Understanding the link between immunosuppressant and natural killer cell in the progression of autism: The role of natural killer cell and mammalian target of rapamycin in autistic brain
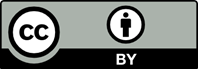
Natural killer cell plays a major role in the pathogenesis of neurodevelopmental disorder including autism spectrum disorder (ASD). ASD is a neurodevelopmental disorder that is associated with symptoms that reduce the quality of life of affected individuals such as social interaction deficit, cognitive impairment, intellectual disabilities, restricted, and repetitive behavioral patterns. This disorder begins in early childhood and continues throughout adulthood. ASD pathogenesis is associated with alteration in natural killer (NK) cells as well as mammalian target of rapamycin signaling functioning that leads to altered physiological processes during development. Here, we review literature highlighting the role of immunosuppressants especially rapamycin and azathioprine and their impact on treating the neurodevelopmental disorder and mechanism by which these drugs may act to ameliorate the autistic symptoms. Furthermore, this review discusses reports highlighting the role of NK cells and familial adenomatous polyposis gene in the etiology of ASD. This review suggests that further investigative work on these drugs could be vital in the management of ASD.
- Shen L, Liu X, Zhang H, et al., 2020, Biomarkers in autism spectrum disorders: Current progress. Clin Chim Acta, 502: 41–54. https://doi.org/10.1016/j.cca.2019.12.009
- Wang S, Liu H, Cheng B, et al., 2021, Vitamin A supplementation ameliorates motor incoordination via modulating RORα in the cerebellum in a valproic acid-treated rat autism model with Vitamin A deficiency. Neurotoxicology, 85: 90–98. https://doi.org/10.1016/j.neuro.2021.05.004
- McPartland J, Volkmar FR, 2012, Autism and related disorders. Handb Clin Neurol, 106: 407–418.
- Ashwood P, Wills S, Van de Water J, 2006, The immune response in autism: A new frontier for autism research. J Leukoc Biol, 80: 1–15. https://doi.org/10.1189/jlb.1205707
- Zeidan J, Fombonne E, Scorah J, et al., 2022, Global prevalence of autism: A systematic review update. Autism Res, 15: 778–790. https://doi.org/10.1002/aur.2696
- Al Dera H, 2022, Cellular and molecular mechanisms underlying autism spectrum disorders and associated comorbidities: A pathophysiological review. Biomed Pharmacother, 148: 112688. https://doi.org/10.1016/j.biopha.2022.112688
- Kumar S, Reynolds K, Ji Y, et al., 2019, Impaired neurodevelopmental pathways in autism spectrum disorder: A review of signaling mechanisms and crosstalk. J Neurodev Disord, 11: 10. https://doi.org/10.1186/s11689-019-9268-y
- Cezar LC, Kirsten TB, da Fonseca CC, et al., 2018, Zinc as a therapy in a rat model of autism prenatally induced by valproic acid. Prog Neuropsychopharmacol Biol Psychiatry 84: 173–180. https://doi.org/10.1016/j.pnpbp.2018.02.008
- Lacivita E, Perrone R, Margari L, et al., 2017, Targets for drug therapy for autism spectrum disorder: Challenges and future directions. J Med Chem, 60: 9114–9141. https://doi.org/10.1021/acs.jmedchem.7b00965
- He JL, Oeltzschner G, Mikkelsen M, et al., 2021, Region-specific elevations of glutamate + glutamine correlate with the sensory symptoms of autism spectrum disorders. Transl Psychiatry, 11: 411. https://doi.org/10.31234/osf.io/n4ujh
- Sharma A, Mehan S, 2021, Targeting PI3K-AKT/mTOR signaling in the prevention of autism. Neurochem Int, 147: 105067. https://doi.org/10.1016/j.neuint.2021.105067
- Van Gent T, Heijnen CJ, Treffers PD, 1997, Autism and the immune system. J Child Psychol Psychiatry, 38: 337–349. https://doi.org/10.1111/j.1469-7610.1997.tb01518.x
- Atladóttir HO, Pedersen MG, Thorsen P, et al., 2009, Association of family history of autoimmune diseases and autism spectrum disorders. Pediatrics, 124: 687–694. https://doi.org/10.1542/peds.2008-2445
- Croen LA, Grether JK, Yoshida CK, et al., 2005, Maternal autoimmune diseases, asthma and allergies, and childhood autism spectrum disorders: A case-control study. Arch Pediatr Adolesc Med, 159: 151–157. https://doi.org/10.1001/archpedi.159.2.151
- Careaga M, Van de Water J, Ashwood P, 2010, Immune dysfunction in autism: A pathway to treatment. Neurotherapeutics, 7: 283–292. https://doi.org/10.1016/j.nurt.2010.05.003
- Massrali A, Adhya D, Srivastava DP, et al., 2022, Virus-induced maternal immune activation as an environmental factor in the etiology of autism and schizophrenia. Front Neurosci, 16: 834058. https://doi.org/10.3389/fnins.2022.834058
- Caron A, Richard D, Laplante M, 2015, The roles of mTOR complexes in lipid metabolism. Annu Rev Nutr, 35: 321–348. https://doi.org/10.1146/annurev-nutr-071714-034355
- Sotgiu S, Manca S, Gagliano A, et al., 2020, Immune regulation of neurodevelopment at the mother-foetus interface: The case of autism. Clin Transl Immunol, 9: e1211. https://doi.org/10.1002/cti2.1211
- Malek M, Ashraf-Ganjouei A, Moradi K, et al., 2020, Prednisolone as adjunctive treatment to risperidone in children with regressive type of autism spectrum disorder: A randomized, placebo-controlled trial. Clin Neuropharmacol, 43: 39–45. https://doi.org/10.1097/wnf.0000000000000382
- Hollville E, Deshmukh M, 2018, Physiological functions of non-apoptotic caspase activity in the nervous system. Semin Cell Dev Biol, 82: 127–136. https://doi.org/10.1016/j.semcdb.2017.11.037
- Lawrie SM, Buechel C, Whalley HC, et al., 2022, Reduced frontotemporal functional connectivity in schizophrenia associated with auditory hallucinations. Biol Psychiatry, 51: 1008–1011. https://doi.org/10.1016/s0006-3223(02)01316-1
- Thomas MS, Davis R, Karmiloff-Smith A, et al., 2015, The over-pruning hypothesis of autism. Dev Sci, 19: 1–22.
- Morales-Torres S, 2022, Study on Sub-lethal Executioner Caspase Activation in the Mammalian Nervous System. Oakland: University of California.
- Goto M, Tanimoto K, Horiuchi Y, 1980, Natural cell mediated cytotoxicity in systemic lupus erythematosus. Arthritis Rheum, 23: 1274–1281. https://doi.org/10.1002/art.1780231108
- Waksman BH, 1981, Current trends in multiple sclerosis research. Immunol Today, 2: 87–93. https://doi.org/10.1016/0167-5699(81)90038-4
- Goto M, Tanimoto K, Chihara T, et al., 1981, Natural cell-mediated cytotoxicity in Sjögren’s syndrome and rheumatoid arthritis. Arthritis Rheum, 24: 1377–1382. https://doi.org/10.1002/art.1780241107
- García-Domínguez I, Suárez-Pereira I, Santiago M, et al., 2021, Selective deletion of Caspase-3 gene in the dopaminergic system exhibits autistic-like behaviour. Prog Neuropsychopharmacol Biol Psychiatry, 104: 110030. https://doi.org/10.1016/j.pnpbp.2020.110030
- Warren RP, Foster A, Margaretten NC, 1987, Reduced natural killer cell activity in autism. J Am Acad Child Adolesc Psychiatry, 26: 333–335. https://doi.org/10.1097/00004583-198705000-00008
- Meimand SE, Rostam-Abadi Y, Rezaei N, 2021, autism spectrum disorders and natural killer cells: A review on pathogenesis and treatment. Expert Rev Clin Immunol, 17: 27–35. https://doi.org/10.1080/1744666x.2020.1850273
- Ganesan H, Balasubramanian V, Iyer M, et al., 2019, mTOR signalling pathway-A root cause for idiopathic autism? BMB Rep, 52: 424–433. https://doi.org/10.5483/bmbrep.2019.52.7.137
- Hutsler JJ, Zhang H, 2010, Increased dendritic spine densities on cortical projection neurons in autism spectrum disorders. Brain Res, 1309: 83–94. https://doi.org/10.1016/j.brainres.2009.09.120
- Winden KD, Ebrahimi-Fakhari D, Sahin M, 2018, Abnormal mTOR activation in autism. Annu Rev Neurosci, 41: 1–23. https://doi.org/10.1146/annurev-neuro-080317-061747
- Zhang B, Wong M, 2012, Pentylenetetrazole‐induced seizures cause acute, but not chronic, mTOR pathway activation in rat. Epilepsia, 53: 506–511. https://doi.org/10.1111/j.1528-1167.2011.03384.x
- Gatto CL, Broadie K, 2010, Genetic controls balancing excitatory and inhibitory synaptogenesis in neurodevelopmental disorder models. Front Synaptic Neurosci, 2: 4. https://doi.org/10.3389/fnsyn.2010.00004
- Won H, Mah W, Kim E, 2013, autism spectrum disorder causes, mechanisms, and treatments: Focus on neuronal synapses. Front Mol Neurosci, 6: 19. https://doi.org/10.3389/fnmol.2013.00019
- Lin TV, Hsieh L, Kimura T, et al., 2016, Normalizing translation through 4E-BP prevents mTOR-driven cortical mislamination and ameliorates aberrant neuron integration. Proc Natl Acad Sci U S A, 113: 11330–11335. https://doi.org/10.1073/pnas.1605740113
- Tylee DS, Espinoza AJ, Hess JL, et al., 2017, RNA sequencing of transformed lymphoblastoid cells from siblings discordant for autism spectrum disorders reveals transcriptomic and functional alterations: Evidence for sex-specific effects. Autism Res, 10: 439–455. https://doi.org/10.1002/aur.1679
- Kassai H, Sugaya Y, Noda S, et al., 2014, Selective activation of mTORC1 signaling recapitulates microcephaly, tuberous sclerosis, and neurodegenerative diseases. Cell Rep, 7: 1626–1639. https://doi.org/10.1016/j.celrep.2014.04.048
- Magri L, Cambiaghi M, Cominelli M, et al., 2011, Sustained activation of mTOR pathway in embryonic neural stem cells leads to development of tuberous sclerosis complex-associated lesions. Cell Stem Cell, 9: 447–462. https://doi.org/10.1016/j.stem.2011.09.008
- Jernigan CS, Goswami DB, Austin MC, et al., 2011, The mTOR signaling pathway in the prefrontal cortex is compromised in major depressive disorder. Prog Neuropsychopharmacol Biol Psychiatry, 35: 1774–1779. https://doi.org/10.1016/j.pnpbp.2011.05.010
- Talos DM, Sun H, Zhou X, et al., 2012, The interaction between early life epilepsy and autistic-like behavioral consequences: A role for the mammalian target of rapamycin (mTOR) pathway. PLoS One, 7: e35885. https://doi.org/10.1371/journal.pone.0035885
- Buckmaster PS, Ingram EA, Wen X, 2009, Inhibition of the mammalian target of rapamycin signaling pathway suppresses dentate granule cell axon sprouting in a rodent model of temporal lobe epilepsy. J Neurosci, 29: 8259–8269. https://doi.org/10.1523/jneurosci.4179-08.2009
- Onore C, Yang H, Van de Water J, et al., 2017, Dynamic Akt/ mTOR signaling in children with autism spectrum disorder. Front Pediatr, 5: 43. https://doi.org/10.3389/fped.2017.00043
- Neniskyte U, Gross CT, 2017, Errant gardeners: Glial-cell-dependent synaptic pruning and neurodevelopmental disorders. Nat Rev Neurosci, 18: 658–670. https://doi.org/10.1038/nrn.2017.110
- Wong M, 2019, The role of glia in epilepsy, intellectual disability, and other neurodevelopmental disorders in tuberous sclerosis complex. J Neurodev Disord, 11: 30. https://doi.org/10.1186/s11689-019-9289-6
- Yui K, Sato A, Imataka G, 2015, Mitochondrial dysfunction and its relationship with mTOR signaling and oxidative damage in autism spectrum disorders. Mini Rev Med Chem, 15: 373–389. https://doi.org/10.2174/1389557515666150324122930
- Leite JA, Orellana AM, Kinoshita PF, et al., 2017, Neuroinflammation and neurotransmission mechanisms involved in neuropsychiatric disorders. In: Mechanisms of Neuroinflammation. London: IntechOpen. https://doi.org/10.5772/intechopen.69343
- Andrews MG, Subramanian L, Kriegstein AR, 2020, mTOR signaling regulates the morphology and migration of outer radial glia in developing human cortex. Elife, 9: e58737. https://doi.org/10.7554/elife.58737
- Da Silva Vaccaro T, Sorrentino JM, Salvador S, et al., 2018, Alterations in the microRNA of the blood of autism spectrum disorder patients: Effects on epigenetic regulation and potential biomarkers. Behav Sci (Basel), 8: 75. https://doi.org/10.3390/bs8080075
- Gevezova M, Sarafian V, Anderson G, et al., 2020, Inflammation and mitochondrial dysfunction in autism spectrum disorder. CNS Neurol Disord Drug Targets, 19: 320–333. https://doi.org/10.2174/1871527319666200628015039
- Meltzer A, Van de Water J, 2017, The role of the immune system in autism spectrum disorder. Neuropsychopharmacology, 42: 284–298. https://doi.org/10.1038/npp.2016.158
- Gilmore JH, Jarskog LF, Vadlamudi S, 2005, Maternal poly I: C exposure during pregnancy regulates TNF alpha, BDNF, and NGF expression in neonatal brain and the maternal-fetal unit of the rat. J Neuroimmunol, 159: 106–112. https://doi.org/10.1016/j.jneuroim.2004.10.008
- Meyer U, Feldon J, Schedlowski M, et al., 2005, Towards an immuno-precipitated neurodevelopmental animal model of schizophrenia. Neurosci Biobehav Rev, 29: 913–947. https://doi.org/10.1016/j.neubiorev.2004.10.012
- Urakubo A, Jarskog LF, Lieberman JA, et al., 2001, Prenatal exposure to maternal infection alters cytokine expression in the placenta, amniotic fluid, and fetal brain. Schizophr Res, 47: 27–36. https://doi.org/10.1016/s0920-9964(00)00032-3
- Zuckerman L, Weiner I, 2005, Maternal immune activation leads to behavioral and pharmacological changes in the adult offspring. J Psychiatr Res, 39: 311–323. https://doi.org/10.1016/j.jpsychires.2004.08.008
- Shi L, Fatemi SH, Sidwell RW, et al., 2003, Maternal influenza infection causes marked behavioral and pharmacological changes in the offspring. J Neurosci, 23: 297–302. https://doi.org/10.1523/jneurosci.23-01-00297.2003
- Chez MG, Guido-Estrada N, 2010, Immune therapy in autism: Historical experience and future directions with immunomodulatory therapy. Neurotherapeutics, 7: 293–301. https://doi.org/10.1016/j.nurt.2010.05.008
- Trifonova EA, Klimenko AI, Mustafin ZS, et al., 2021, Do autism spectrum and autoimmune disorders share predisposition gene signature due to mTOR signaling pathway controlling expression? Int J Mol Sci, 22: 5248. https://doi.org/10.3390/ijms22105248
- Wu S, Ding Y, Wu F, et al., 2015, Family history of autoimmune diseases is associated with an increased risk of autism in children: A systematic review and meta-analysis. Neurosci Biobehav Rev, 55: 322–332. https://doi.org/10.1016/j.neubiorev.2015.05.004
- Rudolph MD, Graham AM, Feczko E, et al., 2018, Maternal IL-6 during pregnancy can be estimated from newborn brain connectivity and predicts future working memory in offspring. Nat Neurosci, 21: 765–772. https://doi.org/10.1038/s41593-018-0128-y
- Rasmussen JM, Graham AM, Entringer S, et al., 2019, Maternal Interleukin-6 concentration during pregnancy is associated with variation in frontolimbic white matter and cognitive development in early life. Neuroimage, 185: 825–835. https://doi.org/10.1016/j.neuroimage.2018.04.020
- Hornig M, Bresnahan MA, Che X, et al., 2018, Prenatal fever and autism risk. Mol Psychiatry, 23: 759–766. https://doi.org/10.1038/mp.2017.119
- Steinman L, Conlon P, Maki R, et al., 2003, The intricate interplay among body weight, stress, and the immune response to friend or foe. J Clin Invest, 111: 183–185. https://doi.org/10.1172/jci200317622
- Tracey KJ, Wei H, Manogue KR, et al., 1988, Cachectin/ tumor necrosis factor induces cachexia, anemia, and inflammation. J Exp Med, 167: 1211–12127. https://doi.org/10.1084/jem.167.3.1211
- Glantz LA, Gilmore JH, Lieberman JA, et al., 2006, Apoptotic mechanisms and the synaptic pathology of schizophrenia. Schizophr Res, 81: 47–63. https://doi.org/10.1016/j.schres.2005.08.014
- Verma V, Paul A, Vishwanath AA, et al., 2019, Understanding intellectual disability and autism spectrum disorders from common mouse models: Synapses to behaviour. Open Biol, 9: 180265. https://doi.org/10.1098/rsob.180265
- Hyman BT, Yuan J, 2012, Apoptotic and non-apoptotic roles of caspases in neuronal physiology and pathophysiology. Nat Rev Neurosci, 13: 395–406. https://doi.org/10.1038/nrn3228
- Suárez-Pereira I, García-Domínguez I, Bravo L, et al., 2022, The absence of caspase-8 in the dopaminergic system leads to mild autism-like behavior. Front Cell Dev Biol, 10: 839715. https://doi.org/10.3389/fcell.2022.1040623
- El-Ansary A, Al-Ayadhi L, 2012, Neuroinflammation in autism spectrum disorders. J Neuroinflamm, 9: 265. https://doi.org/10.1186/1742-2094-9-265
- El-Ansary AK, Ben Bacha AG, Al-Ayadhi LY, 2011, Proinflammatory and proapoptotic markers in relation to mono and di-cations in plasma of autistic patients from Saudi Arabia. J Neuroinflamm, 8: 142. https://doi.org/10.1186/1742-2094-8-142
- Paulsen M, Ussat S, Jakob M, et al., 2008, Interaction with XIAP prevents full caspase-3/-7 activation in proliferating human T lymphocytes. Eur J Immunol, 38: 1979–1987. https://doi.org/10.1002/eji.200838211
- Bauernfeind F, Ablasser A, Bartok E, et al., 2011, Inflammasomes: Current understanding and open questions. Cell Mol Life Sci, 68: 765–783. https://doi.org/10.1007/s00018-010-0567-4
- Ruiz-Vela A, Opferman JT, Cheng EH, et al., 2005, Proapoptotic BAX and BAK control multiple initiator caspases. EMBO Rep, 6: 379–385. https://doi.org/10.1038/sj.embor.7400375
- Siniscalco D, Sapone A, Giordano C, et al., 2012, The expression of caspases is enhanced in peripheral blood mononuclear cells of autism spectrum disorder patients. J Autism Dev Disord, 42: 1403–1410. https://doi.org/10.1007/s10803-011-1373-z
- Zhang YQ, Lin WP, Huang LP, et al., 2021, Dopamine D2 receptor regulates cortical synaptic pruning in rodents. Nat Commun, 12: 6444. https://doi.org/10.1038/s41467-021-26769-9
- Zajicek AS, Ruan H, Dai H, et al., 2022, Cylindromatosis drives synapse pruning and weakening by promoting macroautophagy through Akt-mTOR signaling. Mol Psychiatry, 27: 2414–2424. https://doi.org/10.1038/s41380-022-01571-1
- Kelleher RJ, Bear MF, 2008, The autistic neuron: Troubled translation? Cell, 135: 401–406. https://doi.org/10.1016/j.cell.2008.10.017
- Kim HJ, Cho MH, Shim WH, et al., 2017, Deficient autophagy in microglia impairs synaptic pruning and causes social behavioral defects. Mol Psychiatry, 22: 1576–1584. https://doi.org/10.1038/mp.2016.103
- Ma T, Hoeffer CA, Capetillo-Zarate E, et al., Dysregulation of the mTOR pathway mediates impairment of synaptic plasticity in a mouse model of Alzheimer’s disease. PLoS One, 5: e12845. https://doi.org/10.1371/journal.pone.0012845
- Meng XF, Yu JT, Song JH, et al., 2013, Role of the mTOR signaling pathway in epilepsy. J Neurol Sci, 332: 4–15.
- Trowsdale J, Barten R, Haude A, et al., 2001, The genomic context of natural killer receptor extended gene families. Immunol Rev, 181: 20–38. https://doi.org/10.1034/j.1600-065x.2001.1810102.x
- Patterson PH, 2011, Maternal infection and immune involvement in autism. Trends Mol Med, 17: 389–394. https://doi.org/10.1016/j.molmed.2011.03.001
- Enstrom AM, Lit L, Onore CE, et al., 2009, Altered gene expression and function of peripheral blood natural killer cells in children with autism. Brain Behav Immun, 23: 124–133. https://doi.org/10.1016/j.bbi.2008.08.001
- Torres AR, Westover JB, Gibbons C, et al., 2012, Activating killer-cell immunoglobulin-like receptors (KIR) and their cognate HLA ligands are significantly increased in autism. Brain Behav Immun, 26: 1122–1127. https://doi.org/10.1016/j.bbi.2012.07.014
- Blanco K, Radoff GP, 2007, Lyme disease and autism a new paradigm. Townsend letter: Exam Alternat Med, 285: 78–82.
- Boulanger LM, 2009, Immune proteins in brain development and synaptic plasticity. Neuron, 64: 93–109. https://doi.org/10.1016/j.neuron.2009.09.001
- Garay PA, McAllister AK, 2010, Novel roles for immune molecules in neural development: implications for neurodevelopmental disorders. Front Synaptic Neurosci, 2: 136. https://doi.org/10.3389/fnsyn.2010.00136
- Yin J, Valin KL, Dixon ML, et al., 2017, The role of microglia and macrophages in CNS homeostasis, autoimmunity, and cancer. J Immunol Res, 2017: 5150678. https://doi.org/10.1155/2017/5150678
- Onore C, Careaga M, Ashwood P, 2012, The role of immune dysfunction in the pathophysiology of autism. Brain Behav Immun, 26: 383–392. https://doi.org/10.1016/j.bbi.2011.08.007
- Penzes P, Cahill ME, Jones KA, et al., 2011, Dendritic spine pathology in neuropsychiatric disorders. Nat Neurosci, 14: 285–293. https://doi.org/10.1038/nn.2741
- Masi A, Glozier N, Dale R, et al., 2017, The immune system, cytokines, and biomarkers in autism spectrum disorder. Neurosci Bull, 33: 194–204. https://doi.org/10.1007/s12264-017-0103-8
- Gibney SM, Drexhage HA, 2013, Evidence for a dysregulated immune system in the etiology of psychiatric disorders. J Neuroimmune Pharmacol, 8: 900–920. https://doi.org/10.1007/s11481-013-9462-8
- Vojdani A, Mumper E, Granpeesheh D, et al., 2008, Low natural killer cell cytotoxic activity in autism: The role of glutathione, IL-2 and IL-15. J Neuroimmunol, 205: 148–154. https://doi.org/10.1016/j.jneuroim.2008.09.005
- Siniscalco D, Mijatovic T, Bosmans E, et al., 2016, Decreased numbers of CD57+CD3- cells identify potential innate immune differences in patients with autism spectrum disorder. In vivo, 30: 83–89.
- Bjørklund G, Meguid NA, El-Bana MA, et al., 2020, Oxidative stress in autism spectrum disorder. Mol Neurobiol, 57: 2314–2332.
- Matelski L, Van de Water J, 2016, Risk factors in autism: Thinking outside the brain. J Autoimmun, 67: 1–7. https://doi.org/10.1016/j.jaut.2015.11.003
- Yirmiya R, Goshen I, 2011, Immune modulation of learning, memory, neural plasticity and neurogenesis. Brain Behav Immun, 25: 181–213. https://doi.org/10.1016/j.bbi.2010.10.015
- Zou R, Xu F, Wang Y, et al., 2020, Changes in the gut microbiota of children with autism spectrum disorder. Autism Res, 13: 1614–1625. https://doi.org/10.1002/aur.2358
- Mulle JG, Sharp WG, Cubells JF, 2013, The gut microbiome: A new frontier in autism research. Curr Psychiatry Rep, 15: 337. https://doi.org/10.1007/s11920-012-0337-0
- Illiano P, Brambilla R, Parolini C, 2020, The mutual interplay of gut microbiota, diet and human disease. FEBS J, 287: 833–855. https://doi.org/10.1111/febs.15217
- Chung J, Kuo CJ, Crabtree GR, et al., 1992, Rapamycin- FKBP specifically blocks growth-dependent activation of and signaling by the 70 kd S6 protein kinases. Cell, 69: 1227–1236. https://doi.org/10.1016/0092-8674(92)90643-q
- Price DJ, Grove JR, Calvo V, et al., 1992, Rapamycin-induced inhibition of the 70-kilodalton S6 protein kinase. Science, 257: 973–977. https://doi.org/10.1126/science.1380182
- Li J, Kim SG, Blenis J, 2014, Rapamycin: One drug, many effects. Cell Metab, 19: 373–379. https://doi.org/10.1016/j.cmet.2014.01.001
- Muncy J, Butler IJ, Koenig MK, 2009, Rapamycin reduces seizure frequency in tuberous sclerosis complex. J Child Neurol, 24: 477–477. https://doi.org/10.1177/0883073808324535
- Sato A, Kasai S, Kobayashi T, et al., 2012, Rapamycin reverses impaired social interaction in mouse models of tuberous sclerosis complex. Nat Commun, 3: 1292. https://doi.org/10.1038/ncomms2295
- Chen J, Alberts I, Li X, 2014, Dysregulation of the IGF-I/ PI3K/AKT/mTOR signaling pathway in autism spectrum disorders. Int J Dev Neurosci, 35: 35–41. https://doi.org/10.1016/j.ijdevneu.2014.03.006
- Eden KE, de Vries PJ, Moss J, et al., 2014, Self-injury and aggression in tuberous sclerosis complex: Cross syndrome comparison and associated risk markers. J Neurodev Disord, 6: 10. https://doi.org/10.1186/1866-1955-6-10
- Tsai PT, Hull C, Chu Y, et al., 2012, Autistic-like behaviour and cerebellar dysfunction in Purkinje cell Tsc1 mutant mice. Nature, 488: 647–651. https://doi.org/10.1038/nature11310
- Curatolo P, Moavero R, de Vries PJ, 2015, Neurological and neuropsychiatric aspects of tuberous sclerosis complex. Lancet Neurol, 14: 733–745. https://doi.org/10.1016/s1474-4422(15)00069-1
- Tang G, Gudsnuk K, Kuo SH, et al., 2014, Loss of mTOR-dependent macroautophagy causes autistic-like synaptic pruning deficits. Neuron, 83: 1131–1143. https://doi.org/10.1016/j.neuron.2014.07.040
- Meimand SE, Rostam-Abadi Y, Rezaei N, 2020, autism spectrum disorders and natural killer cells: A review on pathogenesis and treatment. Expert Rev Clin Immunol, 17: 27-35. https://doi.org/10.1080/1744666x.2020.1850273
- Marçais A, Marotel M, Degouve S, et al., 2017, High mTOR activity is a hallmark of reactive natural killer cells and amplifies early signaling through activating receptors. Elife, 6: e26423. https://doi.org/10.7554/elife.26423
- Marçais A, Cherfils-Vicini J, Viant C, et al., 2014, The metabolic checkpoint kinase mTOR is essential for IL-15 signaling during the development and activation of NK cells. Nat Immunol, 15: 749–757. https://doi.org/10.1038/ni.2936
- Nandagopal N, Ali AK, Komal AK, et al., 2014, The critical role of IL-15-PI3K-mTOR pathway in natural killer cell effector functions. Front Immunol, 5: 187. https://doi.org/10.3389/fimmu.2014.00187
- Ali AK, Nandagopal N, Lee SH, 2015, IL-15-PI3K-AKT-mTOR: A critical pathway in the life journey of natural killer cells. Front Immunol, 6: 355. https://doi.org/10.3389/fimmu.2015.00355
- Mah AY, Cooper MA, 2016, Metabolic regulation of natural killer cell IFN-γ production. Crit Rev Immunol, 36: 131–147. https://doi.org/10.1615/critrevimmunol.2016017387
- Wai LE, Fujiki M, Takeda S, et al., 2008, Rapamycin, but not cyclosporine or FK506, alters natural killer cell function. Transplantation, 85: 145–149. https://doi.org/10.1097/01.tp.0000296817.28053.7b
- Kumar S, Mehan S, Narula AS, 2022, Therapeutic modulation of JAK-STAT, mTOR, and PPAR-γ signaling in neurological dysfunctions. J Mol Med, 101: 1–41. https://doi.org/10.1007/s00109-022-02272-6
- Dreyer C, Krey G, Keller H, et al., 1992, Control of the peroxisomal beta-oxidation pathway by a novel family of nuclear hormone receptors. Cell, 68: 879–887. https://doi.org/10.1016/0092-8674(92)90031-7
- Kwan V, Unda BK, Singh KK, 2016, Wnt signaling networks in autism spectrum disorder and intellectual disability. J Neurodev Disord, 8: 45. https://doi.org/10.1186/s11689-016-9176-3
- Caracci MO, Ávila ME, De Ferrari GV, 2016, Synaptic Wnt/GSK3β signaling hub in autism. Neural Plast, 2016: 9603751. https://doi.org/10.1155/2016/9603751
- Wei J, Bhattacharyya S, Tourtellotte WG, et al., 2011, Fibrosis in systemic sclerosis: Emerging concepts and implications for targeted therapy. Autoimmun Rev, 10: 267–275. https://doi.org/10.1016/j.autrev.2010.09.015
- Ricote M, Glass CK, 2007, PPARs and molecular mechanisms of transrepression. Biochim Biophys Acta, 1771: 926–935. https://doi.org/10.1016/j.bbalip.2007.02.013
- Bussey H, 1975, Familial Polyposis Coli. Family Studies, Histopathology, Differential Diagnosis, and Results of Treatment. United States: The John Hopkins University Press. p 47–49. https://doi.org/10.7326/0003-4819-83-6-924_1
- Nieuwenhuis MH, Vasen HF, 2007, Correlations between mutation site in APC and phenotype of familial adenomatous polyposis (FAP): A review of the literature. Crit Rev Oncol Hematol, 61: 153–161. https://doi.org/10.1016/j.critrevonc.2006.07.004
- Nishisho I, Nakamura Y, Miyoshi Y, et al., 1991, Mutations of chromosome 5q21 genes in FAP and colorectal cancer patients. Science, 253: 665–669. https://doi.org/10.1126/science.1651563
- Kinzler KW, Nilbert MC, Su LK, et al., 1991, Identification of FAP locus genes from chromosome 5q21. Science, 253: 661–665. https://doi.org/10.1126/science.1651562
- Goss KH, Groden J, 2000, Biology of the adenomatous polyposis coli tumor suppressor. J Clin Oncol, 18: 1967–1979. https://doi.org/10.1200/jco.2000.18.9.1967
- Sieber OM, Tomlinson IP, Lamlum H, 2000, The adenomatous polyposis coli (APC) tumour suppressor-- genetics, function and disease. Mol Med Today, 6: 462–469. https://doi.org/10.1016/s1357-4310(00)01828-1
- Maffini V, Fornaroli F, Vincenzi F, et al., 2008, Autism and gastrointestinal disorders: A close relationship? Dig Liver Dis, 40: A93. https://doi.org/10.1016/j.dld.2008.07.265
- Zhou XL, Giacobini M, Anderlid BM, et al., 2007, Association of adenomatous polyposis coli (APC) gene polymorphisms with autism spectrum disorder (ASD). Am J Med Genet B Neuropsychiatr Genet, 144B: 351–354. https://doi.org/10.1002/ajmg.b.30415
- Reggio A, Spada F, Rosina M, et al., 2019, The immunosuppressant drug azathioprine restrains adipogenesis of muscle Fibro/Adipogenic Progenitors from dystrophic mice by affecting AKT signaling. Sci Rep, 9: 4360. https://doi.org/10.1038/s41598-019-39538-y
- Mozzetta C, Consalvi S, Saccone V, et al., 2003, Fibroadipogenic progenitors mediate the ability of HDAC inhibitors to promote regeneration in dystrophic muscles of young, but not old Mdx mice. EMBO Mol Med, 5: 626–639. https://doi.org/10.1002/emmm.201202096
- Maltzman JS, Koretzky GA, 2003, Azathioprine: Old drug, new actions. J Clin Investig, 111: 1122–1124. https://doi.org/10.1172/jci200318384
- Elion GB, Hitchings GH, 1975, Azathioprine, in Antineoplastic and Immunosuppressive Agents. Berlin: Springer. p 404–425. https://doi.org/10.1007/978-3-642-65806-8_19
- Aarbakke J, Janka-Schaub G, Elion GB, 1997, Thiopurine biology and pharmacology. Trends Pharmacol Sci, 18: 3–7. https://doi.org/10.1016/s0165-6147(96)01007-3
- Elion GB, 1993, The george hitchings and gertrude elion lecture. The pharmacology of azathioprine. Ann N Y Acad Sci, 685: 400–407. https://doi.org/10.1111/j.1749-6632.1993.tb35896.x
- Huang HY, Chang HF, Tsai MJ, et al., 2016, 6-Mercaptopurine attenuates tumor necrosis factor-α production in microglia through Nur77-mediated transrepression and PI3K/Akt/mTOR signaling-mediated translational regulation. J Neuroinflamm, 13: 78. https://doi.org/10.1186/s12974-016-0543-5
- Hooper KM, Casanova V, Kemp S, et al., 2019, The inflammatory bowel disease drug azathioprine induces autophagy via mTORC1 and the unfolded protein response sensor PERK. Inflamm Bowel Dis, 25: 1481–1496. https://doi.org/10.1093/ibd/izz039
- Green MR, Kennell AS, Larche MJ, et al., 2005, Natural killer cell activity in families of patients with systemic lupus erythematosus: Demonstration of a killing defect in patients. Clin Exp Immunol, 141: 165–173. https://doi.org/10.1111/j.1365-2249.2005.02822.x
- Ehninger D, Silva AJ, 2011, Rapamycin for treating Tuberous sclerosis and autism spectrum disorders. Trends Mol Med, 17: 78–87. https://doi.org/10.1016/j.molmed.2010.10.002
- Pithadia AB, Jain S, 2011, Treatment of inflammatory bowel disease (IBD). Pharmacol Rep, 63: 629–642. https://doi.org/10.1016/s1734-1140(11)70575-8
- McWilliam M, Khan U, 2020, Azathioprine and the neurologist. Pract Neurol, 20: 69–74.
- Viana SD, Reis F, Alves R, 2018, Therapeutic use of mTOR inhibitors in renal diseases: Advances, drawbacks, and challenges. Oxid Med Cell Longev, 2018: 3693625. https://doi.org/10.1155/2018/3693625
- Jeong A, Wong M, 2016, mTOR inhibitors in children: Current indications and future directions in neurology. Curr Neurol Neurosci Rep, 16: 102. https://doi.org/10.1007/s11910-016-0708-8
- Berry-Kravis E, Sumis A, Hervey C, et al., 2008, Open-label treatment trial of lithium to target the underlying defect in fragile X syndrome. J Dev Behav Pediatr, 29: 293–302. https://doi.org/10.1097/dbp.0b013e31817dc447
- Paribello C, Tao L, Folino A, et al., 2010, Open-label add-on treatment trial of minocycline in fragile X syndrome. BMC Neurol, 10: 91. https://doi.org/10.1186/1471-2377-10-91
- Ghosh A, Michalon A, Lindemann L, et al., 2013, Drug discovery for autism spectrum disorder: Challenges and opportunities. Nat Rev Drug Discov, 12: 777–790. https://doi.org/10.1038/nrd4102
- Hasan MS, Ryan PL, Bergmeier LA, et al., 2017, Circulating NK cells and their subsets in Behçet’s disease. Clin Exp Immunol, 188: 311–322. https://doi.org/10.1111/cei.12939
- Prince HE, Ettenger RB, Dorey FJ, et al., 1984, Azathioprine suppression of natural killer activity and antibody-dependent cellular cytotoxicity in renal transplant recipients. J Clin Immunol, 4: 312–318. https://doi.org/10.1007/bf00915299
- Kucuksezer UC, Cetin EA, Esen F, et al., 2021, The role of natural killer cells in autoimmune diseases. Front Immunol, 12: 622306.
- Nguyen S, Morel V, Le Garff-Tavernier M, et al., 2006, Persistence of CD16+/CD56-/2B4+ natural killer cells: A highly dysfunctional NK subset expanded in ocular myasthenia gravis. J Neuroimmunol, 179: 117–125. https://doi.org/10.1016/j.jneuroim.2006.05.028
- Nived O, Johansson I, Sturfelt G, 1992, Effects of ultraviolet irradiation on natural killer cell function in systemic lupus erythematosus. Ann Rheum Dis, 51: 726–730. https://doi.org/10.1136/ard.51.6.726
- McCracken JT, Anagnostou E, Arango C, et al., 2021, Drug development for autism spectrum disorder (ASD): Progress, challenges, and future directions. Eur Neuropsychopharmacol, 48: 3–31. https://doi.org/10.1016/j.euroneuro.2021.05.010