Combining PET and Compton imaging with edge-on CZT detectors for enhanced diagnostic capabilities
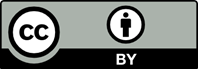
The key metrics for positron emission tomography (PET) imaging devices include the capability to capture the maximum available amount of annihilation photon information while generating high-quality images of the radiation distribution. This capability carries clinical implications by reducing scanning time for imaging, thus reducing radiation exposure for patients. However, imaging quality is degraded by positron range effects and the non-collinearity of positron annihilation photons. Utilizing an edge-on configuration of cadmium zinc telluride (CZT) detector crystals offers a potential solution to increase PET sensitivity. The high cross-section of CZT and its capacity to detect both 511 keV annihilation gammas and high-energy prompt gammas, along with multiple photon interaction events, contribute to this increased sensitivity. In this study, we propose a dual-panel edge-on CZT detector system comprised of 4 × 4 × 0.5 cm3 CZT detectors, with panel dimensions of 20 × 15 cm2 and a thickness of 4 cm. In this study, we demonstrate the increased sensitivity of our imaging system due to the detection of the Compton kinematics of high-energy gammas originating from prompt-gamma-emitting isotopes. This was achieved using Monte Carlo simulations of a prompt-gamma-emitting isotope,72As, with mean positron ranges >3 mm. Our system’s dynamic energy range, capable of detecting gammas up to 1.2 MeV, allows it to operate in a dual-mode fashion as both a Compton camera (CC) and standard PET. By presenting reconstructions of 72As, we highlight the absence of positron range effects in CC reconstructions compared to PET reconstructions. In addition, we evaluate the system’s increased sensitivity resulting from its ability to detect high-energy prompt gammas.
- Dolgin E. Radioactive drugs emerge from the shadows to storm the market. Nat Biotechnol. 2018;36(12):1125-1127. doi: 10.1038/nbt1218-1125
- Bodei L, Herrmann K, Schöder H, Scott AM, Lewis JS. Radiotheranostics in oncology: Current challenges and emerging opportunities. Nat Rev Clin Oncol. 2022;19(8):534-550. doi: 10.1038/s41571-022-00652-y
- Sgouros G, Bodei L, McDevitt MR, Nedrow JR. Radiopharmaceutical therapy in cancer: Clinical advances and challenges. Nat Rev Drug Discov. 2020;19(9):589-608. doi: 10.1038/s41573-020-0073-9
- Chomet M, Schreurs M, Vos R, et al. Performance of nanoScan PET/CT and PET/MR for quantitative imaging of 18F and 89Zr as compared with ex vivo biodistribution in tumor-bearing mice. EJNMMI Res. 2021;11:57. doi: 10.1186/s13550-021-00799-2
- Ferguson S, Jans HS, Wuest M, Riauka T, Wuest F. Comparison of scandium-44 g with other PET radionuclides in pre-clinical PET phantom imaging. EJNMMI Phys. 2019;6(1):23. doi: 10.1186/s40658-019-0260-0
- PSMA PET Scan for Prostate Cancer. Available from: https:// www.uchicagomedicine.org/cancer/types-treatments/ prostate-cancer/screening-diagnosis/psma-pet [Last accessed on 2023 Nov 29].
- Hoshi S, Yaginuma K, Meguro S, et al. PSMA targeted molecular imaging and radioligand therapy for prostate cancer: Optimal patient and treatment issues. Curr Oncol. 2023;30(8):7286-7302. doi: 10.3390/curroncol30080529
- Sutherland DE, Azad AA, Murphy DG, Eapen RS, Kostos L, Hofman MS. Role of FDG PET/CT in management of patients with prostate cancer. Semin Nucl Med. 2024;54(1):4-13. doi: 10.1053/j.semnuclmed.2023.06.005
- Kuzmenko NK. Updated decay data evaluation for 68Ga. Appl Radiat Isot. 2019;152:188-192. doi: 10.1016/j.apradiso.2019.07.008
- Piron S, Verhoeven J, Descamps B, et al. Intra-individual dynamic comparison of 18F-PSMA-11 and 68Ga-PSMA-11 in LNCaP xenograft bearing mice. Sci Rep. 2020;10(1):21068. doi: 10.1038/s41598-020-78273-7
- Huang S, Ong S, McKenzie D, et al. Comparison of 18F-based PSMA radiotracers with [68Ga]Ga-PSMA-11 in PET/CT imaging of prostate cancer-a systematic review and meta-analysis. Prostate Cancer Prostatic Dis. 2023:1-11. doi: 10.1038/s41391-023-00755-2
- Roberts MJ, Maurer T, Perera M, et al. Using PSMA imaging for prognostication in localized and advanced prostate cancer. Nat Rev Urol. 2023;20(1):23-47. doi: 10.1038/s41585-022-00670-6
- George SC, Samuel EJ. Developments in 177Lu-based radiopharmaceutical therapy and dosimetry. Front Chem. 2023;11:1218670. doi: 10.3389/fchem.2023.1218670.
- Carter LM, Kesner AL, Pratt EC, et al. The impact of positron range on PET resolution, evaluated with phantoms and PHITS monte carlo simulations for conventional and non-conventional radionuclides. Mol Imaging Biol. 2020;22(1):73-84. doi: 10.1007/s11307-019-01337-2
- Beekman FJ, Kamphuis C, Koustoulidou S, Ramakers RM, Goorden MC. Positron range-free and multi-isotope tomography of positron emitters. Phys Med Biol. 2021; 66(6):065011. doi: 10.1088/1361-6560/abe5fc
- Pratt EC, Lopez-Montes A, Volpe A, et al. Simultaneous quantitative imaging of two PET radiotracers via the detection of positron-electron annihilation and prompt gamma emissions. Nat Biomed Eng. 2023;7(8):1028-1039. doi: 10.1038/s41551-023-01060-y
- Severin GW, Engle JW, Nickles RJ, Barnhart TE. 89Zr radiochemistry for positron emission tomography. Med Chem. 2011;7(5):389-394. doi: 10.2174/157340611796799186
- Deri MA, Zeglis BM, Francesconi LC, Lewis JS. PET imaging with 89Zr: From radiochemistry to the clinic. Nucl Med Biol. 2013;40(1):3-14. doi: 10.1016/j.nucmedbio.2012.08.004
- Yoon JK, Park BN, Ryu EK, An YS, Lee SJ. Current perspectives on 89Zr-PET imaging. Int J Mol Sci. 2020;21(12):4309. doi: 10.3390/ijms21124309
- Sarcan ET, Silindir-Gunay M, Ozer AY, Hartman N. 89Zr as a promising radionuclide and it’s applications for effective cancer imaging. J Radioanal Nucl Chem. 2021;330(1):15-28. doi: 10.1007/s10967-021-07928-0
- Lee YJ, Van den Berg NS, Duan H, et al. 89Zr-panitumumab combined with 18F-FDG PET improves detection and staging of head and neck squamous cell carcinoma. Clin Cancer Res. 2022;28(20):4425-4434. doi: 10.1158/1078-0432.CCR-22-0094
- Knight JC, Mosley MJ, Kersemans V, et al. Dual-isotope imaging allows in vivo immunohistochemistry using radiolabelled antibodies in tumours. Nucl Med Biol. 2019;70:14-22. doi: 10.1016/j.nucmedbio.2019.01.010
- Yoshida E, Tashima H, Nagatsu K, et al. Whole gamma imaging: A new concept of PET combined with Compton imaging. Phys Med Biol. 2020;65(12):125013. doi: 10.1088/1361-6560/ab8e89
- Tashima H, Yoshida E, Wakizaka H, et al. 3D Compton image reconstruction method for whole gamma imaging. Phys Med Biol. 2020;65(22):225038. doi: 10.1088/1361-6560/abb92e
- Giovagnoli D, Bousse A, Beaupere N, et al. A pseudo-TOF image reconstruction approach for three-gamma small animal imaging. IEEE Trans Radiat Plasma Med Sci. 2021;5(6):826-834. doi: 10.1109/TRPMS.2020.3046409
- Llosá G, Rafecas M. Hybrid PET/Compton-camera imaging: An imager for the next generation. Eur Phys J Plus. 2023;138(3):214. doi: 10.1140/epjp/s13360-023-03805-9
- Parajuli RK, Sakai M, Parajuli R, Tashiro M. Development and applications of compton camera-a review. Sensors (Basel). 2022;22(19):7374. doi: 10.3390/s22197374
- Abbaszadeh S, Gu Y, Sikora U, Levin CS. First Acquisition of Data from a Prototype 3-D Position Sensitive CZT PET System. In: 2014 IEEE Nuclear Science Symposium and Medical Imaging Conference (NSS/MIC); 2014. p. 1-2. doi: 10.1109/NSSMIC.2014.7430998
- Abbaszadeh S, Gu Y, Reynolds PD, Levin CS. Characterization of a sub-assembly of 3D position sensitive cadmium zinc telluride detectors and electronics from a sub-millimeter resolution PET system. Phys Med Biol. 2016;61(18):6733. doi: 10.1088/0031-9155/61/18/6733
- Abbaszadeh S, Levin CS. New-generation small animal positron emission tomography system for molecular imaging. J Med Imaging (Bellingham). 2017;4(1):011008. doi: 10.1117/1.JMI.4.1.011008
- Abbaszadeh S, Chinn G, Levin CS. Positioning true coincidences that undergo inter-and intra-crystal scatter for a sub-mm resolution cadmium zinc telluride-based PET system. Phys Med Biol. 2018;63(2):025012. doi: 10.1088/1361-6560/aa9a2b
- Li M, Abbaszadeh S. Influence of channel configuration on bandwidth of cadmium zinc telluride detector with a cross-strip pattern. Radiat Phys Chem. 2019;155:213-216. doi: 10.1016/j.radphyschem.2018.04.015
- Vernekohl D, Abbaszadeh S, Gu Y, Levin CS. Robust detector calibration for a novel PET system based on cross-strip CZT detectors. IEEE Trans Radiat Plasma Med Sci. 2019;3(6):626-633. doi: 10.1109/TRPMS.2018.2878574
- Li M, Yockey B, Abbaszadeh S. Design study of a dedicated head and neck cancer PET system. IEEE Trans Radiat Plasma Med Sci. 2020;4(4):489-497. doi: 10.1109/TRPMS.2020.2964293
- Li M, Wang Y, Abbaszadeh S. Development and initial characterization of a high-resolution PET detector module with DOI. Biomed Phys Eng Express. 2020;6(6):065020. doi: 10.1088/2057-1976/abbd4f
- Farahmandzadeh M, Abbaszadeh S. A machine learning approach for identifying correct sequence of multiple interaction photon event in PET systems. J Nucl Med. 2020;61(Suppl 1):1514-1514.
- Nasiri N, Abbaszadeh S. A Deep Learning Approach to Correctly Identify the Sequence of Coincidences in Cross- Strip CZT Detectors. In: Medical Imaging 2021: Physics of Medical Imaging. Vol. 11595. Bellingham: SPIE; 2021. p. 1011-1018. doi: 10.1117/12.2582063
- Wang Y, Herbst R, Abbaszadeh S. Electronic noise characterization of a dedicated head-and-neck cancer PET based on CZT. J Nucl Med. 2021;62(Suppl 1):1154.
- Wang Y, Herbst R, Abbaszadeh S. Back-end readout electronic design and initial results: A head-and-neck dedicated PET system based on CZT. In: Medical Imaging 2021: Physics of Medical Imaging. Vol. 11595. Bellingham: SPIE; 2021. p. 262-267. doi: 10.1117/12.2576598
- Wang Y, Herbst R, Abbaszadeh S. Development and characterization of modular readout design for two-panel head-and-neck dedicated PET system based on CZT detectors. IEEE Trans Radiat Plasma Med Sci. 2022;6(5):517-521. doi: 10.1109/TRPMS.2021.3111547
- Enlow E, Diba M, Clayton J, Harris B, Abbaszadeh S. Impact of flexible circuit bonding and system integration on energy resolution of cross-strip CZT detectors. IEEE Trans Radiat Plasma Med Sci. 2023;7(6):580-586. doi: 10.1109/TRPMS.2023.3256406
- Romanchek G, Abbaszadeh S. Parameter selection for convex optimization time calibration for a 2-panel PET system. In: Medical Imaging 2023: Physics of Medical Imaging. Vol. 12463. Bellingham: SPIE; 2023. p. 828-834. doi: 10.1117/12.2654423
- Technical Documentation. Available from: https:// pdf.medicalexpo.com/pdf/mediso/npmpcb-0817- webpdf/94149-251994.html [Last accessed on 2024 May 09].
- Bao Q, Newport D, Chen M, Stout DB, Chatziioannou AF. Performance evaluation of the inveon dedicated PET preclinical tomograph based on the NEMA NU-4 standards. J Nucl Med. 2009;50(3):401-408. doi: 10.2967/jnumed.108.056374
- Abbaszadeh S, Kupinski M, Furenlid L. βγ imaging: Simultaneous detection of single-gamma and position-annihilation with CZT-based PET scanner. J Nucl Med. 2023;64(Suppl 1):P1282.
- Sarrut D, Arbor N, Baudier T, et al. The OpenGATE ecosystem for monte carlo simulation in medical physics. Phys Med Biol. 2022;67(18):184001. doi: 10.1088/1361-6560/ac8c83
- Agostinelli S, Allison J, Amako K, et al. Geant4-a simulation toolkit. Nucl Instrum Methods Phys Res A. 2003;506(3):250-303. doi: 10.1016/S0168-9002(03)01368-8
- Parra L, Barrett HH. List-mode likelihood: EM algorithm and image quality estimation demonstrated on 2-D PET. IEEE Trans Med Imaging. 1998;17(2):228-235. doi: 10.1109/42.700734
- Boellaard R. Standards for PET image acquisition and quantitative data analysis. J Nucl Med. 2009;50(Suppl 1):11S-20S. doi: 10.2967/jnumed.108.057182
- Aguiar P, Rafecas M, Ortuño JE, et al. Geometrical and monte carlo projectors in 3D PET reconstruction. Med Phys. 2010;37(11):5691-5702. doi: 10.1118/1.3501884
- Lojacono X. Image Reconstruction for Compton Camera with Application to Hadrontherapy. Thesis. France: INSA de Lyon; 2013. Available from: https://theses.hal.science/tel- 01081066 [Last accessed on 2023 Jul 15].
- Kim SM, Lee JS, Lee MN, et al. Two approaches to implementing projector-backprojector pairs for 3D reconstruction from compton scattered data. Nucl Instrum Methods Phys Res A. 2007;571(1):255-258. doi: 10.1016/j.nima.2006.10.076
- Feng Y, Létang JM, Sarrut D, Maxim V. Influence of doppler broadening model accuracy in compton camera list-mode MLEM reconstruction. Inverse Probl Sci Eng. 2021;29(13):3509-3529. doi: 10.1080/17415977.2021.2011863
- Zoglauer A, Kanbach G. Doppler broadening as a lower limit to the angular resolution of next-generation Compton telescopes. In: X-Ray and Gamma-Ray Telescopes and Instruments for Astronomy. Vol. 4851. Bellingham: SPIE; 2003. p. 1302-1309. doi: 10.1117/12.461177
- Brun R, Rademakers F. ROOT-An object oriented data analysis framework. Nucl Instrum Methods Phys Res A. 1997;389(1):81-86. doi: 10.1016/S0168-9002(97)00048-X
- Zhang H, Wang Y, Qi J, Abbaszadeh S. Penalized maximum-likelihood reconstruction for improving limited-angle artifacts in a dedicated head and neck PET system. Phys Med Biol. 2020;65(16):165016. doi: 10.1088/1361-6560/ab8c92
- Watts DP, Bordes J, Brown JR, et al. Photon quantum entanglement in the MeV regime and its application in PET imaging. Nat Commun. 2021;12(1):2646. doi: 10.1038/s41467-021-22907-5
- Kim D, Rachman AN, Taisei U, Uenomachi M, Shimazoe K, Takahashi H. Background reduction in PET by double Compton scattering of quantum entangled annihilation photons. J Inst. 2023;18(07):P07007. doi: 10.1088/1748-0221/18/07/P07007
- Kumar S, Chauhan SS, Bhatnagar V. Q-PET: PET with 3rd Eye Quantum Entanglement Based Positron Emission Tomography. In: Behera PK, Bhatnagar V, Shukla P, Sinha R, editors. XXIII DAE High Energy Physics Symposium. Germany: Springer Nature; 2021. p. 949-952. doi: 10.1007/978-981-33-4408-2_136
- Moskal P. Positronium and Quantum Entanglement Imaging: A New Trend in Positron Emission Tomography. In: 2021 IEEE Nuclear Science Symposium and Medical Imaging Conference (NSS/MIC); 2021. p. 1-3. doi: 10.1109/NSS/MIC44867.2021.9875524
- Ivashkin A, Abdurashitov D, Baranov A, et al. Testing entanglement of annihilation photons. Sci Rep. 2023; 13(1):7559. doi: 10.1038/s41598-023-34767-8