Neurodevelopmental disorders and the role of PSD-95: Understanding pathways and pharmacological interventions
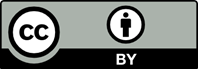
Neurodevelopmental disorders (NDDs) are often linked to disruption in brain development and present challenges for affected individuals in achieving their cognitive, emotional, and motor developmental milestones. NDDs encompass a spectrum of conditions, including autism spectrum disorder (ASD), schizophrenia (SCZ), attention-deficit hyperactivity disorder (ADHD), and epilepsy. The unequivocal diagnosis of an NDD is often challenging due to overlapping signs and symptoms across different conditions. Synaptic plasticity, the activity-driven modification of synaptic strength and efficacy, plays a crucial role in brain network formation and organization and is frequently altered in NDDs. Here, we explore the multifaceted roles of postsynaptic density-95 kDa (PSD-95) in NDDs. Psd-95 is a scaffolding protein belonging to the membrane-associated guanylate kinases (MAGUKs) family, located at the core of synapses, and is central to synaptic plasticity. Dysregulation of PSD-95 is linked to various neuropsychiatric disorders. In SCZ, decreased PSD-95 expression affects synaptic plasticity, thereby impacting learning and memory. Genes associated with ASD interact with PSD-95, and its removal in mice leads to ASD-like behavioral abnormalities. Furthermore, PSD-95 is implicated in ADHD, where its modulation influences neurotransmission. Medications used in NDD treatment, such as antipsychotic drugs and selective serotonin reuptake inhibitors (SSRIs), can alter PSD-95 levels, potentially influencing synapse formation. Alpha-2 adrenergic agonists might enhance synaptic integrity by impacting PSD-95. Alternative pharmacotherapies such as memantine, allopurinol, and ketamine, all influencing PSD-95 to a certain extent, hold promise in managing NDDs. Understanding the role of PSD-95 in these disorders can deepen our biological comprehension and pave the way for targeted therapies. Specifically, exploring how PSD-95 affects synaptic plasticity and dendritic spine development could uncover opportunities for repurposing drugs to treat NDDs associated with mutations in the DLG4 gene encoding PSD-95.
- Tărlungeanu DC, Novarino G. Genomics in neurodevelopmental disorders: An avenue to personalized medicine. Exp Mol Med. 2018;50(8):1-7. doi: 10.1038/s12276-018-0129-7
- Gilissen C, Hehir-Kwa JY, Thung DT, et al. Genome sequencing identifies major causes of severe intellectual disability. Nature. 2014;511(7509):344-347. doi: 10.1038/nature13394
- Mullin AP, Gokhale A, Moreno-De-Luca A, Sanyal S, Waddington JL, Faundez V. Neurodevelopmental disorders: Mechanisms and boundary definitions from genomes, interactomes and proteomes. Transl Psychiatry. 2013;3(12):e329. doi: 10.1038/tp.2013.108
- Lesch KP, Waider J. Serotonin in the modulation of neural plasticity and networks: Implications for neurodevelopmental disorders. Neuron. 2012;76(1):175-191. doi: 10.1016/j.neuron.2012.09.013
- Zoghbi HY, Bear MF. Synaptic dysfunction in neurodevelopmental disorders associated with autism and intellectual disabilities. Cold Spring Harb Perspect Biol. 2012;4(3):a009886. doi: 10.1101/cshperspect.a009886
- Turrigiano GG, Nelson SB. Homeostatic plasticity in the developing nervous system. Nat Rev Neurosci. 2004;5(2):97-107. doi: 10.1038/nrn1327
- Citri A, Malenka RC. Synaptic plasticity: Multiple forms, functions, and mechanisms. Neuropsychopharmacol. 2008;33(1):18-41. doi: 10.1038/sj.npp.1301559
- Engert F, Bonhoeffer T. Dendritic spine changes associated with hippocampal long-term synaptic plasticity. Nature. 1999;399(6731):66-70. doi: 10.1038/19978
- Bliss TVP, Collingridge GL. A synaptic model of memory: Long-term potentiation in the hippocampus. Nature. 1993;361(6407):31-39. doi: 10.1038/361031a0
- Malinow R, Schulman H, Tsien RW. Inhibition of postsynaptic PKC or CaMKII blocks induction but not expression of LTP. Science. 1989;245(4920):862-866. doi: 10.1126/science.2549638
- Coley AA, Gao WJ. PSD-95 deficiency disrupts PFC-associated function and behavior during neurodevelopment. Sci Rep. 2019;9(1):9486.
- Won S, Levy JM, Nicoll RA, Roche KW. MAGUKs: Multifaceted synaptic organizers. Curr Opin Neurobiol. 2017;43:94-101. doi: 10.1016/j.conb.2017.01.006
- Kim E, Sheng M. PDZ domain proteins of synapses. Nat Rev Neurosci. 2004;5(10):771-781. doi: 10.1038/nrn1517
- Zhang H, Etherington LA, Hafner AS, et al. Regulation of AMPA receptor surface trafficking and synaptic plasticity by a cognitive enhancer and antidepressant molecule. Mol Psychiatry. 2013;18(4):471-484. doi: 10.1038/mp.2012.80.
- Mujtaba T, Mayer-Proschel M, Rao M. A common neural progenitor for the CNS and PNS. Dev Biol. 1998;200(1):1-15. doi: 10.1006/dbio.1998.8913
- Coley AA, Gao WJ. PSD95: A synaptic protein implicated in schizophrenia or autism? Prog Neuropsychopharmacol Biol Psychiatry. 2018;82:187-194. doi: 10.1016/j.pnpbp.2017.11.016
- Kaizuka T, Takumi T. Postsynaptic density proteins and their involvement in neurodevelopmental disorders. J Biochem. 2018;163(6):447-455. doi: 10.1093/jb/mvy022
- Clinton SM, Haroutunian V, Meador‐Woodruff JH. Up‐regulation of NMDA receptor subunit and post‐synaptic density protein expression in the thalamus of elderly patients with schizophrenia. J Neurochem. 2006;98(4):1114-1125. doi: 10.1111/j.1471-4159.2006.03954.x
- Hosy E, Butler C, Sibarita JB. Organization and dynamics of AMPA receptors inside synapses-nano-organization of AMPA receptors and main synaptic scaffolding proteins revealed by super-resolution imaging. Curr Opin Chem Biol. 2014;20:120-126. doi: 10.1016/j.cbpa.2014.05.017
- Compans B, Camus C, Kallergi E, et al. NMDAR-dependent long-term depression is associated with increased short term plasticity through autophagy mediated loss of PSD-95. Nat Commun. 2021;12(1):2849.
- Schnell E, Sizemore M, Karimzadegan S, Chen L, Bredt DS, Nicoll RA. Direct interactions between PSD-95 and stargazin control synaptic AMPA receptor number. Proc Natl Acad Sci U S A. 2002;99(21):13902-13907. doi: 10.1073/pnas.172511199
- El-Husseini AE, Schnell E, Chetkovich DM, Nicoll RA, Bredt DS. PSD-95 involvement in maturation of excitatory synapses. Science. 2000;290(5495):1364-1368.
- Rodríguez-Palmero A, Boerrigter MM, Gómez-Andrés D, et al. DLG4-related synaptopathy: A new rare brain disorder. Genet Med. 2021;23(5):888-899. doi: 10.1038/s41436-020-01075-9
- Levy AM, Gomez-Puertas P, Tümer Z. Neurodevelopmental disorders associated with PSD-95 and its interaction partners. Int J Mol Sci. 2022;23(8):4390. doi: 10.3390/ijms23084390
- Yang M, Rubin A, Wondimu R, Grebe T, Ritfeld G. Significant improvement of psychotic symptoms in treatment-resistant schizophrenia with clozapine in an adolescent with SHINE syndrome: A case report. BMC Psychiatry. 2023;23(1):483. doi: 10.1186/s12888-023-04962-y
- Tümer Z, Dye TJ, Prada C, White-Brown AM, MacKenzie A, Levy AM. DLG4-related synaptopathy. In: GeneReviews®. Seattle, WA: University of Washington,Seattle; 2023.
- Vallejo D, Codocedo JF, Inestrosa NC. Posttranslational modifications regulate the postsynaptic localization of PSD-95. Mol Neurobiol. 2017;54:1759-1776. doi: 10.1007/s12035-016-9745-1
- Xu W. PSD-95-like membrane associated guanylate kinases (PSD-MAGUKs) and synaptic plasticity. Curr Opin Neurobiol. 2011;21(2):306-312. doi: 10.1016/j.conb.2011.03.001
- Swann JW, Le JT, Lee CL. Recurrent seizures and the molecular maturation of hippocampal and neocortical glutamatergic synapses. Dev Neurosci. 2006;29(1-2):168-178. doi: 10.1159/000096221
- Catts VS, Derminio DS, Hahn CG, Weickert CS. Postsynaptic density levels of the NMDA receptor NR1 subunit and PSD-95 protein in prefrontal cortex from people with schizophrenia. NPJ Schizophr. 2015;1(1):15037. doi: 10.1038/npjschz.2015.37
- De Rubeis S, He X, Goldberg AP, et al. Synaptic, transcriptional and chromatin genes disrupted in autism. Nature. 2014;515(7526):209-215. doi: 10.1038/nature13772
- Feyder M, Karlsson RM, Mathur P, et al. Association of mouse Dlg4 (PSD-95) gene deletion and human DLG4 gene variation with phenotypes relevant to autism spectrum disorders and Williams’ syndrome. Am J Psychiatry. 2010;167(12):1508-1517. doi: 10.1176/appi.ajp.2010.10040484
- Lelieveld SH, Reijnders MRF, Pfundt R, et al. Meta-analysis of 2,104 trios provides support for 10 new genes for intellectual disability. Nat Neurosci. 2016;19(9):1194-1196. doi: 10.1038/nn.4352
- Shao CY, Mirra SS, Sait HB, Sacktor TC, Sigurdsson EM. Postsynaptic degeneration as revealed by PSD-95 reduction occurs after advanced Aβ and tau pathology in transgenic mouse models of Alzheimer’s disease. Act Neuropathol. 2011;122:285-292. doi: 10.1007/s00401-011-0843-x
- Dore K, Carrico Z, Alfonso S, et al. PSD-95 protects synapses from β-amyloid. Cell Rep. 2021;35(9):109194. doi: 10.1016/j.celrep.2021.109194
- Glantz LA, Gilmore JH, Hamer RM, Lieberman JA, Jarskog LF. Synaptophysin and postsynaptic density protein 95 in the human prefrontal cortex from mid-gestation into early adulthood. Neuroscience. 2007;149(3):582-591. doi: 10.1016/j.neuroscience.2007.06.036
- Crespo-Facorro B, Barbadillo L, Pelayo-Terán JM, Rodríguez-Sánchez JM. Neuropsychological functioning and brain structure in schizophrenia. Int Rev Psychiatry. 2007;19(4):325-336. doi: 10.1080/09540260701486647
- Kolluri N, Sun Z, Sampson AR, Lewis DA. Lamina-specific reductions in dendritic spine density in the prefrontal cortex of subjects with schizophrenia. Am J Psychiatry. 2005;162(6):1200-1202. doi: 10.1176/appi.ajp.162.6.1200
- Ohnuma T, Kato H, Arai H, Faull RLM, McKenna PJ, Emson PC. Gene expression of PSD95 in prefrontal cortex and hippocampus in schizophrenia. Neuroreport. 2000;11(14):3133-3137. doi: 10.1097/00001756-200009280-00019
- Dracheva S, Marras SA, Elhakem SL, Kramer FR, Davis KL, Haroutunian V. N-methyl-D-aspartic acid receptor expression in the dorsolateral prefrontal cortex of elderly patients with schizophrenia. Am J Psychiatry. 2001;158(9):1400-1410. doi: 10.1176/appi.ajp.158.9.1400
- Toro C, Deakin JFW. NMDA receptor subunit NRI and postsynaptic protein PSD-95 in hippocampus and orbitofrontal cortex in schizophrenia and mood disorder. Schizophr Res. 2005;80(2):323-330. doi: 10.1016/j.schres.2005.07.003
- Hanse E, Seth H, Riebe I. AMPA-silent synapses in brain development and pathology. Nat Rev Neurosci. 2013;14(12):839-850. doi: 10.1038/nrn3642
- Huang X, Stodieck SK, Goetze B, et al. Progressive maturation of silent synapses governs the duration of a critical period. Proc Natl Acad Sci U S A. 2015;112(24):E3131-E3140. doi: 10.1073/pnas.1506488112
- Béïque JC, Lin DT, Kang MG, Aizawa H, Takamiya K, Huganir RL. Synapse-specific regulation of AMPA receptor function by PSD-95. Proc Natl Acad Sci U S A. 2006;103(51):19535-19540. doi: 10.1073/pnas.0608492103
- de Bartolomeis A, Barone A, Vellucci L, et al. Linking inflammation, aberrant glutamate-dopamine interaction, and post-synaptic changes: Translational relevance for schizophrenia and antipsychotic treatment: A systematic review. Mol Neurobiol. 2022;59(10):6460-6501. doi: 10.1007/s12035-022-02976-3
- Clinton SM, Haroutunian V, Davis KL, Meador-Woodruff JH. Altered transcript expression of NMDA receptor-associated postsynaptic proteins in the thalamus of subjects with schizophrenia. Am J Psychiatry. 2003;160(6):1100-1109. doi: 10.1176/appi.ajp.160.6.1100
- Kristiansen LV, Beneyto M, Haroutunian V, Meador- Woodruff JH. Changes in NMDA receptor subunits and interacting PSD proteins in dorsolateral prefrontal and anterior cingulate cortex indicate abnormal regional expression in schizophrenia. Mol Psychiatry. 2006;11(8):737-747. doi: 10.1038/sj.mp.4001844
- Xiong GJ, Cheng XT, Sun T, et al. Defects in syntabulin-mediated synaptic cargo transport associate with autism-like synaptic dysfunction and social behavioral traits. Mol Psychiatry. 2021;26(5):1472-1490. doi: 10.1038/s41380-020-0713-9
- Bhandari R, Paliwal JK, Kuhad A. Neuropsychopathology of autism spectrum disorder: Complex interplay of genetic, epigenetic, and environmental factors. Adv Neurobiol. 2020;24:97-141. doi: 10.1007/978-3-030-30402-7_4
- Kim KC, Kim P, Go HS, et al. Male‐specific alteration in excitatory post‐synaptic development and social interaction in pre‐natal valproic acid exposure model of autism spectrum disorder. J Neurochem. 2013;124(6):832-843. doi: 10.1111/jnc.12147
- Tsai NP, Wilkerson JR, Guo W, et al. Multiple autism-linked genes mediate synapse elimination via proteasomal degradation of a synaptic scaffold PSD-95. Cell. 2012;151(7):1581-1594. doi: 10.1016/j.cell.2012.11.040
- Fujita-Jimbo E, Tanabe Y, Yu Z, et al. The association of GPR85 with PSD-95-neuroligin complex and autism spectrum disorder: A molecular analysis. Mol Autism. 2015;6:17. doi: 10.1186/s13229-015-0012-5
- Xing J, Kimura H, Wang C, et al. Resequencing and association analysis of six PSD-95-related genes as possible susceptibility genes for schizophrenia and autism spectrum disorders. Sci Rep. 2016;6(1):27491. doi: 10.1038/srep27491
- Prange O, Wong TP, Gerrow K, Wang YT, El-Husseini A. A balance between excitatory and inhibitory synapses is controlled by PSD-95 and neuroligin. Proc Natl Acad Sci U S A. 2004;101(38):13915-13920. doi: 10.1073/pnas.0405939101
- Todd PK, Mack KJ, Malter JS. The fragile X mental retardation protein is required for type-I metabotropic glutamate receptor-dependent translation of PSD-95. Proc Natl Acad Sci U S A. 2003;100(24):14374-14378. doi: 10.1073/pnas.2336265100
- de Bartolomeis A, Barone A, Buonaguro EF, Tomasetti C, Vellucci L, Iasevoli F. The Homer1 family of proteins at the crossroad of dopamine-glutamate signaling: An emerging molecular “Lego” in the pathophysiology of psychiatric disorders. A systematic review and translational insight. Neurosci Biobehav Rev. 2022;136:104596. doi: 10.1016/j.neubiorev.2022.104596
- da Silva BS, Grevet EH, Silva LCF, Ramos JKN, Rovaris DL, Bau CHD. An overview on neurobiology and therapeutics of attention-deficit/hyperactivity disorder. Discov Ment Health. 2023;3(1):2.
- del Campo N, Chamberlain SR, Sahakian BJ, Robbins TW. The roles of dopamine and noradrenaline in the pathophysiology and treatment of attention-deficit/hyperactivity disorder. Biol Psychiatry. 2011;69(12):e145-e157. doi: 10.1016/j.biopsych.2011.02.036
- Yao WD, Gainetdinov RR, Arbuckle MI, et al. Identification of PSD-95 as a regulator of dopamine-mediated synaptic and behavioral plasticity. Neuron. 2004;41(4):625-638. doi: 10.1016/s0896-6273(04)00048-0
- Wang LJ, Yu YH, Fu ML, et al. Attention deficit–hyperactivity disorder is associated with allergic symptoms and low levels of hemoglobin and serotonin. Sci Rep. 2018;8(1):10229. doi: 10.1038/s41598-018-28702-5
- Bauer J, Werner A, Kohl W, et al. Hyperactivity and impulsivity in adult attention-deficit/hyperactivity disorder is related to glutamatergic dysfunction in the anterior cingulate cortex. World J Biol Psychiatry. 2018;19(7):538-546. doi: 10.1080/15622975.2016.1262060
- Bollmann S, Ghisleni C, Poil SS, et al. Developmental changes in gamma-aminobutyric acid levels in attention-deficit/ hyperactivity disorder. Transl Psychiatry. 2015;5(6):e589. doi: 10.1038/tp.2015.79
- Cheng J, Liu A, Shi MY, Yan Z. Disrupted glutamatergic transmission in prefrontal cortex contributes to behavioral abnormality in an animal model of ADHD. Neuropsychopharmacol. 2017;42(10):2096-2104. doi: 10.1038/npp.2017.30
- Kotecha SA, Oak JN, Jackson MF, et al. A D2 class dopamine receptor transactivates a receptor tyrosine kinase to inhibit NMDA receptor transmission. Neuron. 2002;35(6):1111-1122. doi: 10.1016/s0896-6273(02)00859-0
- Yuen EY, Zhong P, Yan Z. Homeostatic regulation of glutamatergic transmission by dopamine D4 receptors. Proc Natl Acad Sci U S A. 2010;107(51):22308-22313. doi: 10.1073/pnas.1010025108
- Swann JW, Le JT, Lam TT, Owens J, Mayer AT. The impact of chronic network hyperexcitability on developing glutamatergic synapses. Eur Neurosci. 2007;26(4):975-991. doi: 10.1111/j.1460-9568.2007.05739.x
- Critchlow HM, Maycox PR, Skepper JN, Krylova O. Clozapine and haloperidol differentially regulate dendritic spine formation and synaptogenesis in rat hippocampal neurons. Mol Cell Neurosci. 2006;32(4):356-365. doi: 10.1016/j.mcn.2006.05.007
- Park SW, Lee CH, Cho HY, et al. Effects of antipsychotic drugs on the expression of synaptic proteins and dendritic outgrowth in hippocampal neuronal cultures. Synapse. 2013;67(5):224-234. doi: 10.1002/syn.21634
- Takaki M, Kodama M, Mizuki Y, et al. Effects of the antipsychotics haloperidol, clozapine, and aripiprazole on the dendritic spine. Eur Neuropsychopharmacol. 2018;28(5):610-619. doi: 10.1016/j.euroneuro.2018.03.004
- Leucht S, Cipriani A, Spineli L, et al. Comparative efficacy and tolerability of 15 antipsychotic drugs in schizophrenia: A multiple-treatments meta-analysis. Lancet. 2013;382(9896):951-962. doi: 10.1016/S0140-6736(13)60733-3
- Urs NM, Peterson SM, Caron MG. New concepts in dopamine D2 receptor biased signaling and implications for schizophrenia therapy. Biol Psychiatry. 2017;81(1):78-85. doi: 10.1016/j.biopsych.2016.10.011
- Singh KK, De Rienzo G, Drane L, et al. Common DISC1 polymorphisms disrupt Wnt/GSK3β signaling and brain development. Neuron. 2011;72(4):545-558. doi: 10.1016/j.neuron.2011.09.030
- Mao Y, Ge X, Frank CL, et al. Disrupted in schizophrenia 1 regulates neuronal progenitor proliferation via modulation of GSK3β/β-catenin signaling. Cell. 2009;136(6):1017-1031. doi: 10.1016/j.cell.2008.12.044
- Wang CX, Song JH, Song DK, Yong VW, Shuaib A, Hao C. Cyclin-dependent kinase-5 prevents neuronal apoptosis through ERK-mediated upregulation of Bcl-2. Cell Death Differ. 2006;13(7):1203-1212. doi: 10.1038/sj.cdd.4401804
- de Bartolomeis A, Vellucci L, De Simone G, Mazza B, Barone A, Ciccarelli M. Dysregulated signaling at postsynaptic density: A systematic review and translational appraisal for the pathophysiology, clinics, and antipsychotics’ treatment of schizophrenia. Cells. 2023;12(4):574. doi: 10.3390/cells12040574
- Iasevoli F, Ambesi-Impiombato A, Fiore G, Panariello F, Muscettola G, de Bartolomeis A. Pattern of acute induction of Homer1a gene is preserved after chronic treatment with first-and second-generation antipsychotics: Effect of short-term drug discontinuation and comparison with Homer1a-interacting genes. J Psychopharmacol. 2011;25(7):875-887. doi: 10.1177/0269881109358199
- Pithadia AB, Jain SM. 5-Hydroxytryptamine receptor subtypes and their modulators with therapeutic potentials. J Clin Med Res. 2009;1(2):72-80. doi: 10.4021/jocmr2009.05.1237
- Rapport MM, Green AA, Page IH. Serum vasoconstrictor (serotonin): IV. Isolation and characterization. J Bio Chem. 1948;176(3):1243-1251.
- Harrington RA, Lee LC, Crum RM, Zimmerman AW, Hertz‐ Picciotto I. Serotonin hypothesis of autism: Implications for selective serotonin reuptake inhibitor use during pregnancy. Autism Res. 2013;6(3):149-168. doi: 10.1002/aur.1288
- Lacivita E, Perrone R, Margari L, Leopoldo M. Targets for drug therapy for autism spectrum disorder: Challenges and future directions. J Med Chem. 2017;60(22):9114-9141. doi: 10.1021/acs.jmedchem.7b00965
- Shi J, Landry M, Carrasco GA, Battaglia G, Muma NA. Sustained treatment with a 5-HT2A receptor agonist causes functional desensitization and reductions in agonist-labeled 5-HT2A receptors despite increases in receptor protein levels in rats. Neuropharmacology. 2008;55(5):687-692. doi: 10.1016/j.neuropharm.2008.06.001
- de la Fuente Revenga M, Jaster AM, McGinn J, Silva G, Saha S, González-Maeso J. Tolerance and cross-tolerance among psychedelic and nonpsychedelic 5-HT2A receptor agonists in mice. ACS Chem Neurosci. 2022;13(16):2436-2448. doi: 10.1021/acschemneuro.2c00170
- Gray JA, Roth BL. Paradoxical trafficking and regulation of 5-HT2A receptors by agonists and antagonists. Brain Res. 2001;56(5):441-451. doi: 10.1016/s0361-9230(01)00623-2
- Abbas AI, Yadav PN, Yao WD, et al. PSD-95 is essential for hallucinogen and atypical antipsychotic drug actions at serotonin receptors. J Neurosci. 2009;29(22):7124-7136. doi: 10.1523/JNEUROSCI.1090-09.2009
- Celada P, Puig MV, Amargós-Bosch M, Adell A, Artigas F. The therapeutic role of 5-HT1A and 5-HT2A receptors in depression. J Psychiatry Neurosci. 2004;29(4):252-265.
- Yamauchi M, Miyara T, Matsushima T, Imanishi T. Desensitization of 5-HT2A receptor function by chronic administration of selective serotonin reuptake inhibitors. Brain Res. 2006;1067(1):164-169. doi: 10.1016/j.brainres.2005.10.075
- Sawyer EK, Mun J, Nye JA, et al. Neurobiological changes mediating the effects of chronic fluoxetine on cocaine use. Neuropsychopharmacology. 2012;37(8):1816-1824. doi: 10.1038/npp.2012.29
- Quesseveur G, Reperant C, David D, Gardier A, Sanchez C, Guiard B. 5-HT 2A receptor inactivation potentiates the acute antidepressant-like activity of escitalopram: Involvement of the noradrenergic system. Exp Brain Res. 2013;226:285-295. doi: 10.1007/s00221-013-3434-3
- Siepmann T, Kepplinger J, Zerna C, et al. The effects of pretreatment versus de novo treatment with selective serotonin reuptake inhibitors on short-term outcome after acute ischemic stroke. J Stroke Cerebrovasc Dis. 2015;24(8):1886-1892. doi: 10.1016/j.jstrokecerebrovasdis.2015.04.033
- Aguiar RP, Soares LM, Meyer E, et al. Activation of 5-HT1A postsynaptic receptors by NLX-101 results in functional recovery and an increase in neuroplasticity in mice with brain ischemia. Prog Neuropsychopharmacol Biol Psychiatry. 2020;99:109832. doi: 10.1016/j.pnpbp.2019.109832
- Blanpied TA, Kerr JM, Ehlers MD. Structural plasticity with preserved topology in the postsynaptic protein network. Proc Natl Acad Sci U S A. 2008;105(34):12587-12592. doi: 10.1073/pnas.0711669105
- Bastianetto S, Danik M, Mennicken F, Williams S, Quirion R. Prototypical antipsychotic drugs protect hippocampal neuronal cultures against cell death induced by growth medium deprivation. BMC Neurosci. 2006;7:28. doi: 10.1186/1471-2202-7-28
- Seo MK, Hien LT, Park MK, et al. AMPA receptor-mTORC1 signaling activation is required for neuroplastic effects of LY341495 in rat hippocampal neurons. Sci Rep. 2020;10(1):993. doi: 10.1038/s41598-020-58017-3
- Kobayashi M, Nagata S, Kita Y, et al. Expression of a constitutively active phosphatidylinositol 3-kinase induces process formation in rat PC12 cells: Use of Cre/loxP recombination system. J Biol Chem. 1997;272(26):16089-16092. doi: 10.1074/jbc.272.26.16089
- Reinés A, Cereseto M, Ferrero A, Sifonios L, Podestá MF, Wikinski S. Maintenance treatment with fluoxetine is necessary to sustain normal levels of synaptic markers in an experimental model of depression: Correlation with behavioral response. Neuropsychopharmacology. 2008;33(8):1896-1908. doi: 10.1038/sj.npp.1301596
- O’Leary OF, Wu X, Castren E. Chronic fluoxetine treatment increases expression of synaptic proteins in the hippocampus of the ovariectomized rat: Role of BDNF signalling. Psychoneuroendocrinology. 2009;34(3):367-381. doi: 10.1016/j.psyneuen.2008.09.015
- Liu YM, Hu CY, Shen JD, Wu SH, Li YC, Yi LT. Elevation of synaptic protein is associated with the antidepressant-like effects of ferulic acid in a chronic model of depression. Physiol Behav. 2017;169:184-188. doi: 10.1016/j.physbeh.2016.12.003
- Li Q, Gao Y, Li H, et al. Brain structure and synaptic related protein expression alterations after antidepressant treatment in WKY depression model rats. J Affect Disord. 2022;314:293-302. doi: 10.1016/j.jad.2022.07.037
- Nguyen V, Tiemann D, Park E, Salehi A. Alpha-2 agonists. Anesth Clin. 2017;35(2):233-245. doi: 10.1016/j.anclin.2017.01.009
- Giovannitti JA Jr., Thoms SM, Crawford JJ. Alpha-2 adrenergic receptor agonists: A review of current clinical applications. Anesth Prog. 2015;62(1):31-39. doi: 10.2344/0003-3006-62.1.31
- Munawar N, Al Madhoun A, Nader J, et al. 24-LB: Guanfacine normalizes Presynaptic alpha-2 adrenoceptors enrichment and ameliorates neuropathic pain in type 1 diabetic rats. Diabetes. 2022;71(Suppl 1):2146. doi: 10.3390/pharmaceutics14102146
- Wang M, Ramos BP, Paspalas CD, et al. α2A-adrenoceptors strengthen working memory networks by inhibiting cAMP-HCN channel signaling in prefrontal cortex. Cell. 2007;129(2):397-410. doi: 10.1016/j.cell.2007.03.015
- Smith RJ, Aston-Jones G. α2 Adrenergic and imidazoline receptor agonists prevent cue-induced cocaine seeking. Biol Psychiatry. 2011;70(8):712-719. doi: 10.1016/j.biopsych.2011.06.010
- Sabetkasaie M, Vala S, Khansefid N, Hosseini AR, Sadat Ladgevardi MAR. Clonidine and guanfacine-induced antinociception in visceral pain: Possible role of α2/I2 binding sites. Eur Pharmacol. 2004;501(1):95-101. doi: 10.1016/j.ejphar.2004.08.010
- Birnbaum SG, Podell DM, Arnsten AFT. Noradrenergic alpha-2 receptor agonists reverse working memory deficits induced by the anxiogenic drug, FG7142, in rats. Pharmacol Biochem Behav. 2000;67(3):397-403. doi: 10.1016/s0091-3057(00)00306-3
- Hains AB, Yabe Y, Arnsten AF. Chronic stimulation of alpha-2A-adrenoceptors with guanfacine protects rodent prefrontal cortex dendritic spines and cognition from the effects of chronic stress. Neurobiol Stress. 2015;2:1-9. doi: 10.1016/j.ynstr.2015.01.001
- Arnsten AFT, Raskind MA, Taylor FB, Connor DF. The effects of stress exposure on prefrontal cortex: Translating basic research into successful treatments for post-traumatic stress disorder. Neurobiol Stress. 2015;1:89-99. doi: 10.1016/j.ynstr.2014.10.002
- Johnson JW, Kotermanski SE. Mechanism of action of memantine. Curr Pharmacol. 2006;6(1):61-67. doi: 10.1016/j.coph.2005.09.007
- Krivoy A, Weizman A, Laor L, Hellinger N, Zemishlany Z, Fischel T. Addition of memantine to antipsychotic treatment in schizophrenia inpatients with residual symptoms: A preliminary study. Eur Neuropsychopharmacol. 2008;18(2):117-121. doi: 10.1016/j.euroneuro.2007.07.008
- Olney JW, Farber NB. Glutamate receptor dysfunction and schizophrenia. Arch Gen Psychiatry. 1995;52(12):998-1007. doi: 10.1001/archpsyc.1995.03950240016004
- Carpenter SS, Hatchett AD, Fuller MA. Catatonic schizophrenia and the use of memantine. Ann Pharmacother. 2006;40(2):344-346. doi: 10.1345/aph.1G297
- Prickaerts J, Gieling ET, Bruder AK, van der Staay FJ, Vanmierlo T. Long‐term effects of prenatal allopurinol treatment on brain plasticity markers in low and normal birth weight piglets. Int J Dev Neurosci. 2014;33(1):29-32. doi: 10.1016/j.ijdevneu.2013.11.001
- Herken H, Gurel A, Selek S, et al. Adenosine deaminase, nitric oxide, superoxide dismutase, and xanthine oxidase in patients with major depression: Impact of antidepressant treatment. Arch Med Res. 2007;38(2):247-252. doi: 10.1016/j.arcmed.2006.10.005
- Gibney SM, Fagan EM, Waldron AM, O’Byrne J, Connor TJ, Harkin A. Inhibition of stress-induced hepatic tryptophan 2, 3-dioxygenase exhibits antidepressant activity in an animal model of depressive behaviour. Int J Neuropsychopharmacol. 2014;17(6):917-928. doi: 10.1017/S1461145713001673
- Réus GZ, Jansen K, Titus S, Carvalho AF, Gabbay V, Quevedo J. Kynurenine pathway dysfunction in the pathophysiology and treatment of depression: Evidences from animal and human studies. J Psychiatr Res. 2015;68:316-328. doi: 10.1016/j.jpsychires.2015.05.007
- Yoshii A, Constantine-Paton M. BDNF induces transport of PSD-95 to dendrites through PI3K-AKT signaling after NMDA receptor activation. Nat Neurosci. 2007;10(6):702-711. doi: 10.1038/nn1903
- Fond G, Loundou A, Rabu C, et al. Ketamine administration in depressive disorders: A systematic review and meta-analysis. Psychopharmacology (Berl). 2014;231:3663-3676. doi: 10.1007/s00213-014-3664-5
- Sleigh J, Harvey M, Voss L, Denny B. Ketamine-more mechanisms of action than just NMDA blockade. Trends Anaesth Crit. 2014;4(2-3):76-81.
- Lisek M, Ferenc B, Studzian M, et al. Glutamate deregulation in ketamine-induced psychosis-a potential role of PSD95, NMDA receptor and PMCA interaction. Front Cell Neurosci. 2017;11:181. doi: 10.3389/fncel.2017.00181
- Bard L, Groc L. Glutamate receptor dynamics and protein interaction: Lessons from the NMDA receptor. Mol Cell Neurosci. 2011;48(4):298-307. doi: 10.1016/j.mcn.2011.05.009
- Shih CT, Sporns O, Yuan SL, et al. Connectomics-based analysis of information flow in the Drosophila Curr Biol. 2015;25(10):1249-1258.