Amniotic Fluid-Derived Stem Cells: A Promising Resource for Cardiomyogenesis
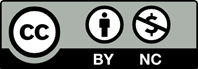
Amniotic fluid stem cells (AFSCs) are one of the prenatal stem cell populations isolated from the amniotic fluid/membrane. They include different subpopulations from various sources of origin. AFSCs have important characteristics, including high self-renewal and proliferation capacity, immunocompatibility and anti-inflammatory properties, and differentiation potential into cell types of all three germ layers in vitro. Given the cardiomyogenesis potential of AFSCs and their importance in tissue engineering and regenerative medicine, this review aims to address the current findings regarding the characteristics of the AFSCs. Moreover, we present the strategies and methods used for the differentiation of AFSCs into cardiomyocytes in vitro and in vivo. This review also describes and discusses findings regarding the possible signaling pathways, the well-known molecular regulators, and modifications that are important for AFSCs and their differentiation potential into the cardiomyocytes. In general, this review indicates that AFSCs can efficiently differentiate into cardiomyocytes by different methods. Moreover, induction of the ERK signaling pathway, upregulation of epigenetic modifiers (GCN5, EZH2, SUZ12, DNMT1/2, and HP-1α) and the cell cycle regulators (p53, p21, Rb, and p130), suppression of HDAC1/2 and stemness markers (OCT4, NANOG, SOX2, KLF4, and REX1), the relative expression of miR-34a and miR-145, and induction of the expression of structural and functional-specific genes of cardiomyocytes (GATA4, Nkx2.5, cTnT, MHC, Myh6, and Tnni3) are the most important molecular changes during the differentiation of AFSCs into the cardiomyocytes.
1. Loukogeorgakis S, Maghsoudlou P, De Coppi P. Recent developments in therapies with stem cells from amniotic fluid and placenta. Fetal Maternal Med Rev 2013;24:148–68.
2. Murphy SV, Atala A. Amniotic fluid and placental membranes: unexpected sources of highly multipotent cells. Semin Reprod Med 2013;31:62–8.
3. Modena AB, Fieni S. Amniotic fluid dynamics. Acta Biomed 2004;75:11–3.
4. Eslaminejad BM, Jahangir S. Tissue based-cell in application their and cells stem fluid amniotic regeneration. Int J Fertil Steril 2012;6:147–56.
5. Borlongan CV. Amniotic fluid as a source of engraftable stem cells. Brain Circ 2017;3:175–9.
6. Gao Y, Zhu Z, Zhao Y, Hua J, Ma Y, Guan W. Multilineage potential research of bovine amniotic fluid mesenchymal stem cells. Int J Mol Sci 2014;15:3698–710.
7. Torricelli F, Brizzi L, Bernabei PA, Gheri G, Di Lollo S, Nutini L, et al. Identification of hematopoietic progenitor cells in human amniotic fluid before the 12th week of gestation. Ital J Anat Embryol 1993;98:119–26.
8. Prusa AR, Marton E, Rosner M, Bernaschek G, Hengstschläger M. Oct-4-expressing cells in human amniotic fluid: a new source for stem cell research? Hum Reprod 2003;18:1489–93.
9. In 't Anker PS, Scherjon SA, Kleijburg-van der Keur C, de Groot-Swings GM, Claas FH, Fibbe WE, Kanhai HH. Isolation of mesenchymal stem cells of fetal or maternal origin from human placenta. Stem Cells 2004;22:1338–45.
10. Tsai MS, Lee JL, Chang YJ, Hwang SM. Isolation of human multipotent mesenchymal stem cells from second-trimester amniotic fluid using a novel two-stage culture protocol. Hum Reprod 2004;19:1450–6.
11. De Coppi P, Bartsch G Jr, Siddiqui MM, Xu T, Santos CC, Perin L, et al. Isolation of amniotic stem cell lines with potential for therapy. Nat Biotechnol 2007;25:100–6.
12. Trounson A. A fluid means of stem cell generation. Nat Biotechnol 2007;25:62–3.
13. Fang YH, Wang SPH, Chang HY, Yang PJ, Liu PY, Liu YW. Progress and challenges of amniotic fluid derived stem cells in therapy of ischemic heart disease. Int J Mol Sci 2020;22.
14. Toda A, Okabe M, Yoshida T, Nikaido T. The potential of amniotic membrane/amnion-derived cells for regeneration of various tissues. J Pharmacol Sci 2007;105:215–28.
15. Mauro A, Turriani M, Ioannoni A, Russo V, Martelli A, Di Giacinto O, et al. Isolation, characterization, and in vitro differentiation of ovine amniotic stem cells. Vet Res Commun 2010;34:25–8.
16. Antonucci I, Stuppia L, Kaneko Y, Yu S, Tajiri N, Bae EC, et al. Amniotic fluid as a rich source of mesenchymal stromal cells for transplantation therapy. Cell Transplant 2011;20:789–95.
17. Chen Q, Xiao P, Chen JN, Cai JY, Cai XF, Ding H, et al. AFM studies of cellular mechanics during osteogenic differentiation of human amniotic fluid-derived stem cells. Anal Sci 2010;26:1033–7.
18. Gekas J, Walther G, Skuk D, Bujold E, Harvey I, Bertrand OF. In vitro and in vivo study of human amniotic fluid-derived stem cell differentiation into myogenic lineage. Clin Exp Med 2010;10:1–6.
19. Decembrini S, Cananzi M, Gualdoni S, Battersby A, Allen N, Pearson RA, et al. Comparative analysis of the retinal potential of embryonic stem cells and amniotic fluid-derived stem cells. Stem Cells Dev 2011;20:851–63.
20. Guan X, Delo DM, Atala A, Soker S. In vitro cardiomyogenic potential of human amniotic fluid stem cells. J Tissue Eng Regen Med 2011;5:220–8.
21. Orciani M, Morabito C, Emanuelli M, Guarnieri S, Sartini D, Giannubilo SR, et al. Neurogenic potential of mesenchymallike stem cells from human amniotic fluid: the influence of extracellular growth factors. J Biol Regul Homeost Agents 2011;25:115–30.
22. Kooreman NG, Wu JC. Tumorigenicity of pluripotent stem cells: biological insights from molecular imaging. J R Soc Interface 2010;7:S753–63.
23. Maraldi T, Russo V. Amniotic fluid and placental membranes as sources of stem cells: progress and challenges. Int J Mol Sci 2022;23:5362.
24. Marei HE, Althani A, Afifi N, Hasan A, Caceci T, Pozzoli G, et al. p53 signaling in cancer progression and therapy. Cancer Cell Int 2021;21:703.
25. Cozene B, Antonucci I, Stuppia L. Activity of p53 in human amniotic fluid stem cells increases their potentiality as a candidate for stem cell therapy. Brain Circ 2019;5:134–9.
26. Sisakhtnezhad S, Alimoradi E, Akrami H. External factors in-fluencing mesenchymal stem cell fate in vitro. Eur J Cell Biol 2017;96:13–33.
27. Kunisaki SM, Armant M, Kao GS, Stevenson K, Kim H, Fauza DO. Tissue engineering from human mesenchymal amniocytes: a prelude to clinical trials. J Pediatr Surg 2007;42:974– 9; discussion 979–80.
28. Kim J, Lee Y, Kim H, Hwang KJ, Kwon HC, Kim SK, et al. Human amniotic fluid-derived stem cells have characteristics of multipotent stem cells. Cell Prolif 2007;40:75–90.
29. Pipino C, Pierdomenico L, Di Tomo P, Di Giuseppe F, Cianci E, D'Alimonte I, et al. Molecular and phenotypic characterization of human amniotic fluid-derived cells: a morphological and proteomic approach. Stem Cells Dev 2015;24:1415–28.
30. Di Trapani M, Bassi G, Fontana E, Giacomello L, Pozzobon M, Guillot PV, et al. Immune regulatory properties of CD117(pos) amniotic fluid stem cells vary according to gestational age. Stem Cells Dev 2015;24:132–43.
31. Savickiene J, Treigyte G, Baronaite S, Valiuliene G, Kaupinis A, Valius M, et al. Human amniotic fluid mesenchymal stem cells from second- and third-trimester amniocentesis: differentiation potential, molecular signature, and proteome analysis. Stem Cells Int 2015;2015:319238.
32. You Q, Tong X, Guan Y, Zhang D, Huang M, Zhang Y, et al. The biological characteristics of human third trimester amniotic fluid stem cells. J Int Med Res 2009;37:105–12.
33. Nadri S, Soleimani M. Comparative analysis of mesenchymal stromal cells from murine bone marrow and amniotic fluid. Cytotherapy 2007;9:729–37.
34. Tancharoen W, Aungsuchawan S, Pothacharoen P, Markmee R, Narakornsak S, Kieodee J, et al. Differentiation of mesenchymal stem cells from human amniotic fluid to vascular endothelial cells. Acta Histochem 2017;119:113–21.
35. Antonucci I, Provenzano M, Rodrigues M, Pantalone A, Salini V, Ballerini P, et al. Amniotic fluid stem cells: a novel source for modeling of human genetic diseases. Int J Mol Sci 2016;17:607.
36. Antonucci I, Crowley MG, Stuppia L. Amniotic fluid stem cell models: A tool for filling the gaps in knowledge for human genetic diseases. Brain Circ 2017;3:167–74.
37. Fang YH, Wang SPH, Gao ZH, Wu SN, Chang HY, Yang PJ, et al. Efficient cardiac differentiation of human amniotic fluidderived stem cells into induced pluripotent stem cells and their potential immune privilege. Int J Mol Sci 2020;21:2359.
38. Ambrósio CE, Orlandin JR, Oliveira VC, Motta LCB, Pinto PAF, Pereira VM, et al. Potential application of aminiotic stem cells in veterinary medicine. Anim Reprod 2020;16:24–30.
39. Abe Y, Ochiai D, Sato Y, Otani T, Fukutake M, Ikenoue S, et al. Amniotic fluid stem cells as a novel strategy for the treatment of fetal and neonatal neurological diseases. Placenta 2021;104:247–52.
40. Yeh YC, Wei HJ, Lee WY, Yu CL, Chang Y, Hsu LW, et al. Cellular cardiomyoplasty with human amniotic fluid stem cells: in vitro and in vivo studies. Tissue Eng Part A 2010;16:1925–36.
41. Bollini S, Cheung KK, Riegler J, Dong X, Smart N, Ghionzoli M, et al. Amniotic fluid stem cells are cardioprotective following acute myocardial infarction. Stem Cells Dev 2011; 20:1985–94.
42. Bollini S, Pozzobon M, Nobles M, Riegler J, Dong X, Piccoli M, et al. In vitro and in vivo cardiomyogenic differentiation of amniotic fluid stem cells. Stem Cell Rev Rep 2011; 7:364– 80.
43. Castellani C, Vescovo G, Ravara B, Franzin C, Pozzobon M, Tavano R, et al. The contribution of stem cell therapy to skeletal muscle remodeling in heart failure. Int J Cardiol 2013;168:2014–21.
44. Jiang G, Herron TJ, Di Bernardo J, Walker KA, O'Shea KS, Kunisaki SM. Human cardiomyocytes prior to birth by integration-free reprogramming of amniotic fluid cells. Stem Cells Transl Med 2016;5:1595–606.
45. Gasiūnienė M, Zubova A, Utkus A, Navakauskienė R. Epigenetic and metabolic alterations in human amniotic fluid stem cells induced to cardiomyogenic differentiation by DNA methyltransferases and p53 inhibitors. J Cell Biochem 2019;120:8129–43.
46. Gasiūnienė M, Valatkaitė E, Navakauskaitė A, Navakauskienė R. The effect of angiotensin ii, retinoic acid, egcg, and vitamin c on the cardiomyogenic differentiation induction of human amniotic fluid-derived mesenchymal stem cells. Int J Mol Sci 2020;21:8752
47. Markmee R, Aungsuchawan S, Tancharoen W, Narakornsak S, Pothacharoen P. Differentiation of cardiomyocyte-like cells from human amniotic fluid mesenchymal stem cells by combined induction with human platelet lysate and 5-azacytidine. Heliyon 2020;6:e04844.
48. Sano S, Sano T, Ishigami S, Ito T. Cardiac stem cell therapy: Does a newborn infant's heart have infinite potential for stem cell therapy? J Thorac Cardiovasc Surg 2022;163:242–7.
49. Via AG, Frizziero A, Oliva F. Biological properties of mesenchymal Stem Cells from different sources. Muscles Ligaments Tendons J 2012;2:154–62.
50. Jung SC, Park S. New sources, differentiation, and therapeutic uses of mesenchymal stem cells 2.0. Int J Mol Sci 2023;24:3938.
51. Sessarego N, Parodi A, Podestà M, Benvenuto F, Mogni M, Raviolo V, et al. Multipotent mesenchymal stromal cells from amniotic fluid: solid perspectives for clinical application. Haematologica 2008;93:339–46.
52. Alessio N, Pipino C, Mandatori D, Di Tomo P, Ferone A, Marchiso M, et al. Mesenchymal stromal cells from amniotic fluid are less prone to senescence compared to those obtained from bone marrow: An in vitro study. J Cell Physiol 2018;233:8996–9006.
53. Huang J, Ma W, Wei X, Yuan Z. Amniotic fluid mesenchymal stromal cells from early stages of embryonic development have higher self-renewal potential. In Vitro Cell Dev Biol Anim 2020;56:701–14.
54. Roubelakis MG, Pappa KI, Bitsika V, Zagoura D, Vlahou A, Papadaki HA, et al. Molecular and proteomic characterization of human mesenchymal stem cells derived from amniotic fluid: comparison to bone marrow mesenchymal stem cells. Stem Cells Dev 2007;16:931–52.
55. Gholizadeh-Ghalehaziz S, Farahzadi R, Fathi E, Pashaiasl M. A mini overview of isolation, characterization and application of amniotic fluid stem cells. Int J Stem Cells 2015;8:115– 20.
56. Phermthai T, Odglun Y, Julavijitphong S, Titapant V, Chuenwattana P, Vantanasiri C, et al. A novel method to derive amniotic fluid stem cells for therapeutic purposes. BMC Cell Biol 2010;11:79.
57. Thilakavathy K, Nordin N, Ramasamy R, Ghoraishizadeh P, Rohayu IMR, Singh G. Characteristics of full-term amniotic fluid-derived mesenchymal stem cells in different culture media, mesenchymal stem cells - isolation, characterization and applications. Phuc Van Pham, editor. IntechOpen. Available at: https://www.intechopen.com/chapters/55767. Accessed Jun 7, 2023.
58. Pappa KI, Anagnou NP. Novel sources of fetal stem cells: where do they fit on the developmental continuum? Regen Med 2009;4:423–33.
59. Shamsnajafabadi H, Soheili ZS. Amniotic fluid characteristics and its application in stem cell therapy: A review. I nt J Reprod Biomed 2022;20:627–43.
60. Larson A, Gallicchio VS. Amniotic derived stem cells: role and function in regenerative medicine. J Cell Sci Ther 2017;8:1–10.
61. Chen JY, Mou XZ, Du XC, Xiang C. Comparative analysis of biological characteristics of adult mesenchymal stem cells with different tissue origins. Asian Pac J Trop Med 2015;8:739–46.
62. Ramkisoensing AA, Pijnappels DA, Askar SF, Passier R, Swildens J, Goumans MJ, et al. Human embryonic and fetal mesenchymal stem cells differentiate toward three different cardiac lineages in contrast to their adult counterparts. PLoS One 2011;6:e24164.
63. Jiang S, Zhang S. Differentiation of cardiomyocytes from amniotic fluid-derived mesenchymal stem cells by combined induction with transforming growth factor β1 and 5-azacytidine. Mol Med Rep 2017;16:5887–93.
64. Liu YW, Roan JN, Wang SP, Hwang SM, Tsai MS, Chen JH, et al. Xenografted human amniotic fluid-derived stem cell as a cell source in therapeutic angiogenesis. Int J Cardiol 2013;168:66–75.
65. Luo H, Wang Z, Qi F, Wang D. Applications of human amniotic fluid stem cells in wound healing. Chin Med J (Engl) 2022;135:2272–81.
66. Soncini M, Vertua E, Gibelli L, Zorzi F, Denegri M, Albertini A, et al. Isolation and characterization of mesenchymal cells from human fetal membranes. J Tissue Eng Regen Med 2007;1:296–305.
67. Alviano F, Fossati V, Marchionni C, Arpinati M, Bonsi L, Franchina M, et al. Term Amniotic membrane is a high throughput source for multipotent Mesenchymal Stem Cells with the ability to differentiate into endothelial cells in vitro. BMC Dev Biol 2007;7:11.
68. Stefanidis K, Loutradis D, Koumbi L, Anastasiadou V, Dinopoulou V, Kiapekou E, et al. Deleted in AzoospermiaLike (DAZL) gene-expressing cells in human amniotic fluid: a new source for germ cells research? Fertil Steril 2008;90:798–804.
69. Rasini V, Dominici M, Kluba T, Siegel G, Lusenti G, Northoff H, et al. Mesenchymal stromal/stem cells markers in the human bone marrow. Cytotherapy 2013;15:292–306.
70. Mareschi K, Ferrero I, Rustichelli D, Aschero S, Gammaitoni L, Aglietta M, et al. Expansion of mesenchymal stem cells isolated from pediatric and adult donor bone marrow. J Cell Biochem 2006;97:744–54.
71. Schallmoser K, Bartmann C, Rohde E, Reinisch A, Kashofer K, Stadelmeyer E, et al. Human platelet lysate can replace fetal bovine serum for clinical-scale expansion of functional mesenchymal stromal cells. Transfusion 2007;47:1436–46.
72. Sotiropoulou PA, Perez SA, Salagianni M, Baxevanis CN, Papamichail M. Characterization of the optimal culture conditions for clinical scale production of human mesenchymal stem cells. Stem Cells 2006;24:462–71.
73. Deschaseaux F, Gindraux F, Saadi R, Obert L, Chalmers D, Herve P. Direct selection of human bone marrow mesenchymal stem cells using an anti-CD49a antibody reveals their CD45med,low phenotype. Br J Haematol 2003;122:506–17.
74. Jones EA, Kinsey SE, English A, Jones RA, Straszynski L, Meredith DM, et al. Isolation and characterization of bone marrow multipotential mesenchymal progenitor cells. Arthritis Rheum 2002;46:3349–60.
75. Conget PA, Minguell JJ. Phenotypical and functional properties of human bone marrow mesenchymal progenitor cells. J Cell Physiol 1999;181:67–73.
76. Majumdar MK, Keane-Moore M, Buyaner D, Hardy WB, Moorman MA, McIntosh KR, et al. Characterization and functionality of cell surface molecules on human mesenchymal stem cells. J Biomed Sci 2003;10:228–41.
77. Gronthos S, Mankani M, Brahim J, Robey PG, Shi S. Postnatal human dental pulp stem cells (DPSCs) in vitro and in vivo. Proc Natl Acad Sci U S A 2000;97:13625–30.
78. Ringe J, Strassburg S, Neumann K, Endres M, Notter M, Burmester GR, et al. Towards in situ tissue repair: human mes-enchymal stem cells express chemokine receptors CXCR1, CXCR2 and CCR2, and migrate upon stimulation with CXCL8 but not CCL2. J Cell Biochem 2007;101:135–46.
79. Walsh S, Jefferiss C, Stewart K, Jordan GR, Screen J, Beresford JN. Expression of the developmental markers STRO-1 and alkaline phosphatase in cultures of human marrow stromal cells: regulation by fibroblast growth factor (FGF)- 2 and relationship to the expression of FGF receptors 1-4. Bone 2000;27:185–95.
80. Sullivan MO, Gordon-Evans WJ, Fredericks LP, Kiefer K, Conzemius MG, Griffon DJ. Comparison of mesenchymal stem cell surface markers from bone marrow aspirates and adipose stromal vascular fraction sites. Front Vet Sci 2015;2:82.
81. Zannettino AC, Paton S, Arthur A, Khor F, Itescu S, Gimble JM, et al. Multipotential human adipose-derived stromal stem cells exhibit a perivascular phenotype in vitro and in vivo. J Cell Physiol 2008;214:413–21.
82. Gill ME, Hu YC, Lin Y, Page DC. Licensing of gametogenesis, dependent on RNA binding protein DAZL, as a gateway to sexual differentiation of fetal germ cells. Proc Natl Acad Sci U S A 2011;108:7443–8.
83. Besmer P, Manova K, Duttlinger R, Huang EJ, Packer A, Gyssler C, et al. The kit-ligand (steel factor) and its receptor ckit/W: pleiotropic roles in gametogenesis and melanogenesis. Dev Suppl 1993:125–37.
84. Sisakhtnezhad S, Bahrami AR, Matin MM, Dehghani H, Momeni-Moghaddam M, Boozarpour S, et al. The molecular signature and spermatogenesis potential of newborn chicken spermatogonial stem cells in vitro. In Vitro Cell Dev Biol Anim 2015;51:415–25.
85. Afsartala Z, Rezvanfar MA, Hodjat M, Tanha S, Assadollahi V, Bijangi K, et al. Amniotic membrane mesenchymal stem cells can differentiate into germ cells in vitro. In Vitro Cell Dev Biol Anim 2016;52:1060–71.
86. Müller I, Kordowich S, Holzwarth C, Spano C, Isensee G, Staiber A, et al. Animal serum-free culture conditions for isolation and expansion of multipotent mesenchymal stromal cells from human BM. Cytotherapy 2006;8:437–44.
87. Murry CE, Soonpaa MH, Reinecke H, Nakajima H, Nakajima HO, Rubart M, et al. Haematopoietic stem cells do not transdifferentiate into cardiac myocytes in myocardial infarcts. Nature 2004;428:664–8.
88. Reinecke H, Poppa V, Murry CE. Skeletal muscle stem cells do not transdifferentiate into cardiomyocytes after cardiac grafting. J Mol Cell Cardiol 2002;34:241–9.
89. Iijima Y, Nagai T, Mizukami M, Matsuura K, Ogura T, Wada H, et al. Beating is necessary for transdifferentiation of skeletal muscle-derived cells into cardiomyocytes. Faseb J 2003;17:1361–3.
90. Ieda M, Fu JD, Delgado-Olguin P, Vedantham V, Hayashi Y, Bruneau BG, et al. Direct reprogramming of fibroblasts into functional cardiomyocytes by defined factors. Cell 2010;142:375–86.
91. Chen Y, Yang Z, Zhao ZA, Shen Z. Direct reprogramming of fibroblasts into cardiomyocytes. Stem Cell Res Ther 2017;8:118.
92. Fu JD, Srivastava D. Direct reprogramming of fibroblasts into cardiomyocytes for cardiac regenerative medicine. Circ J 2015;79:245–54.
93. Ebert AD, Diecke S, Chen IY, Wu JC. Reprogramming and transdifferentiation for cardiovascular development and regenerative medicine: where do we stand? EMBO Mol Med 2015;7:1090–103.
94. Sadahiro T. Cardiac regeneration with pluripotent stem cellderived cardiomyocytes and direct cardiac reprogramming. Regen Ther 2019;11:95–100.
95. Bhuvanalakshmi G, Arfuso F, Kumar AP, Dharmarajan A, Warrier S. Epigenetic reprogramming converts human Wharton's jelly mesenchymal stem cells into functional cardiomyocytes by differential regulation of Wnt mediators. Stem Cell Res Ther 2017;8:185.
96. Perán M, Marchal JA, López E, Jiménez-Navarro M, Boulaiz H, Rodríguez-Serrano F, et al. Human cardiac tissue induces transdifferentiation of adult stem cells towards cardiomyocytes. Cytotherapy 2010;12:332–7.
97. Guan K, Wagner S, Unsöld B, Maier LS, Kaiser D, Hemmerlein B, et al. Generation of functional cardiomyocytes from adult mouse spermatogonial stem cells. Circ Res 2007;100:1615– 25.
98. Budniatzky I, Gepstein L. Concise review: reprogramming strategies for cardiovascular regenerative medicine: from induced pluripotent stem cells to direct reprogramming. Stem Cells Transl Med 2014;3:448–57.
99. Qin Y, Chen Y, Li J. Research progress of cell co-culture method. Zhonghua Wei Zhong Bing Ji Jiu Yi Xue 2016;28:765–8.
100. Kook YM, Jeong Y, Lee K, Koh WG. Design of biomimetic cellular scaffolds for co-culture system and their application. J Tissue Eng 2017;8:2041731417724640.
101. Kuppusamy P, Kim D, Soundharrajan I, Hwang I, Choi KC. Adipose and muscle cell co-culture system: a novel in vitro tool to mimic the in vivo cellular environment. Biology (Basel) 2020;10:6.
102. Yoon J, Shim WJ, Ro YM, Lim DS. Transdifferentiation of mesenchymal stem cells into cardiomyocytes by direct cellto-cell contact with neonatal cardiomyocyte but not adult cardiomyocytes. Ann Hematol 2005;84:715–21.
103. Bao W, Ballard VL, Needle S, Hoang B, Lenhard SC, Tunstead JR, et al. Cardioprotection by systemic dosing of thymosin beta four following ischemic myocardial injury. Front Pharmacol 2013;4:149.
104. Maioli M, Contini G, Santaniello S, Bandiera P, PigliaruG, Sanna R, et al. Amniotic fluid stem cells morph into a cardiovascular lineage: analysis of a chemically induced cardiac and vascular commitment. Drug Des Devel Ther 2013;7:1063–73.
105. Bitsika V, Roubelakis MG, Zagoura D, Trohatou O, Makridakis M, Pappa KI, et al. Human amniotic fluid-derived mesenchymal stem cells as therapeutic vehicles: a novel approach for the treatment of bladder cancer. Stem Cells Dev 2012;21:1097–111.
106. Woodbury D, Kramer BC, Reynolds K, Marcus AJ, Coyne TM, Black IB. Long-term cryopreserved amniocytes retain proliferative capacity and differentiate to ectodermal and mesodermal derivatives in vitro. Mol Reprod Dev 2006;73:1463–72.
107. Christophersen NS, Helin K. Epigenetic control of embryonic stem cell fate. J Exp Med 2010;207:2287–95.
108. Minarovits J, Banati F, Szenthe K, Niller HH. Epigenetic regulation. Adv Exp Med Biol 2016;879:1–25.
109. Al Aboud NM, Tupper C, Jialal I. Genetics, epigenetic mechanism. 2022 Aug 8. In: StatPearls [Internet]. Treasure Island (FL): StatPearls Publishing; 2023 Jan–.
110. Cakouros D, Gronthos S. Epigenetic regulators of mesenchymal stem/stromal cell lineage determination. Curr Osteoporos Rep 2020;18:597–605.
111. Teven CM, Liu X, Hu N, Tang N, Kim SH, Huang E, et al. Epigenetic regulation of mesenchymal stem cells: a focus on osteogenic and adipogenic differentiation. Stem Cells Int 2011;2011:201371.
112. Vasilatou D, Papageorgiou SG, Dimitriadis G, Pappa V. Epigenetic alterations and microRNAs: new players in the pathogenesis of myelodysplastic syndromes. Epigenetics 2013;8:561–70.
113. Jafari A, Rezaei-Tavirani M, Farhadihosseinabadi B, Zali H, Niknejad H. Human amniotic mesenchymal stem cells to promote/suppress cancer: two sides of the same coin. Stem Cell Res Ther 2021;12:126.
114. Mirabella T, Gentili C, Daga A, Cancedda R. Amniotic fluid stem cells in a bone microenvironment: driving host angiogenic response. Stem Cell Res 2013;11:540–51.
115. Fauza DO. Transamniotic stem cell therapy: a novel strategy for the prenatal management of congenital anomalies. Pediatr Res 2018;83:241–8.
116. Savickienė J, Baronaitė S, Zentelytė A, Treigytė G, Navakauskienė R. Senescence-associated molecular and epigenetic alterations in mesenchymal stem cell cultures from amniotic fluid of normal and fetus-affected pregnancy. Stem Cells Int 2016;2016:2019498.
117. Savickienė J, Matuzevičius D, Baronaitė S, Treigytė G, Krasovskaja N, Zaikova I, et al. Histone modifications pattern associated with a state of mesenchymal stem cell cultures derived from amniotic fluid of normal and fetus-affected gestations. J Cell Biochem 2017;118:3744–55.
118. Zentelytė A, Gasiūnienė M, Treigytė G, Baronaitė S, Savickienė J, Borutinskaitė V, et al. Epigenetic regulation of amniotic fluid mesenchymal stem cell differentiation to the mesodermal lineages at normal and fetus-diseased gestation. J Cell Biochem 2020;121:1811–22.
119. Upadhyaya P, Di Serafino A, Sorino L, Ballerini P, Marchisio M, Pierdomenico L, et al. Genetic and epigenetic modifications induced by chemotherapeutic drugs: human amniotic fluid stem cells as an in-vitro model. BMC Med Genomics 2019;12:146.
120. Phermthai T, Pokathikorn P, Wichitwiengrat S, Thongbopit S, Tungprasertpol K, Julavijitphong S. P53 mutation and epigenetic imprinted IGF2/H19 gene analysis in mesenchymal stem cells derived from amniotic fluid, amnion, endometrium, and wharton's jelly. Stem Cells Dev 2017;26:1344–54.
121. Asumda FZ. Towards the development of a reliable protocol for mesenchymal stem cell cardiomyogenesis. Stem Cell Discovery 2013;3:13–21.
122. Rosenblatt-Velin N, Lepore MG, Cartoni C, Beermann F, Pedrazzini T. FGF-2 controls the differentiation of resident cardiac precursors into functional cardiomyocytes. J Clin Invest 2005;115:1724–33.
123. Xu H, Yi Q, Yang C, Wang Y, Tian J, Zhu J. Histone modifications interact with DNA methylation at the GATA4 promoter during differentiation of mesenchymal stem cells into cardiomyocyte-like cells. Cell Prolif 2016;49:315–29.
124. Yi Q, Xu H, Yang K, Wang Y, Tan B, Tian J, et al. Islet-1 induces the differentiation of mesenchymal stem cells into cardiomyocyte-like cells through the regulation of Gcn5 and DNMT-1. Mol Med Rep 2017;15:2511–20.
125. Ratajczak MZ, Marycz K, Poniewierska-Baran A, Fiedorowicz K, Zbucka-Kretowska M, Moniuszko M. Very small embryonic-like stem cells as a novel developmental concept and the hierarchy of the stem cell compartment. Adv Med Sci 2014;59:273–80.
126. Yoon BS, Yoo SJ, Lee JE, You S, Lee HT, Yoon HS. Enhanced differentiation of human embryonic stem cells into cardiomyocytes by combining hanging drop culture and 5-azacytidine treatment. Differentiation 2006;74:149–59.
127. Khajeniazi S, Solati M, Yazdani Y, Soleimani M, Kianmehr A. Synergistic induction of cardiomyocyte differentiation from human bone marrow mesenchymal stem cells by interleukin 1β and 5-azacytidine. Biol Chem 2016;397:1355–64.
128. Qian Q, Qian H, Zhang X, Zhu W, Yan Y, Ye S, et al. 5-Azacytidine induces cardiac differentiation of human umbilical cord-derived mesenchymal stem cells by activating extracellular regulated kinase. Stem Cells Dev 2012;21:67–75.
129. Ren J, Huang D, Li R, Wang W, Zhou C. Control of mesenchymal stem cell biology by histone modifications. Cell Biosci 2020;10:11.
130. Feng C, Zhu J, Zhao L, Lu T, Zhang W, Liu Z, et al. Suber-oylanilide hydroxamic acid promotes cardiomyocyte differentiation of rat mesenchymal stem cells. Exp Cell Res 2009;315:3044–51.
131. Li L, Zhu J, Tian J, Liu X, Feng C. A role for Gcn5 in cardiomyocyte differentiation of rat mesenchymal stem cells. Mol Cell Biochem 2010;345:309–16.
132. Rajasingh J, Thangavel J, Siddiqui MR, Gomes I, Gao XP, Kishore R, et al. Improvement of cardiac function in mouse myocardial infarction after transplantation of epigenetically-modified bone marrow progenitor cells. PLoS One 2011;6:e22550.
133. Wang M, Yu Q, Wang L, Gu H. Distinct patterns of histone modifications at cardiac-specific gene promoters between cardiac stem cells and mesenchymal stem cells. Am J Physiol Cell Physiol 2013;304:1080–90.
134. Lu DF, Wang Y, Su ZZ, Zeng ZH, Xing XW, He ZY, et al. Knockdown of the HDAC1 promotes the directed differentiation of bone mesenchymal stem cells into cardiomyocytes. PLoS One 2014;9:e92179.
135. Sdek P, Zhao P, Wang Y, Huang CJ, Ko CY, Butler PC, et al. Rb and p130 control cell cycle gene silencing to maintain the postmitotic phenotype in cardiac myocytes. J Cell Biol 2011;194:407–23.