Advances in nanoparticle-based drug delivery in cancer treatment
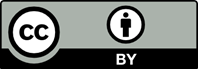
As a pathologically heterogeneous disease, cancer is one of the leading causes of global morbidity. According to the World Health Organization, approximately one in six deaths are caused by cancer. Fortunately, many cancers can be cured if diagnosed at early stages and treated efficiently. Despite the benefits of conventional cancer treatments such as surgery, chemotherapy, hormone therapy, and radiation therapy, they have several drawbacks, including cytotoxicity, inaccurate targeting of tumor cells, and multi-drug resistance, which underscore the importance of developing novel and effective strategies to improve diagnosis, prognosis, therapy, and patient survival. Recently, the advancement of nanotechnology has opened new horizons for cancer treatment thanks to the discovery of nanoparticles (NPs) and the small-sized molecules that revolutionized the drug delivery methods in cancerous tissues. The specific characteristics of NPs, such as reduced toxicity, improved permeability, and accurate targeting of tumor cells, provide a great advantage in cancer treatment and help to overcome the limitations and challenges of conventional cancer treatment methods. Besides, the role of NPs in immunotherapy has created a novel concept for cancer treatment. This review gives a brief overview regarding the importance of NPs and their targeting mechanism, as well as the challenges and limitations associated with their use in cancer treatment.
- Gavas S, Quazi S, Karpiński TM, 2021, Nanoparticles for cancer therapy: Current progress and challenges. Nanoscale Res Lett, 16: 173. https://doi.org/10.1186/s11671-021-03628-6
- Boisseau P, Loubaton B, 2011, Nanomedicine, nanotechnology in medicine. C R Phys, 12: 620–636. https://doi.org/10.1016/j.crhy.2011.06.001
- Bertrand N, Wu J, Xu X, et al., 2014, Cancer nanotechnology: The impact of passive and active targeting in the era of modern cancer biology. Adv Drug Deliv Rev, 66: 2–25. https://doi.org/10.1016/j.addr.2013.11.009
- Kalyane D, Raval N, Maheshwari R, et al., 2019, Employment of enhanced permeability and retention effect (EPR): Nanoparticle-based precision tools for targeting of therapeutic and diagnostic agent in cancer. Mater Sci Eng C Mater Biol Appl, 98: 1252–1276. https://doi.org/10.1016/j.msec.2019.01.066
- O’Brien MER, Wigler N, Inbar M, et al., 2004, Reduced cardiotoxicity and comparable efficacy in a phase III trial of pegylated liposomal doxorubicin HCl (CAELYX/Doxil) versus conventional doxorubicin for first-line treatment of metastatic breast cancer. Ann Oncol, 15: 440–449. https://doi.org/10.1093/annonc/mdh097
- Saha RN, Vasanthakumar S, Bende G, et al., 2010, Nanoparticulate drug delivery systems for cancer chemotherapy. Mol Membr Biol, 27: 215–231. https://doi.org/10.3109/09687688.2010.510804
- Chen Y, Gao DY, Huang L, 2015, In vivo delivery of miRNAs for cancer therapy: Challenges and strategies. Adv Drug Deliv Rev, 81: 128–141. https://doi.org/10.1016/j.addr.2014.05.009
- Zhang F, Correia A, Mäkilä E, et al., 2017, Receptor-mediated surface charge inversion platform based on porous silicon nanoparticles for efficient cancer cell recognition and combination therapy. ACS Appl Mater Interfaces, 9: 10034–10046. https://doi.org/10.1021/acsami.7b02196
- Bahrami B, Hojjat-Farsangi M, Mohammadi H, et al., 2017, Nanoparticles and targeted drug delivery in cancer therapy. Immunol Lett, 190: 64–83. https://doi.org/10.1016/j.imlet.2017.07.015
- Yao Y, Zhou Y, Liu L, et al., 2020, Nanoparticle-based drug delivery in cancer therapy and its role in overcoming drug resistance. Front Mol Biosci, 7: 193. https://doi.org/10.3389/fmolb.2020.00193
- Decuzzi P, Pasqualini R, Arap W, et al., 2009, Intravascular delivery of particulate systems: Does geometry really matter? Pharm Res, 26: 235–243. https://doi.org/10.1007/s11095-008-9697-x
- Venturoli D, Rippe B, 2005, Ficoll and dextran vs. globular proteins as probes for testing glomerular permselectivity: Effects of molecular size, shape, charge, and deformability. Am J Phys Renal Physiol, 288: F605–F613. https://doi.org/10.1152/ajprenal.00171.2004
- Prokop A, Davidson JM, 2008, Nanovehicular intracellular delivery systems. J Pharm Sci, 97: 3518–3590. https://doi.org/10.1002/jps.21270
- Yang Q, Jones SW, Parker CL, et al., 2014, Evading immune cell uptake and clearance requires PEG grafting at densities substantially exceeding the minimum for brush conformation. Mol Pharm, 11: 1250–1258. https://doi.org/10.1021/mp400703d
- Perrault SD, Walkey C, Jennings T, et al., 2009, Mediating tumor targeting efficiency of nanoparticles through design. Nano Lett, 9: 1909–1915. https://doi.org/10.1021/nl900031y
- Anselmo AC, Zhang M, Kumar S, et al., 2015, Elasticity of nanoparticles influences their blood circulation, phagocytosis, endocytosis, and targeting. ACS Nano, 9: 3169–3177. https://doi.org/10.1021/acsnano.5b00147
- Zylberberg C, Matosevic S, 2016, Pharmaceutical liposomal drug delivery: A review of new delivery systems and a look at the regulatory landscape. Drug Deliv, 23: 3319–3329. https://doi.org/10.1080/10717544.2016.1177136
- Dadwal A, Baldi A, Narang RK, 2018, Nanoparticles as carriers for drug delivery in cancer. Artif Cells Nanomed Biotechnol, 46: 295–305. https://doi.org/10.1080/21691401.2018.1457039
- Palazzolo S, Bayda S, Hadla M, et al., 2018, The clinical translation of organic nanomaterials for cancer therapy: A focus on polymeric nanoparticles, micelles, liposomes and exosomes. Curr Med Chem, 25: 4224–4268. https://doi.org/10.2174/0929867324666170830113755
- Murakami M, Cabral H, Matsumoto Y, et al., 2011, Improving drug potency and efficacy by nanocarrier-mediated subcellular targeting. Sci Transl Med, 3: 64ra2. https://doi.org/10.1126/scitranslmed.3001385
- Housman, G, Byler S, Heerboth S, et al., 2014, Drug resistance in cancer: An overview. Cancers (Basel), 6: 1769–1792. https://doi.org/10.3390/cancers6031769
- Schneider E, Hunke S, 1998, ATP-binding-cassette (ABC) transport systems: Functional and structural aspects of the ATP-hydrolyzing subunits/domains. FEMS Microbiol Rev, 22: 1–20. https://doi.org/10.1111/j.1574-6976.1998.tb00358.x
- Chintamani, Singh JP, Mittal MK, et al., 2005, Role of p-glycoprotein expression in predicting response to neoadjuvant chemotherapy in breast cancer--a prospective clinical study. World J Surg Oncol, 3: 61. https://doi.org/10.1186/1477-7819-3-61
- Allen JD, Brinkhuis RF, van Deemter L, et al., 2000, Extensive contribution of the multidrug transporters P-glycoprotein and Mrp1 to basal drug resistance. Cancer Res, 60: 5761–5766.
- Agarwal R, Kaye SB, 2003, Ovarian cancer: Strategies for overcoming resistance to chemotherapy. Nat Rev Cancer, 3: 502–516. https://doi.org/10.1038/nrc1123
- Kipp JE, 2004, The role of solid nanoparticle technology in the parenteral delivery of poorly water-soluble drugs. Int J Pharm, 284: 109–122. https://doi.org/10.1016/j.ijpharm.2004.07.019
- Zhang L, Chan JM, Gu FX, et al., 2008, Self-assembled lipid--polymer hybrid nanoparticles: A robust drug delivery platform. ACS Nano, 2: 1696–1702. https://doi.org/10.1021/nn800275r
- Wacker M, 2013, Nanocarriers for intravenous injection-- the long hard road to the market. Int J Pharm, 457: 50–62. https://doi.org/10.1016/j.ijpharm.2013.08.079
- Lu H, Zhang S, Wang J, et al., 2021, A review on polymer and lipid-based nanocarriers and its application to nano-pharmaceutical and food-based systems. Front Nutr, 8: 783831. https://doi.org/10.3389/fnut.2021.783831
- Patel T, Zhou J, Piepmeier JM, et al., 2012 Polymeric nanoparticles for drug delivery to the central nervous system. Adv Drug Deliv Rev, 64: 701–705. https://doi.org/10.1016/j.addr.2011.12.006
- Cabral H, Miyata K, Osada K, et al., 2018, Block copolymer micelles in nanomedicine applications. Chem Rev, 118: 6844–6892. https://doi.org/10.1021/acs.chemrev.8b00199
- Adams ML, Lavasanifar A, Kwon GS, 2003, Amphiphilic block copolymers for drug delivery. J Pharm Sci, 92: 1343–1355. https://doi.org/10.1002/jps.10397
- Hallan SS, Kaur P, Kaur V, et al., 2016, Lipid polymer hybrid as emerging tool in nanocarriers for oral drug delivery. Artif Cells Nanomed Biotechnol, 44: 334–349. https://doi.org/10.3109/21691401.2014.951721
- Xu HL, ZhuGe DL, Chen PP, et al., 2018, Silk fibroin nanoparticles dyeing indocyanine green for imaging-guided photo-thermal therapy of glioblastoma. Drug Deliv, 25: 364–375. https://doi.org/10.1080/10717544.2018.1428244
- Lo ST, Kumar A, Hsieh JT, et al., 2013, Dendrimer nanoscaffolds for potential theranostics of prostate cancer with a focus on radiochemistry. Mol Pharm, 10: 793–812. https://doi.org/10.1021/mp3005325
- Lu B, Xiong SB, Yang H, et al., 2006, Solid lipid nanoparticles of mitoxantrone for local injection against breast cancer and its lymph node metastases. Eur J Pharm Sci, 28: 86–95. https://doi.org/10.1016/j.ejps.2006.01.001
- Hornsby TK, Jakhmola A, Kolios MC, et al., 2023, A quantitative study of thermal and non-thermal mechanisms in ultrasound-induced nano-drug delivery. Ultrasound Med Biol, 49: 1288–1298. https://doi.org/10.1016/j.ultrasmedbio.2023.01.015
- Jakhmola A, Ashokkumar M, Grieser F, 2022, Sustainable synthesis and theoretical studies of polyhedral gold nanoparticles displaying high SERS activity, NIR absorption, and cellular uptake. J Phys Chem B, 26: 101016. https://doi.org/10.1016/j.mtchem.2022.101016
- Jakhmola A, Vecchione R, Onesto V, et al., 2021, A theoretical and experimental study on L-tyrosine and citrate mediated sustainable production of near infrared absorbing twisted gold nanorods. Mater Sci Eng C Mater Biol Appl, 118: 111515. https://doi.org/10.1016/j.msec.2020.111515
- Zhang X, Tian W, Cai X, et al., 2013, Hydrazinocurcumin Encapsuled nanoparticles “re-educate” tumor-associated macrophages and exhibit anti-tumor effects on breast cancer following STAT3 suppression. PLoS One, 8: e65896. https://doi.org/10.1371/journal.pone.0065896
- Byrne JD, Betancourt T, Brannon-Peppas L, 2008, Active targeting schemes for nanoparticle systems in cancer therapeutics. Adv Drug Deliv Rev, 60: 1615–1626. https://doi.org/10.1016/j.addr.2008.08.005
- Alexis F, Pridgen E, Molnar LK, et al., 2008, Factors affecting the clearance and biodistribution of polymeric nanoparticles. Mol Pharm, 5: 505–515. https://doi.org/10.1021/mp800051m
- Sarvari P, Sarvari P, Ramírez-Díaz I, et al., 2022, Advances of epigenetic biomarkers and epigenome editing for early diagnosis in breast cancer. Int J Mol Sci, 23: 9521. https://doi.org/10.3390/ijms23179521
- Shi J, Xiao Z, Kamaly N, et al., 2011, Self-assembled targeted nanoparticles: Evolution of technologies and bench to bedside translation. Acc Chem Res, 44: 1123–1134. https://doi.org/10.1021/ar200054n
- Danhier F, Feron O, Préat V, 2010, To exploit the tumor microenvironment: Passive and active tumor targeting of nanocarriers for anti-cancer drug delivery. J Control Release, 148: 135–146. https://doi.org/10.1016/j.jconrel.2010.08.027
- Bae YH, Park K, 2011, Targeted drug delivery to tumors: Myths, reality and possibility. J Control Release, 153: 198. https://doi.org/10.1016/j.jconrel.2011.06.001
- Nag OK, Delehanty JB, 2019, Active cellular and subcellular targeting of nanoparticles for drug delivery. Pharmaceutics, 11: 543. https://doi.org/10.3390/pharmaceutics11100543
- Torchilin VP, 2005, Recent advances with liposomes as pharmaceutical carriers. Nat Rev Drug Discov, 4: 145–160. https://doi.org/10.1038/nrd1632
- Carita AC, Eloy JO, Chorilli M, et al., 2018, Recent advances and perspectives in liposomes for cutaneous drug delivery. Curr Med Chem, 25: 606–635. https://doi.org/10.2174/0929867324666171009120154
- Sykes EA, Chen J, Zheng G, et al., 2014, Investigating the impact of nanoparticle size on active and passive tumor targeting efficiency. ACS Nano, 8: 5696–5706. https://doi.org/10.1021/nn500299p
- Carmeliet P, Jain RK, 2000, Angiogenesis in cancer and other diseases. Nature, 407: 249–257. https://doi.org/10.1038/35025220
- Matsumura Y, Maeda H, 1986, A new concept for macromolecular therapeutics in cancer chemotherapy: Mechanism of tumoritropic accumulation of proteins and the antitumor agent smancs. Cancer Res, 46: 6387–6392.
- Padera TP, Stoll BR, Tooredman JB, et al., 2004, Cancer cells compress intratumour vessels. Nature, 427: 695–695. https://doi.org/10.1038/427695a
- Jain RK, 1994, Barriers to drug delivery in solid tumors. Sci Am, 271: 58–65. https://doi.org/10.1038/scientificamerican0794-58