Regulation. Cold Spring Harbor, New York: Laboratory Press.
- Jablonka E, Lamb MJ, 2002, The changing concept of epigenetics. Ann N Y Acad Sci, 981: 82–96. https://doi.org/10.1111/j.1749-6632.2002.tb04913.x
- Holliday R, 2006, Epigenetics: A historical overview. Epigenetics, 1: 76–80. https://doi.org/10.4161/epi.1.2.2762
- Arimondo PB, Barberousse A, Pontarottic G, 2019, The many faces of epigenetics Oxford, December 2017. Epigenetics, 14: 623–631. https://doi.org/10.1080/15592294.2019.1595298
- Nicolosi G, Ruivenkamp G, 2012, The epigenetic turn. Some notes about the epistemological change of perspective in biosciences. Med Health Care Philos, 15: 309–319. https://doi.org/10.1007/s11019-011-9342-z
- Cavalli G, Heard E, 2019, Advances in epigenetics link genetics to the environment and disease. Nature, 571: 489–499. https://doi.org/10.1038/s41586-019-1411-0
- Shi Y, Zhang H, Huang S, et al, 2022, Epigenetic regulation in cardiovascular disease: Mechanisms and advances in clinical trials. Signal Transduct Target Ther, 7: 200. https://doi.org/10.1038/s41392-022-01055-2
- Chiapperino L, 2018, Epigenetics: Ethics, politics, biosociality. Br Med Bull, 128: 49–60. https://doi.org/10.1093/bmb/ldy033
- Fox Keller E, 2010, The Mirage of a Space between Nature and Nurture. Durham and London, UK: Duke University Press.
- Santaló J, Berdasco M, 2022, Ethical implications of epigenetics in the era of personalized medicine. Clin Epigenetics, 14: 44. https://doi.org/10.1186/s13148-022-01263-1
- Dupras C, Saulnier KM, Joly Y, 2019, Epigenetics, ethics, law and society: A multidisciplinary review of descriptive, instrumental, dialectical and reflexive analyses. Soc Stud Sci, 49: 785–810. https://doi.org/10.1177/0306312719866007
- Bunnik EM, Timmers M, Bolt IL, 2020, Ethical issues in research and development of epigenome-wide technologies. Epigenet Insights, 13: 2516865720913253. https://doi.org/10.1177/2516865720913253
- Deichmann U, 2020, The social construction of the social epigenome and the larger biological context. Epigenetics Chromatin, 13: 37. https://doi.org/10.1186/s13072-020-00360-w
- Federoff HJ, Gostin LO, 2009, Evolving from reductionism to holism: Is there a future for systems medicine? JAMA, 302: 994–996. https://doi.org/10.1001/jama.2009.1264
- Lourida KG, Louridas GE, 2021, Constraints in clinical cardiology and personalized medicine: Interrelated concepts in clinical cardiology. Cardiogenetics, 11: 50–67. https://doi.org/10.3390/cardiogenetics11020007
- Boorse C, 1997, A rebuttal on health. In: Humber JM, Almeder JF, (Eds). What is Disease? Totowa, NJ, USA: Humana Press, p1–134.
- Louridas GE, 2019, The limited role of genetics explaining the atherosclerotic process and coronary artery disease and the holistic perspective of systems biology. Hellenic J Cardiol, 60: 141–142. https://doi.org/10.1016/j.hjc.2018.06.002
- Louridas GE, Lourida KG, 2017, The complex cardiac atherosclerotic disorder: The elusive role of genetics and the new consensus of systems biology approach. J Atamis, 2: 10-17.
- Gilbert SF, 2000, Developmental Biology. A New Evolutionary Synthesis. 6th ed. Sunderland, MA: Sinauer Associates. Available from: https://www.ncbi.nlm.nih.gov/ books/NBK10128 [Last accessed on 2023 May 20].
- Noble D, 2013, Physiology is rocking the foundations of evolutionary biology. Exp Physiol, 98: 1235–1243. https://doi.org/10.1113/expphysiol.2012.071134
- Noble D, 2015, Evolution beyond neo-Darwinism: A new conceptual framework. J Exp Biol, 218: 7–13. https://doi.org/10.1242/jeb.106310. Erratum in: J Exp Biol, 218: 1273.
- Noble D, 2008, Genes and causation. Philos Trans A Math Phys Eng Sci, 366: 3001–3015. https://doi.org/10.1098/rsta.2008.0086
- Boi L, Lobo C, 2022, Geometry and phenomenology of the living: Limits and possibilities of mathematization, complexity and individuation in biological sciences. Theory Biosci, 141: 53–58. https://doi.org/10.1007/s12064-022-003
- Robinson SW, Fernandes M, Husi H, 2014, Current advances in systems and integrative biology. Computat Struct Biotechnol J, 11: 35–46. https://doi.org/10.1016/j.csbj.2014.08.007
- Kellenberger E, 2004, The evolution of molecular biology. EMBO Rep, 5: 546–549. https://doi.org/10.1038/sj.embor.7400180
- Fiandaca MS, Mapstone M, Connors E, et al., 2017, Systems healthcare: A holistic paradigm for tomorrow. BMC Syst Biol, 11: 142. https://doi.org/10.1186/s12918-017-0521-2
- Boogerd FC, Bruggeman FJ, Hofmeyr JHS, eds, et al., 2007, Systems Biology, Philosophical Foundations. Amsterdam, The Netherlands: Elsevier BV.
- Naylor S, Chen JY, 2010, Unraveling human complexity and disease with systems biology and personalized medicine. Pers Med, 7: 275–289. https://doi.org/10.2217/pme.10.16
- Ellis GFR, Noble D, O’Connor T, 2012, Top-down causation: An integrating theme within and across the sciences. Interface Focus, 2: 1–3. https://doi.org/10.1098/rsfs.2011.0110
- Kitano H, 2002, Systems biology: A brief overview. Science, 295: 1662–1664. https://doi.org/10.1126/science.1069492
- Noble D, 2021, The principle of biological relativity: Origins and current status. In: Voosholz J, Gabriel M, (eds). Top- Down Causation and Emergence. Synthese Library. Vol. 439. Cham: Springer. https://doi.org/10.1007/978-3-030-71899-2_6
- Delker RK, Mann RS, 2017, From reductionism to holism: Toward a more complete view of development through genome engineering. Adv Exp Med Biol, 1016: 45–74. https://doi.org/10.1007/978-3-319-63904-8_3
- Sarno F, Benincasa G, List M, et al., 2021, Clinical epigenetics settings for cancer and cardiovascular diseases: Real-life applications of network medicine at the bedside. Clin Epigenetics, 13: 66. https://doi.org/10.1186/s13148-021-01047-z
- Soler-Botija C, Gálvez-Montón C, Bayés-Genís A, 2019, Epigenetic biomarkers in cardiovascular diseases. Front Genet, 10: 950. https://doi.org/10.3389/fgene.2019.00950
- Fouse SD, Shen Y, Pellegrini M, et al., 2008, Promoter CpG methylation contributes to ES cell gene regulation in parallel with Oct4/Nanog, PcG complex, and histone H3 K4/K27 trimethylation. Cell Stem Cell, 2: 160–169. https://doi.org/10.1016/j.stem.2007.12.011
- Kouzarides T, 2007, Chromatin modifications and their function. Cell, 128: 693–705. https://doi.org/10.1016/j.cell.2007.02.005
- Baccarelli A, Rienstra M, Benjamin EJ, 2010, Cardiovascular epigenetics: Basic concepts and results from animal and human studies. Circ Cardiovasc Genet, 3: 567–573. https://doi.org/10.1161/CIRCGENETICS.110.958744
- Cardona-Monzonís A, Beltrán-García J, Ibañez-Cabellos JS, et al, 2018, Epigenetic biomarkers in cardiovascular disease. J Lab Precis Med, 3: 24. https://doi.org/10.21037/jlpm.2018.02.04
- Xu S, Pelisek J, Jin ZG, 2018, Atherosclerosis is an epigenetic disease. Trends Endocrinol Metab, 29: 739–742. https://doi.org/10.1016/j.tem.2018.04.007
- Bekkering S, van den Munckhof I, Nielen T, et al., 2016, Innate immune cell activation and epigenetic remodeling in symptomatic and asymptomatic atherosclerosis in humans in vivo. Atherosclerosis, 254: 228–236. https://doi.org/10.1016/j.atherosclerosis.2016.10.019
- Valencia-Morales MDP, Zaina S, Heyn H, et al., 2015, The DNA methylation drift of the atherosclerotic aorta increases with lesion progression. BMC Med Genomics, 8: 7. https://doi.org/10.1186/s12920-015-0085-1
- Cazaly E, Saad J, Wang W, et al., 2019, Making sense of the epigenome using data integration approaches. Front Pharmacol, 10: 126. https://doi.org/10.3389/fphar.2019.00126
- Romano G, Veneziano D, Nigita G, et al., 2018, RNA methylation in ncRNA: Classes, detection, and molecular associations. Front Genet, 9: 243. https://doi.org/10.3389/fgene.2018.00243
- Pidsley R, Zotenko E, Peters TJ, et al., 2016, Critical evaluation of the illumina methylation EPIC beadchip microarray for whole-genome DNA methylation profiling. Genome Biol, 17: 208. https://doi.org/10.1186/s13059-016-1066-1
- Kumar A, Kumar S, Vikram A, et al., 2013, Histone and DNA methylation-mediated epigenetic downregulation of endothelial Kruppel-like factor 2 by low-density lipoprotein cholesterol. Arterioscler Thromb Vasc Biol, 33: 1936–1942. https://doi.org/10.1161/ATVBAHA.113.301765
- Jiang YZ, Jiménez JM, Ou K, et al., 2014, Hemodynamic disturbed flow induces differential DNA methylation of endothelial Kruppel-like factor 4 promoter in vitro and in vivo. Circ Res, 115: 32–43. https://doi.org/10.1161/CIRCRESAHA.115.303883
- Jiang D, Sun M, You L, et al., 2019, DNA methylation and hydroxymethylation are associated with the degree of coronary atherosclerosis in elderly patients with coronary heart disease. Life Sci, 224: 241–248. https://doi.org/10.1016/j.lfs.2019.03.021
- Zhang Y, Zeng C, 2016, Role of DNA methylation in cardiovascular diseases. Clin Exp Hypertens, 38: 261–267. https://doi.org/10.3109/10641963.2015.1107087
- Zhang Y, Jia Z, Zhou Q, et al., 2022, A bibliometric analysis of DNA methylation in cardiovascular diseases from 2001 to 2021. Medicine (Baltimore), 101: e30029. https://doi.org/10.1097/MD.0000000000030029
- Palou-Márquez G, Subirana I, Nonell L, et al., 2021, DNA methylation and gene expression integration in cardiovascular disease. Clin Epigenetics, 13: 75. https://doi.org/10.1186/s13148-021-01064-y
- Fernández-Sanlés A, Sayols-Baixeras S, Subirana I, et al., 2021, DNA methylation biomarkers of myocardial infarction and cardiovascular disease. Clin Epigenetics, 13: 86. https://doi.org/10.1186/s13148-021-01078-6
- ENCODE Project Consortium, 2012, An integrated encyclopedia of DNA elements in the human genome. Nature, 489: 57–74. https://doi.org/10.1038/nature11247
- Janssen KA, Sidoli S, Garcia BA, 2017, Recent achievements in characterizing the histone code and approaches to integrating epigenomics and systems biology. Methods Enzymol, 586: 359–378. https://doi.org/10.1016/bs.mie.2016.10.021
- Jun Q, Youhong L, Yuan Z, et al., 2022, Histone modification of endothelial-mesenchymal transition in cardiovascular diseases. Front Cardiovasc Med, 9: 1022988. https://doi.org/10.3389/fcvm.2022.1022988
- Lecce L, Xu Y, V’Gangula B, et al., 2021, Histone deacetylase 9 promotes endothelial-mesenchymal transition and an unfavorable atherosclerotic plaque phenotype. J Clin Invest, 131: e131178. https://doi.org/10.1172/JCI131178
- Zampetaki A, Zeng L, Margariti A, et al., 2010, Histone deacetylase 3 is critical in endothelial survival and atherosclerosis development in response to disturbed flow. Circulation, 121: 132–142. https://doi.org/10.1161/CIRCULATIONAHA.109.890491
- Findeisen HM, Gizard F, Zhao Y, et al., 2011, Epigenetic regulation of vascular smooth muscle cell proliferation and neointima formation by histone deacetylase inhibition. Arterioscler Thromb Vasc Biol, 31: 851–860. https://doi.org/10.1161/ATVBAHA.110.221952
- Greißel A, Culmes M, Burgkart R, et al., 2016, Histone acetylation and methylation significantly change with severity of atherosclerosis in human carotid plaques. Cardiovasc Pathol, 25: 79–86. https://doi.org/10.1016/j.carpath.2015.11.001
- Yang Y, Luan Y, Yuan RX, et al., 2021, Histone methylation related therapeutic challenge in cardiovascular diseases. Front Cardiovasc Med, 8: 710053. https://doi.org/10.3389/fcvm.2021.710053
- Santulli G, 2023, Non-coding RNAs in clinical practice: From biomarkers to therapeutic tools. J Pharmacol Exp Ther, 384: 225–226. https://doi.org/10.1124/jpet.122.001457
- Valencia-Sanchez MA, Liu J, Hannon GJ, et al., 2006, Control of translation and mRNA degradation by miRNAs and siRNAs. Genes Dev, 20: 515–524. https://doi.org/10.1101/gad.1399806
- Ferreira HJ, Esteller M, 2018, Non-coding RNAs, epigenetics, and cancer: Tying it all together. Cancer Metastasis Rev, 37: 55–73. https://doi.org/10.1007/s10555-017-9715-8
- Macvanin MT, Zafirovic S, Obradovic M, et al., 2023, Editorial: Non-coding RNA in diabetes and cardiovascular diseases. Front Endocrinol (Lausanne), 14: 1149857. https://doi.org/10.3389/fendo.2023.1149857
- Kristensen LS, Wojdacz TK, Thestrup BB, et al., 2009, Quality assessment of DNA derived from up to 30 years old formalin fixed paraffin embedded (FFPE) tissue for PCR-based methylation analysis using SMART-MSP and MS-HRM. BMC Cancer, 9: 453. https://doi.org/10.1186/1471-2407-9-453
- He L, Hannon G, 2004, MicroRNAs: Small RNAs with a big role in gene regulation. Nat Rev Genet, 5: 522–531. https://doi.org/10.1038/nrg1379
- Akhtar MM, Micolucci L, Islam MS, et al., 2016, Bioinformatic tools for microRNA dissection. Nucleic Acids Res, 44: 24–44. https://doi.org/10.1093/nar/gkv1221
- Treiber T, Treiber N, Meister G, 2019, Regulation of microRNA biogenesis and its crosstalk with other cellular pathways. Nat Rev Mol Cell Biol, 20: 5–20. https://doi.org/10.1038/s41580-018-0059-1. Erratum in: Nat Rev Mol Cell Biol, 2018, 19: 808. Erratum in: Nat Rev Mol Cell Biol, 2019, 20: 321.
- Bartel DP, 2018, Metazoan microRNAs. Cell, 173: 20–51. https://doi.org/10.1016/j.cell.2018.03.006
- Laggerbauer B, Engelhardt S, 2022, MicroRNAs as therapeutic targets in cardiovascular disease. J Clin Invest, 132: e159179. https://doi.org/10.1172/JCI159179
- Kozomara A, Birgaoanu M, Griffiths-Jones S, 2019, miRBase: From microRNA sequences to function. Nucleic Acids Res, 47: D155–D162. https://doi.org/10.1093/nar/gky1141
- Kim K, Baek SC, Lee YY, et al., 2021, A quantitative map of human primary microRNA processing sites. Mol Cell, 81: 3422–3439.e11. https://doi.org/10.1016/j.molcel.2021.07.002
- Fernández-Hernando C, Baldán A, 2013, MicroRNAs and cardiovascular disease. Curr Genet Med Rep, 1: 30–38. https://doi.org/10.1007/s40142-013-0008-4
- Bartel DP, 2004, MicroRNAs: Genomics, biogenesis, mechanism, and function. Cell, 116: 281–297. https://doi.org/10.1016/s0092-8674(04)00045-5
- Demirtas D, Demirtas AO, Biskin A, et al., 2018, Micro- RNA in health and disease. Int J Cardiol Res, 5: 116–123. https://doi.org/10.19070/2470-4563-1800020
- Kong D, Duan Y, Wang J, et al., 2022, A functional polymorphism of microRNA-143 is associated with the risk of type 2 diabetes mellitus in the northern Chinese Han population. Front Endocrinol (Lausanne), 13: 994953. https://doi.org/10.3389/fendo.2022.994953
- Zheng B, Xi Z, Liu R, et al., 2018, The function of microRNAs in B-cell development, lymphoma, and their potential in clinical practice. Front Immunol, 9: 936. https://doi.org/10.3389/fimmu.2018.00936
- Raitoharju E, Oksala N, Lehtimäki T, 2013, MicroRNAs in the atherosclerotic plaque. Clin Chem, 59: 1708–1721. https://doi.org/10.1373/clinchem.2013.2049170
- de Yébenes VG, Briones AM, Martos-Folgado I, et al., 2020, Aging-associated miR-217 aggravates atherosclerosis and promotes cardiovascular dysfunction. Arterioscler Thromb Vasc Biol, 40: 2408–2424. https://doi.org/10.1161/ATVBAHA.120.314333
- Tao J, Xia L, Cai Z, et al., 2021, Interaction Between microRNA and DNA methylation in atherosclerosis. DNA Cell Biol, 40: 101–115. https://doi.org/10.1089/dna.2020.6138
- Yang X, Chen Y, Feng C, et al., 2018, MicroRNA-216a induces endothelial senescence and inflammation via Smad3/IκBα pathway. J Cell Mol Med, 22: 2739–2749. https://doi.org/10.1111/jcmm.13567
- Chen J, Xu L, Hu Q, et al., 2015, MiR-17-5p as circulating biomarkers for the severity of coronary atherosclerosis in coronary artery disease. Int J Cardiol, 197: 123–124. https://doi.org/10.1016/j.ijcard.2015.06.037
- Li S, Lee C, Song J, et al., 2017, Circulating microRNAs as potential biomarkers for coronary plaque rupture. Oncotarget, 8: 48145–48156. https://doi.org/10.18632/oncotarget.18308
- Mao Z, Wu F, Shan Y, 2018, Identification of key genes and miRNAs associated with carotid atherosclerosis based on mRNA-seq data. Medicine (Baltimore), 97: e9832. https://doi.org/10.1097/MD.0000000000009832
- Reddy S, Hu DQ, Zhao M, et al., 2017, miR-21 is associated with fibrosis and right ventricular failure. JCI Insight, 2: e91625. https://doi.org/10.1172/jci.insight.91625
- Petrovic N, Ergun S, 2018, miRNAs as potential treatment targets and treatment options in cancer. Mol Diagn Ther, 22: 157–168. https://doi.org/10.1007/s40291-017-0314-8
- Neppl RL, Wang DZ, 2014, The myriad essential roles of microRNAs in cardiovascular homeostasis and disease. Genes Dis, 1: 18–39. https://doi.org/10.1016/j.gendis.2014.06.003
- Wronska A, Kurkowska-Jastrzebska I, Santulli G, 2015, Application of microRNAs in diagnosis and treatment of cardiovascular disease. Acta Physiol (Oxf), 213: 60–83. https://doi.org/10.1111/apha.12416
- Wronska A, 2023, The role of microRNA in the development, diagnosis, and treatment of cardiovascular disease: Recent developments. J Pharmacol Exp Ther, 384: 123–132. https://doi.org/10.1124/jpet.121.001152
- Griesemer D, Xue JR, Reilly SK, et al., 2021, Genome-wide functional screen of 3’UTR variants uncovers causal variants for human disease and evolution. Cell, 184: 5247–5260.e19. https://doi.org/10.1016/j.cell.2021.08.025
- Maries L, Marian C, Sosdean R, et al., 2021, MicroRNAs-the heart of post-myocardial infarction remodeling. Diagnostics (Basel), 11: 1675. https://doi.org/10.3390/diagnostics11091675
- de Gonzalo-Calvo D, Cenarro A, Civeira F, et al., 2016, microRNA expression profile in human coronary smooth muscle cell-derived microparticles is a source of biomarkers. Clin Investig Arterioscler, 28: 167–177. https://doi.org/10.1016/j.arteri.2016.05.005
- Vaskova E, Ikeda G, Tada Y, et al., 2020, Sacubitril/valsartan improves cardiac function and decreases myocardial fibrosis via downregulation of exosomal miR-181a in a rodent chronic myocardial infarction model. J Am Heart Assoc, 9: e015640. https://doi.org/10.1161/JAHA.119.015640
- Li J, Cai SX, He Q, et al., 2018, Intravenous miR-144 reduces left ventricular remodeling after myocardial infarction. Basic Res Cardiol, 113: 36. https://doi.org/10.1007/s00395-018-0694-x
- Gao F, Kataoka M, Liu N, et al., 2019, Therapeutic role of miR-19a/19b in cardiac regeneration and protection from myocardial infarction. Nat Commun, 10: 1802. https://doi.org/10.1038/s41467-019-09530-1
- Gabisonia K, Prosdocimo G, Aquaro GD, et al., 2019, MicroRNA therapy stimulates uncontrolled cardiac repair after myocardial infarction in pigs. Nature, 569: 418–422. https://doi.org/10.1038/s41586-019-1191-6
- Roberts TC, Langer R, Wood MJA, 2020, Advances in oligonucleotide drug delivery. Nat Rev Drug Discov, 19: 673–694. https://doi.org/10.1038/s41573-020-0075-7
- Dasgupta I, Chatterjee A, 2021, Recent advances in miRNA delivery systems. Methods Protoc, 4: 10. https://doi.org/10.3390/mps4010010
- Hong T, Wei Y, Xue X, et al., 2020, A novel anti-coagulative nanocomplex in delivering miRNA-1 inhibitor against microvascular obstruction of myocardial infarction. Adv Healthc Mater, 9: e1901783. https://doi.org/10.1002/adhm.201901783
- Bejerano T, Etzion S, Elyagon S, et al., 2018, Nanoparticle delivery of miRNA-21 mimic to cardiac macrophages improves myocardial remodeling after myocardial infarction. Nano Lett, 18: 5885–5891. https://doi.org/10.1021/acs.nanolett.8b02578
- Feng YH, Tsao CJ, 2016, Emerging role of microRNA-21 in cancer. Biomed Rep, 5: 395–402. https://doi.org/10.3892/br.2016.747
- Bielska A, Niemira M, Bauer W, et al., 2022, Serum miRNA profile in diabetic patients with ischemic heart disease as a promising non-invasive biomarker. Front Endocrinol (Lausanne), 13: 888948. https://doi.org/10.3389/fendo.2022.888948
- Kilikevicius A, Meister G, Corey DR, 2002, Reexamining assumptions about miRNA-guided gene silencing. Nucleic Acids Res, 50: 617–634. https://doi.org/10.1093/nar/gkab1256
- Quinn JJ, Chang HY, 2016, Unique features of long non-coding RNA biogenesis and function. Nat Rev Genet, 17: 47–62. https://doi.org/10.1038/nrg.2015.10
- Wei JW, Huang K, Yang C, et al., 2017, Non-coding RNAs as regulators in epigenetics (Review). Oncol Rep, 37: 3–9. https://doi.org/10.3892/or.2016.5236
- Fasolo F, Jin H, Winski G, et al., 2021, Long noncoding RNA MIAT controls advanced atherosclerotic lesion formation and plaque destabilization. Circulation, 144: 1567–1583. https://doi.org/10.1161/CIRCULATIONAHA.120.052023
- Devaux Y, Zangrando J, Schroen B, et al., 2015, Long noncoding RNAs in cardiac development and ageing. Nat Rev Cardiol, 12: 415–425. https://doi.org/10.1038/nrcardio.2015.55
- Rezaee M, Masihipour N, Milasi YE, et al., 2023, New insights into the long non-coding RNAs dependent modulation of heart failure and cardiac hypertrophy: From molecular function to diagnosis and treatment. Curr Med Chem, 31: 1404–1426. https://doi.org/10.2174/0929867330666230306143351
- Wang Z, Zhang M, Fu Y, 2023, Downregulated circulating long non-coding RNA GAS6-AS1 screens and predicts acute myocardial infarction. Anatol J Cardiol, 27: 167–172. https://doi.org/10.14744/AnatolJCardiol.2022.2496
- Shi H, Nguyen T, Zhao Q, et al., 2023, Discovery of transacting long noncoding RNAs that regulate smooth muscle cell phenotype. Circ Res, 132: 795–811. https://doi.org/10.1161/CIRCRESAHA.122.321960
- Xun M, Zhang J, Wu M, et al., 2023, Long non-coding RNAs: The growth controller of vascular smooth muscle cells in cardiovascular diseases. Int J Biochem Cell Biol, 157: 106392. https://doi.org/10.1016/j.biocel.2023.106392
- Jakubik D, Fitas A, Eyileten C, et al., 2021, MicroRNAs and long non-coding RNAs in the pathophysiological processes of diabetic cardiomyopathy: Emerging biomarkers and potential therapeutics. Cardiovasc Diabetol, 20: 55. https://doi.org/10.1186/s12933-021-01245-2
- Li C, Wang D, Jiang Z, et al., 2022, Non-coding RNAs in diabetes mellitus and diabetic cardiovascular disease. Front Endocrinol (Lausanne), 13: 961802. https://doi.org/10.3389/fendo.2022.961802
- Yang T, Long T, Du T, et al., 20212, Circle the cardiac remodeling with circRNAs. Front Cardiovasc Med, 8: 702586. https://doi.org/10.3389/fcvm.2021.702586
- Qu S, Zhong Y, Shang R, et al., 2017, The emerging landscape of circular RNA in life processes. RNA Biol, 14: 992–999. https://doi.org/10.1080/15476286.2016.1220473
- Wilusz JE, 2018, A 360° view of circular RNAs: From biogenesis to functions. Wiley Interdiscip Rev RNA, 9: e1478. https://doi.org/10.1002/wrna.1478
- Wen G, Zhou T, Gu W, 2021, The potential of using blood circular RNA as liquid biopsy biomarker for human diseases. Protein Cell, 12: 911–946. https://doi.org/10.1007/s13238-020-00799-3
- Wen C, Li B, Nie L, et al., 2022, Emerging roles of extracellular vesicle-delivered circular RNAs in atherosclerosis. Front Cell Dev Biol, 10: 804247. https://doi.org/10.3389/fcell.2022.804247
- Ward Z, Pearson J, Schmeier S, et al., 2021, Insights into circular RNAs: their biogenesis, detection, and emerging role in cardiovascular disease. RNA Biol, 18: 2055–2072. https://doi.org/10.1080/15476286.2021.1891393
- Yu Z, Huang Q, Zhang Q, et al., 2021, CircRNAs open a new era in the study of cardiovascular disease (Review). Int J Mol Med, 47: 49–64. https://doi.org/10.3892/ijmm.2020.4792
- Holdt LM, Kohlmaier A, Teupser D, 2018, Molecular functions and specific roles of circRNAs in the cardiovascular system. Noncoding RNA Res, 3: 75–98. https://doi.org/10.1016/j.ncrna.2018.05.002. Erratumin: Noncoding RNA Res, 2020, 5: 220–221.
- Dodbele S, Mutlu N, Wilusz JE, 2021, Best practices to ensure robust investigation of circular RNAs: Pitfalls and tips. EMBO Rep, 22: e52072. https://doi.org/10.15252/embr.202052072
- Ju M, Kim D, Son G, et al., 2023, Circular RNAs in and out of cells: Therapeutic usages of circular RNAs. Mol Cells, 46: 33–40. https://doi.org/10.14348/molcells.2023.2170
- Xiao MS, Ai Y, Wilusz JE, 2020, Biogenesis and functions of circular RNAs come into focus. Trends Cell Biol, 30: 226–240. https://doi.org/10.1016/j.tcb.2019.12.004
- Münzel T, Hahad O, Sørensen M, et al., 2022, Environmental risk factors and cardiovascular diseases: A comprehensive expert review. Cardiovasc Res, 118: 2880–2902. https://doi.org/10.1093/cvr/cvab316
- Beekman M, Nederstigt C, Suchiman HED, et al., 2010, Genome-wide association study (GWAS) - identified disease risk alleles do not compromise human longevity. Proc Natl Acad Sci U S A, 107: 18046–18049. https://doi.org/10.1073/pnas.1003540107
- Eichler EE, Flint J, Gibson G, et al., 2010, Missing heritability and strategies for finding the underlying causes of complex disease. Nat Rev Genet, 11: 446–450. https://doi.org/10.1038/nrg2809
- Udali S, Guarini P, Moruzzi S, et al., 2013, Cardiovascular epigenetics: From DNA methylation to microRNAs. Mol Aspects Med, 34: 883–901. https://doi.org/10.1016/j.mam.2012.08.001
- Lloyd-Jones DM, Hong Y, Labarthe D, et al., 2010, Defining and setting national goals for cardiovascular health promotion and disease reduction: The American Heart Association’s strategic Impact Goal through 2020 and beyond. Circulation, 121: 586–613. https://doi.org/10.1161/CIRCULATIONAHA.109.192703
- Joyce BT, Gao T, Zheng Y, et al., 2021, Epigenetic age acceleration reflects long-term cardiovascular health. Circ Res, 129: 770–781. https://doi.org/10.1161/CIRCRESAHA.121.318965
- Davila-Velderrain J, Martinez-Garcia JC, Alvarez-Buylla ER, 2015, Modeling the epigenetic attractors landscape: Toward a post-genomic mechanistic understanding of development. Front Genet, 6: 160. https://doi.org/10.3389/fgene.2015.00160
- Carter AC, Chang HY, Church G, et al., 2017, Challenges and recommendations for epigenomics in precision health. Nat Biotechnol, 35: 1128–1132. https://doi.org/10.1038/nbt.4030
- Alexandar V, Nayar PG, Murugesan R, et al., 2015, CardioGenBase: A literature based multi-omics database for major cardiovascular diseases. PLoS One, 10: e0143188. https://doi.org/10.1371/journal.pone.0143188
- Louridas GE, Lourida KG, 2017, Conceptual foundations of systems biology explaining complex cardiac diseases. Healthcare (Basel), 5: 10. https://doi.org/10.3390/healthcare5010010
- Ahn AC, Tewari M, Poon CS, et al., 2006, The Limits of reductionism in medicine: Could systems biology offer an alternative? PLoS Med, 3: e208. https://doi.org/10.1371/journal.pmed.0030208
- Ahn AC, Tewari M, Poon CS, et al., 2006, The clinical applications of a systems approach. PLoS Med, 3: e209. https://doi.org/10.1371/journal.pmed.0030209
- Ung CY, Correia C, Billadeau DD, et al., 2023, Manifold epigenetics: A conceptual model that guides engineering strategies to improve whole-body regenerative health. Front Cell Dev Biol, 11: 1122422. https://doi.org/10.3389/fcell.2023.1122422
- Pattini L, Sassi R, Cerutti S, 2014, Dissecting heart failure through the multiscale approach of systems medicine. IEEE Trans Biomed Eng, 61: 1593–1603. https://doi.org/10.1109/TBME.2014.2307758
- Green S, 2013, When one model is not enough: Combining epistemic tools in systems biology. Stud Hist Philos Biol Biomed Sci, 44: 170–180. https://doi.org/10.1016/j.shpsc.2013.03.012
- Green S, Andersen H, 2019, Systems science and the art of interdisciplinary integration. Syst Res Behav Sci,36: 727–743. https://doi.org/10.1002/sres.2633
- Matheny M, Israni ST, Ahmed M, et al., (Eds.), 2019, Artificial Intelligence in Health Care: The Hope, the Hype, the Promise, the Peril. Washington, DC, USA: National Academy of Medicine, NAM Special Publication.
- Sethi Y, Patel N, Kaka N, et al., 2023, Precision Medicine and the future of cardiovascular diseases: A clinically oriented comprehensive review. J Clin Med, 12: 1799. https://doi.org/10.3390/jcm12051799
- Rainie L, Anderson J, Vogels E, 2021, Experts Doubt Ethical AI Design Will Be Broadly Adopted as the Norm in the Next Decade. United States: Pew Research Center.
- Brender TD, 2023, Medicine in the era of artificial intelligence: Hey Chatbot, Write Me an H&P. JAMA Intern Med, 183: 507–508. https://doi.org/10.1001/jamainternmed.2023.1832