Targeted protein degraders: Blood–brain barrier permeability and central nervous system exposure
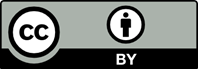
Innovative approaches are essential for treating central nervous system (CNS) diseases that present severe neurological manifestations and low survival rates. Delivering chemical or biological molecules across the blood–brain barrier (BBB) at therapeutically effective concentrations to treat CNS pathologies is a significant challenge. The urgent need for novel treatments targeting disease-causing proteins has propelled targeted protein degraders (TPDs) into the spotlight. TPDs have emerged as promising therapeutics for the treatment of CNS proteinopathies, characterized by the accumulation of misfolded protein aggregates. Given their structural features, the BBB permeability and CNS bioavailability of TPDs may seem improbable. However, several TPDs have demonstrated measurable concentrations in cerebrospinal fluid and the brain. Understanding the mechanisms behind their permeability across the BBB could open new avenues for the development of more effective TPD-based therapies for CNS proteinopathies. This review explores the absorption, distribution, metabolism, and excretion properties of TPDs in relation to brain pharmacokinetic parameters. It also delves into the likely interactions of advanced-stage TPDs with drug transporters and possibilities of disruption-propelled versus B-B barrier permeability-driven CNS bioavailability. Finally, it provides critical insights into the BBB permeability aspects of TPDs, uncovering new dimensions for future research.

- Pajouhesh H, Lenz GR. Medicinal chemical properties of successful central nervous system drugs. NeuroRx. 2005;2(4):541-553. doi: 10.1602/neurorx.2.4.541
- Fong CW. Permeability of the blood brain barrier: Molecular mechanism of transport of drugs and physiologically important compounds. J Membr Biol. 2015;248(4):651-669. doi: 10.1007/s00232-015-9778-9
- Pardridge WM. The blood-brain barrier: Bottleneck in brain drug development. NeuroRx. 2005;2(1):3-14. doi: 10.1602/neurorx.2.1.3
- Liu X, Kalogeropulou AF, Domingos S, et al. Discovery of XL01126: A potent, fast, cooperative, selective, oral bioavailable and blood brain barrier penetrant PROTAC degrader of leucine rich repeat kinase 2. J Am Chem Soc. 2022;144(37):16930-16952. doi: 10.1021/jacs.2c05499
- Catarina Silva M, Ferguson FM, Cai Q, et al. Targeted degradation of aberrant tau in frontotemporal dementia patient-derived neuronal cell models. Elife. 2019;8:e45457. doi: 10.7554/eLife.45457
- Wang W, Zhou Q, Jiang T, et al. A novel small-molecule PROTAC selectively promotes tau clearance to improve cognitive functions in Alzheimer-like models. Theranostics. 2021;11(11):5279-5295. doi: 10.7150/thno.55680
- Risser L, Plouraboué F, Cloetens P, Fonta C. A 3D-investigation shows that angiogenesis in primate cerebral cortex mainly occurs at capillary level. Int J Dev Neurosci. 2009;27(2):185-196. doi: 10.1016/j.ijdevneu.2008.10.006
- Risser L, Plouraboué F, Steyer A, Cloetens P, Duc CL, Fonta C. From homogeneous to fractal normal and tumorous microvascular networks in the brain. J Cereb Blood Flow Metab. 2007;27(2):293-303. doi: 10.1038/sj.jcbfm.9600332
- Heinzer S, Kuhn G, Krucker T, et al. Novel three-dimensional analysis tool for vascular trees indicates complete micro-networks, not single capillaries, as the angiogenic endpoint in mice overexpressing human VEGF (165) in the brain. Neuroimage. 2008;39(4):1549-1558. doi: 10.1016/j.neuroimage.2007.10.054
- Meyer EP, Ulmann-Schuler A, Staufenbiel M, Krucker T. Altered morphology and 3D architecture of brain vasculature in a mouse model for Alzheimer’s disease. Proc Natl Acad Sci USA. 2008;105(9):3587-3592. doi: 10.1073/pnas.0709788105
- Wong AD, Ye M, Levy AF, Rothstein JD, Bergles DE, Searson PC. The blood-brain barrier: An engineering perspective. Front Neuroeng. 2013;6:7. doi: 10.3389/fneng.2013.00007
- Joan Abbott N, Patabendige AAK, Dolman DEM, Yusof SR, Begley DJ. Structure and function of the blood-brain barrier. Neurobiol Dis. 2010;37(1):13-25. doi: 10.1016/j.nbd.2009.07.030
- DeStefano JG, Jamieson JJ, Linville RM, Searson PC. Benchmarking in vitro tissue-engineered blood-brain barrier models. Fluids Barriers CNS. 2018;15(1):32. doi: 10.1186/s12987-018-0117-2
- Palade GE, Simionescu M, Simionescu N. Structural aspects of the permeability of the microvascular endothelium. Acta Physiol Scand Suppl. 1979;463:11-32.
- Nacer A, Movila A, Baer K, Mikolajczak SA, Kappe SHI, Frevert U. Neuroimmunological blood brain barrier opening in experimental cerebral malaria. PLoS Pathog. 2012;8(10):e1002982. doi: 10.1371/journal.ppat.1002982
- Kienast Y, von Baumgarten L, Fuhrmann M, et al. Real-time imaging reveals the single steps of brain metastasis formation. Nat Med. 2010;16(1):116-122. doi: 10.1038/nm.2072
- Larochelle C, Alvarez JI, Prat A. How do immune cells overcome the blood-brain barrier in multiple sclerosis? FEBS Lett. 2011;585(23):3770-3780. doi: 10.1016/j.febslet.2011.04.066
- Holman DW, Klein RS, Ransohoff RM. The blood-brain barrier, chemokines and multiple sclerosis. Biochim Biophys Acta. 2011;1812(2):220-230. doi: 10.1016/j.bbadis.2010.07.019
- Takeshita Y, Ransohoff RM. Inflammatory cell trafficking across the blood-brain barrier: Chemokine regulation and in vitro models. Immunol Rev. 2012;248:228-239. doi: 10.1111/j.1600-065X.2012.01127.x
- Owens T, Bechmann I, Engelhardt B. Perivascular spaces and the two steps to neuroinflammation. J Neuropathol Exp Neurol. 2008;67(12):1113-1121. doi: 10.1097/NEN.0b013e31818f9ca8
- Kristensson K, Nygård M, Bertini G, Bentivoglio M. African trypanosome infections of the nervous system: Parasite entry and effects on sleep and synaptic functions. Prog Neurobiol. 2010;91(2):152-171. doi: 10.1016/j.pneurobio.2009.12.001
- Engelhardt B, Vajkoczy P, Weller RO. The movers and shapers in immune privilege of the CNS. Nat Immunol. 2017;18:123-131. doi: 10.1038/ni.3666
- Kucharz K, Kristensen K, Bendix Johnsen K, et al. Post-capillary venules are the key locus for transcytosis-mediated brain delivery of therapeutic nanoparticles. Nat Commun. 2021;12(1):4121. doi: 10.1038/s41467-021-24323-1
- Macdonald JA, Murugesan N, Pachter JS. Endothelial cell heterogeneity of blood-brain barrier gene expression along the cerebral microvasculature. J Neurosci Res. 2010;88(7):1457-1474. doi: 10.1002/jnr.22316
- Mehta A, Desai A, Rudd D, et al. Bio-mimicking brain vasculature to investigate the role of heterogeneous shear stress in regulating barrier integrity. Adv Biol (Weinh). 2022;6(12):e2200152. doi: 10.1002/adbi.202200152
- Partyka PP, Godsey GA, Galie JR, et al. Mechanical stress regulates transport in a compliant 3D model of the blood-brain barrier. Biomaterials. 2017;115:30-39. doi: 10.1016/j.biomaterials.2016.11.012
- Simionescu M, Simionescu N, Palade GE. Segmental differentiations of cell junctions in the vascular endothelium. The microvasculature. J Cell Biol. 1975;67(3):863-885. doi: 10.1083/jcb.67.3.863
- Cecchelli R, Berezowski V, Lundquist S, et al. Modelling of the blood-brain barrier in drug discovery and development. Nat Rev Drug Discov. 2007;6:650-661. doi: 10.1038/nrd2368
- Nag S, Begley David J. Blood-brain barrier, exchange of metabolites and gases. In: Pathology and Genetics Cerebrovascular Diseases. Basel: ISN Neuropath Press; 2005. p. 22-29.
- Joan Abbott N. Dynamics of CNS barriers: Evolution, differentiation, and modulation. Cell Mol Neurobiol. 2005;25(1):5-23. doi: 10.1007/s10571-004-1374-y
- Arvanitis CD, Ferraro GB, Jain RK. The blood-brain barrier and blood-tumor barrier in brain tumours and metastases. Nat Rev Cancer. 2020;20(1):26-41. doi: 10.1038/s41568-019-0205-x
- Jena L, McErlean E, McCarthy H. Delivery across the blood- brain barrier: Nanomedicine for glioblastoma multiforme. Drug Deliv Transl Res. 2020;10(2):304-318. doi: 10.1007/s13346-019-00679-2
- Kealy J, Greene C, Campbell M. Blood-brain barrier regulation in psychiatric disorders. Neurosci Lett. 2020;726:133664. doi: 10.1016/j.neulet.2018.06.033
- Pulgar VM. Transcytosis to cross the blood brain barrier, new advancements and challenges. Front Neurosci. 2019;12:1019. doi: 10.3389/fnins.2018.01019
- Khan AR, Liu M, Khan MW, Zhai G. Progress in brain targeting drug delivery system by nasal route. J Control Release. 2017;268:364-389. doi: 10.1016/j.jconrel.2017.09.001
- Pardridge WM. Drug transport across the blood-brain barrier. J Cereb Blood Flow Metab. 2012;32:1959-1972. doi: 10.1038/jcbfm.2012.126
- Møllgård K, Dziegielewska KM, Holst CB, Habgood MD, Saunders NR. Brain barriers and functional interfaces with sequential appearance of ABC efflux transporters during human development. Sci Rep. 2017;7(1):11603. doi: 10.1038/s41598-017-11596-0
- Sharma G, Sharma AR, Lee SS, Bhattacharya M, Nam JS, Chakraborty C. Advances in nanocarriers enabled brain targeted drug delivery across blood brain barrier. Int J Pharm. 2019;559:360-372. doi: 10.1016/j.ijpharm.2019.01.056
- Durant ST, Zheng L, Wang Y, et al. The brain-penetrant clinical ATM inhibitor AZD1390 radiosensitizes and improves survival of preclinical brain tumor models. Sci Adv. 2018;4(6):eaat1719. doi: 10.1126/sciadv.aat1719
- Urquhart BL, Kim RB. Blood-brain barrier transporters and response to CNS-active drugs. Eur J Clin Pharmacol. 2009;65(11):1063-1070. doi: 10.1007/s00228-009-0714-8
- Gao B, Stieger B, Noé B, Fritschy JM, Meier PJ. Localization of the organic anion transporting polypeptide 2 (OATP2) in capillary endothelium and choroid plexus epithelium of rat brain. J Histochem Cytochem. 1999;47(10):1255-1264. doi: 10.1177/002215549904701005
- Ose A, Kusuhara H, Endo C, et al. Functional characterization of mouse organic anion transporting peptide 1A4 in the uptake and efflux of drugs across the blood-brain barrier. Drug Metab Dispos. 2010;38(1):168-176. doi: 10.1124/dmd.109.029454
- Burckhardt G, Burckhardt BC. In-vitro and in-vivo evidence of the importance of organic anion transporters (OATs) in drug therapy. Handb Exp Pharmacol. 2011;201:29-104. doi: 10.1007/978-3-642-14541-4_2
- Ballabh P, Braun A, Nedergaard M. The blood-brain barrier: An overview: Structure, regulation, and clinical implications. Neurobiol Dis. 2004;16(1):1-13. doi: 10.1016/j.nbd.2003.12.016
- Wilhelm I, Nyúl-Tóth A, Suciu M, Hermenean A, Krizbai IA. Heterogeneity of the blood-brain barrier. Tissue Barriers. 2016;4(1):e1143544. doi: 10.1080/21688370.2016.1143544
- Brown PD, Davies SL, Speake T, Millar ID. Molecular mechanisms of cerebrospinal fluid production. Neuroscience. 2004;129(4):957-970. doi: 10.1016/j.neuroscience.2004.07.003
- Schilde LM, Kösters S, Steinbach S, et al. Protein variability in cerebrospinal fluid and its possible implications for neurological protein biomarker research. PLoS One. 2018;13(11):e0206478. doi: 10.1371/journal.pone.0206478
- Joan Abbott N. Evidence for bulk flow of brain interstitial fluid: Significance for physiology and pathology. Neurochem Int. 2004;45(4):545-552. doi: 10.1016/j.neuint.2003.11.006
- Cserr HF, Cooper DN, Suri PK, Patlak CS. Efflux of radiolabeled polyethylene glycols and albumin from rat brain. Am J Physiol. 1981;240(4):F319-F328. doi: 10.1152/ajprenal.1981.240.4.F319
- Cserr HF, Patlak CS. Secretion and bulk flow of interstitial fluid. In: Physiology and Pharmacology of the Blood-Brain Barrier. New York: Springer; 1992. p. 245-261. doi: 10.1007/978-3-642-76894-1_9
- Dolman D, Drndarski S, Joan Abbott N, Rattray M. Induction of aquaporin 1 but not aquaporin 4 messenger RNA in rat primary brain microvessel endothelial cells in culture. J Neurochem. 2005;93(4):825-833. doi: 10.1111/j.1471-4159.2005.03111.x
- Pardridge WM. CSF, blood-brain barrier, and brain drug delivery. Expert Opin Drug Deliv. 2016;13(7):963-975. doi: 10.1517/17425247.2016.1171315
- Reiber H, Felgenhauer K. Protein transfer at the blood cerebrospinal fluid barrier and the quantitation of the humoral immune response within the central nervous system. Clin Chim Acta. 1987;163(3):319-328. doi: 10.1016/0009-8981(87)90250-6
- Fishman RA, Christy NP. Fate of adrenal cortical steroids following intrathecal injection. Neurology. 1965;15:1-6. doi: 10.1212/wnl.15.1.1
- Aird RB. A study of intrathecal, cerebrospinal fluid-to-brain exchange. Exp Neurol. 1984;86(2):342-358. doi: 10.1016/0014-4886(84)90192-4
- Pardridge WM. Blood-brain barrier and delivery of protein and gene therapeutics to brain. Front Aging Neurosci. 2020;11:373. doi: 10.3389/fnagi.2019.00373
- Joan Abbott N, Rönnbäck L, Hansson E. Astrocyte-endothelial interactions at the blood-brain barrier. Nat Rev Neurosci. 2006;7(1):41-53. doi: 10.1038/nrn1824
- Kandel ER, Koester JD, Mack SH, Siegelbaum SA. Principles of Neural Science. New York: McGraw-Hill; 2000.
- Hediger MA, Romero MA, Peng JB, Rolfs A, Takanaga H, Bruford HA. The ABCs of solute carriers: physiological, pathological and therapeutic implications of human membrane transport proteins. Introduction. Pfluegers Arch. 2004;447(5):465-468. doi: 10.1007/s00424-003-1192-y
- Eden BD, Rice AJ, Lovett TD, et al. Microwave-assisted synthesis and in vitro stability of N-benzylamide non-steroidal anti-inflammatory drug conjugates for CNS delivery. Bioorg Med Chem Lett. 2019;29(12):1487-1491. doi: 10.1016/j.bmcl.2019.04.016
- Wohlrab C, Phillips E, Dachs GU. Vitamin C transporters in cancer: Current understanding and gaps in knowledge. Front Oncol. 2017;7:74. doi: 10.3389/fonc.2017.00074
- Boado RJ, Pardridge WM. The brain-type glucose transporter mRNA is specifically expressed at the blood-brain barrier. Biochem Biophys Res Commun. 1990;166(1):174-179. doi: 10.1016/0006-291x(90)91927-k
- Farrell CL, Yang J, Pardridge WM. GLUT1 glucose transporter is present within apical and basolateral membranes of brain epithelial interfaces and in microvascular endothelia with and without tight junctions. J Histochem Cytochem. 1992;40(2):193-199. doi: 10.1177/40.2.1552163
- Covarrubias-Pinto A, Acuña AI, Beltrán FA, Torres-Díaz L, Castro MA. Old things new view: Ascorbic acid protects the brain in neurodegenerative disorders. Int J Mol Sci. 2015;16(12):28194-28217. doi: 10.3390/ijms161226095
- Vanlandewijck M, He L, Mäe MA, et al. A molecular atlas of cell types and zonation in the brain vasculature. Nature. 2018;554(7693):475-480. doi: 10.1038/nature25739
- Hagenbuch B, Gao B, Meier PJ. Transport of xenobiotics across the blood-brain barrier. News Physiol Sci. 2002;17: 231-234. doi: 10.1152/nips.01402.2002
- Löscher W, Potschka H. Blood-brain barrier active efflux transporters: ATP-binding cassette gene family. NeuroRx. 2005;2(1):86-98. doi: 10.1602/neurorx.2.1.86
- Tsuji A. Small molecular drug transfer across the blood-brain barrier via carrier-mediated transport systems. NeuroRx. 2005;2(1):54-62. doi: 10.1602/neurorx.2.1.54
- Saunders NR, Habgood MD, Møllgård K, Dziegielewska KM. The biological significance of brain barrier mechanisms: Help or hindrance in drug delivery to the central nervous system? F1000Res. 2016;5:F1000 Faculty Rev-313. doi: 10.12688/f1000research.7378.1
- Virgintino D, Robertson D, Errede M, et al. Expression of P-glycoprotein in human cerebral cortex microvessels. J Histochem Cytochem. 2002;50(12):1671-1676. doi: 10.1177/002215540205001212
- Nies AT. The role of membrane transporters in drug delivery to brain tumors. Cancer Lett. 2007;254(1):11-29. doi: 10.1016/j.canlet.2006.12.023
- Higgins CF, Gottesman MM. Is the multidrug transporter a flippase? Trends Biochem Sci. 1992;17(1):18-21. doi: 10.1016/0968-0004(92)90419-a
- Qosa H, Miller DS, Pasinelli P, Trotti D. Regulation of ABC efflux transporters at blood-brain barrier in health and neurological disorders. Brain Res. 2015;1628:298-316. doi: 10.1016/j.brainres.2015.07.005
- Shawahna R, Uchida Y, Declèves X, et al. Transcriptomic and quantitative proteomic analysis of transporters and drug metabolizing enzymes in freshly isolated human brain microvessels. Mol Pharm. 2011;8(4):1332-1341. doi: 10.1021/mp200129p
- Watanabe R, Esaki T, Ohashi R, et al. Development of an in silico prediction model for P-glycoprotein efflux potential in brain capillary endothelial cells toward the prediction of brain penetration. J Med Chem. 2021;64(5):2725-2738. doi: 10.1021/acs.jmedchem.0c02011
- Li Z, Krippendorff BF, Sharma S, Walz AC, Lavé T, Shah DK. Influence of molecular size on tissue distribution of antibody fragments. MAbs. 2016;8(1):113-119. doi: 10.1080/19420862.2015.1111497
- St-Amour I, Paré I, Alata W, et al. Brain bioavailability of human intravenous immunoglobulin and its transport through the murine blood-brain barrier. J Cereb Blood Flow Metab. 2013;33(12):1983-1992. doi: 10.1038/jcbfm.2013.160
- Felgenhauer K. Protein size and cerebrospinal fluid composition. Klin Wochenschr. 1974;52(24):1158-1164. doi: 10.1007/BF01466734
- Poduslo JF, Curran GL, Berg CT. Macromolecular permeability across the blood-nerve and blood-brain barriers. Proc Natl Acad Sci USA. 1994;91(12):5705-5709. doi: 10.1073/pnas.91.12.5705
- Atwal JK, Chen Y, Chiu C, et al. A therapeutic antibody targeting BACE1 inhibits amyloid-β production in vivo. Sci Transl Med. 2011;3(84):84ra43. doi: 10.1126/scitranslmed.3002254
- Schlachetzki F, Zhu C, Pardridge WM. Expression of the neonatal Fc receptor (FcRn) at the blood-brain barrier. J Neurochem. 2002;81(1):203-206. doi: 10.1046/j.1471-4159.2002.00840.x
- Zhang Y, Pardridge WM. Mediated efflux of IgG molecules from brain to blood across the blood-brain barrier. J Neuroimmunol. 2001;114(1-2):168-172. doi: 10.1016/s0165-5728(01)00242-9
- Catarina Silva M, Nandi G, Donovan KA, et al. Discovery and optimization of tau targeted protein degraders enabled by patient induced pluripotent stem cells-derived neuronal models of tauopathy. Front Cell Neurosci. 2022;16:801179. doi: 10.3389/fncel.2022.801179
- George N, Akhtar J, Al Balushi K, Safi SZ, Azmi SNH, Khan SA. The emerging role of proteolysis targeting chimeras (PROTACs) in the treatment of Alzheimer’s disease. Med Chem Res. 2023;32:601-616. doi: 10.1007/s00044-023-03026-w
- Kargbo RB. PROTAC compounds targeting α-synuclein protein for treating neurogenerative disorders: Alzheimer’s and Parkinson’s diseases. ACS Med Chem Lett. 2020;11(6):1086-1087. doi: 10.1021/acsmedchemlett.0c00192
- Jiang X, Zhou J, Wang Y, et al. PROTACs suppression of GSK-3β, a crucial kinase in neurodegenerative diseases. Eur J Med Chem. 2021;210:112949. doi: 10.1016/j.ejmech.2020.112949
- Tseng YL, Lu PC, Lee CC, et al. Degradation of neurodegenerative disease-associated TDP-43 aggregates and oligomers via a proteolysis-targeting chimera. J Biomed Sci. 2023;30(1):27. doi: 10.1186/s12929-023-00921-7
- De Bock M, Van Haver V, Vandenbroucke RE, Decrock E, Wang N, Leybaert L. Into rather unexplored terrain-transcellular transport across the blood-brain barrier. Glia. 2016;64(7):1097-1123. doi: 10.1002/glia.22960
- Wu JR, Hernandez Y, Miyasaki KF, Kwon EJ. Engineered nanomaterials that exploit blood-brain barrier dysfunction for delivery to the brain. Adv Drug Deliv Rev. 2023;197:114820. doi: 10.1016/j.addr.2023.114820
- Gray SM, Aylor KW, Barrett EJ. Unravelling the regulation of insulin transport across the brain endothelial cell. Diabetologia. 2017;60(8):1512-1521. doi: 10.1007/s00125-017-4285-4
- Roberts RL, Fine RE, Sandra A. Receptor-mediated endocytosis of transferrin at the blood-brain barrier. J Cell Sci. 1993;104:521-532. doi: 10.1242/jcs.104.2.521
- Simionescu M, Ghinea N, Fixman A, et al. The cerebral microvasculature of the rat: Structure and luminal surface properties during early development. J Submicrosc Cytol Pathol. 1988;20(2):243-261.
- Stamatovic SM, Sladojevic N, Keep RF, Andjelkovic AV. Blood-brain barrier permeability: From bench to bedside. In: Gunel MK, editor. Management of Epilepsy-Research, Results and Treatment. London: InTech; 2011.
- Hervé F, Ghinea N, Scherrmann JM. CNS delivery via adsorptive transcytosis. AAPS J. 2008;10(3):455-472. doi: 10.1208/s12248-008-9055-2
- Smith MW, Gumbleton M. Endocytosis at the blood-brain barrier: From basic understanding to drug delivery strategies. J Drug Target. 2006;14(4):191-214. doi: 10.1080/10611860600650086
- Pemberton S, Galindo DC, Schwartz MW, Banks WA, Rhea EM. Endocytosis of insulin at the blood-brain barrier. Front Drug Deliv. 2022;2:1062366. doi: 10.3389/fddev.2022.1062366
- Ben-Zvi A, Lacoste B, Kur E, et al. Mfsd2a is critical for the formation and function of the blood-brain barrier. Nature. 2014;509(7501):507-511. doi: 10.1038/nature13324
- Andreone BJ, Chow BW, Tata A, et al. Blood-brain barrier permeability is regulated by lipid transport-dependent suppression of caveolae-mediated transcytosis. Neuron. 2017;94(3):581-594.e5. doi: 10.1016/j.neuron.2017.03.043
- Tashima T. Proteolysis-targeting chimera (PROTAC) delivery into the brain across the blood-brain barrier. Antibodies (Basel). 2023;12(3):43. doi: 10.3390/antib12030043
- Jones AR, Shusta EV. Blood-brain barrier transport of therapeutics via receptor-mediation. Pharm Res. 2007;24(9):1759-1771. doi: 10.1007/s11095-007-9379-0
- Yu YJ, Watts RJ. Developing therapeutic antibodies for neurodegenerative disease. Neurotherapeutics. 2013;10(3):459-472. doi: 10.1007/s13311-013-0187-4
- Fishman JB, Rubin JB, Handrahan JV, Connor JR, Fine RE. Receptor-mediated transcytosis of transferrin across the blood-brain barrier. J Neurosci Res. 1987;18(2):299-304. doi: 10.1002/jnr.490180206
- Lichota J, Skjørringe T, Thomsen LB, Moos T. Macromolecular drug transport into the brain using targeted therapy. J Neurochem. 2010;113(1):1-13. doi: 10.1111/j.1471-4159.2009.06544.x
- van Gelder W, Huijskes-Heins MI, van Dijk JP, Cleton- Soeteman MI, van Eijk HG. Quantification of different transferrin receptor pools in primary cultures of porcine blood-brain barrier endothelial cells. J Neurochem. 1995;64(6):2708-2715. doi: 10.1046/j.1471-4159.1995.64062708.x
- Johnsen KB, Burkhart A, Thomsen LB, Andresen TL, Moos T. Targeting the transferrin receptor for brain drug delivery. Prog Neurobiol. 2019;181:101665. doi: 10.1016/j.pneurobio.2019.101665
- Niewoehner J, Bohrmann B, Collin L, et al. Increased brain penetration and potency of a therapeutic antibody using a monovalent molecular shuttle. Neuron. 2014;81(1):49-60. doi: 10.1016/j.neuron.2013.10.061
- Yu YJ, Zhang Y, Kenrick M, et al. Boosting brain uptake of a therapeutic antibody by reducing its affinity for a transcytosis target. Sci Transl Med. 2011;3(84):84ra44. doi: 10.1126/scitranslmed.3002230
- Ullman JC, Arguello A, Getz JA, et al. Brain delivery and activity of a lysosomal enzyme using a blood-brain barrier transport vehicle in mice. Sci Transl Med. 2020;12(545):eaay1163. doi: 10.1126/scitranslmed.aay1163
- Kariolis MS, Wells RC, Getz JA, et al. Brain delivery of therapeutic proteins using an Fc fragment blood-brain barrier transport vehicle in mice and monkeys. Sci Transl Med. 2020;12(545):eaay1359. doi: 10.1126/scitranslmed.aay1359
- Santisakultarm TP, Cornelius NR, Nishimura N, et al. In-vivo two-photon excited fluorescence microscopy reveals cardiac-and respiration-dependent pulsatile blood flow in cortical blood vessels in mice. Am J Physiol Heart Circ Physiol. 2012;302(7):H1367-H1377. doi: 10.1152/ajpheart.00417.2011
- Helms HCC, Kristensen M, Saaby L, Fricker G, Brodin B. Drug delivery strategies to overcome the blood-brain barrier (BBB). Handb Exp Pharmacol. 2022;273:151-183. doi: 10.1007/164_2020_403
- Sweeney PW, Walker-Samuel S, Shipley RJ. Insights into cerebral haemodynamics and oxygenation utilising in vivo mural cell imaging and mathematical modelling. Sci Rep. 2018;8(1):1373. doi: 10.1038/s41598-017-19086-z
- de Lange ECM. The physiological characteristics and transcytosis mechanisms of the blood-brain barrier (BBB). Curr Pharm Biotechnol. 2012;13(12):2319-2327. doi: 10.2174/138920112803341860
- Bickel U, Yoshikawa T, Pardridge WM. Delivery of peptides and proteins through the blood-brain barrier. Adv Drug Deliv Rev. 2001;46(1-3):247-279. doi: 10.1016/s0169-409x(00)00139-3
- Thöle M, Nobmann S, Huwyler J, Bartmann A, Fricker G. Uptake of cationized albumin coupled liposomes by cultured porcine brain microvessel endothelial cells and intact brain capillaries. J Drug Target. 2002;10(4):337-344. doi: 10.1080/10611860290031840
- Patching SG. Glucose transporters at the blood-brain barrier: Function, regulation and gateways for drug delivery. Mol Neurobiol. 2017;54(2):1046-1077. doi: 10.1007/s12035-015-9672-6.
- Nadal A, Fuentes E, Pastor J, McNaughton PA. Plasma albumin is a potent trigger of calcium signals and DNA synthesis in astrocytes. Proc Natl Acad Sci USA. 1995;92(5):1426-1430. doi: 10.1073/pnas.92.5.1426
- Dvorak AM, Feng D. The vesiculo-vacuolar organelle (VVO). A new endothelial cell permeability organelle. J Histochem Cytochem. 2001;49(4):419-432. doi: 10.1177/002215540104900401
- Komarova Y, Malik AB. Regulation of endothelial permeability via paracellular and transcellular transport pathways. Annu Rev Physiol. 2010;72:463-493. doi: 10.1146/annurev-physiol-021909-135833
- Schnitzer JE, Oh P. Albondin-mediated capillary permeability to albumin. Differential role of receptors in endothelial transcytosis and endocytosis of native and modified albumins. J Biol Chem. 1994;269(8):6072-6082. doi: 10.1016/S0021-9258(17)37571-3
- Tiruppathi C, Song W, Bergenfeldt M, Sass P, Malik AB. Gp60 activation mediates albumin transcytosis in endothelial cells by tyrosine kinase-dependent pathway. J Biol Chem. 1997;272(41):25968-25975. doi: 10.1074/jbc.272.41.25968
- Minshall RD, Sessa WC, Stan RV, Anderson RGW, Malik AB. Caveolin regulation of endothelial function. Am J Physiol Lung Cell Mol Physiol. 2003;285(6):L1179-L1183. doi: 10.1152/ajplung.00242.2003
- Summerfield SG, Yates JWT, Fairman DA. Free drug theory - no longer just a hypothesis? Pharm Res. 2022;39(2):213-222. doi: 10.1007/s11095-022-03172-7
- Volak LP, Duevel HM, Humphreys S, et al. Industry perspective on the pharmacokinetic and absorption, distribution, metabolism, and excretion characterization of heterobifunctional protein degraders. Drug Metab Dispos. 2023;51(7):792-803. doi: 10.1124/dmd.122.001154
- Bohnert T, Gan LS. Plasma protein binding: From discovery to development. J Pharm Sci. 2013;102(9):2953-2994. doi: 10.1002/jps.23614
- Pardridge WM, Sakiyama R, Fierer G. Transport of propranolol and lidocaine through the rat blood-brain barrier. Primary role of globulin-bound drug. J Clin Invest. 1983;71(4):900-908. doi: 10.1172/jci110844
- Videbaek C, Ott P, Paulson OB, Knudsen GM. Blood-brain barrier transport and protein binding of flumazenil and iomazenil in the rat: Implications for neuroreceptor studies. J Cereb Blood Flow Metab. 1999;19(9):948-955. doi: 10.1097/00004647-199909000-00002
- Jolliet P, Simon N, Brée F, et al. Blood-to-brain transfer of various oxicams: Effects of plasma binding on their brain delivery. Pharm Res. 1997;14(5):650-656. doi: 10.1023/a:1012165414610
- Lin TH, Sawada Y, Sugiyama Y, Iga T, Hanano M. Effects of albumin and alpha 1-acid glycoprotein on the transport of imipramine and desipramine through the blood-brain barrier in rats. Chem Pharm Bull (Tokyo). 1987;35(1):294-301. doi: 10.1248/cpb.35.294
- Urien S, Pinquier JL, Paquette B, Chaumet-Riffaud P, Kiechel JR, Tillement JP. Effect of the binding of isradipine and darodipine to different plasma proteins on their transfer through the rat blood-brain barrier. Drug binding to lipoproteins does not limit the transfer of drug. J Pharmacol Exp Ther. 1987;242(1):349-353.
- Ryu S, Tess D, Di L. Addressing the accuracy of plasma protein binding measurement for highly bound compounds using the dilution method. AAPS J. 2022;25(1):7. doi: 10.1208/s12248-022-00774-2
- Isbell J, Yuan D, Torrao L, Gatlik E, Hoffmann L, Wipfli P. Plasma protein binding of highly bound drugs determined with equilibrium gel filtration of nonradiolabeled compounds and LC-MS/MS detection. J Pharm Sci. 2019;108(2):1053-1060. doi: 10.1016/j.xphs.2018.10.004
- Smith QR, Fisher C, Allen DD. The role of plasma protein binding in drug delivery to brain. In: Kobiler D, Lustig S, Shapira S, editors. Blood-Brain Barrier. Boston, MA: Springer; 2001. doi: 10.1007/978-1-4615-0579-2_25
- Pardridge WM, Fierer G. Transport of tryptophan into brain from the circulating, albumin-bound pool in rats and in rabbits. J Neurochem. 1990;54(3):971-976. doi: 10.1111/j.1471-4159.1990.tb02345.x
- Pardridge WM. Recent advances in blood-brain barrier transport. Annu Rev Pharmacol Toxicol. 1988;28:25-39. doi: 10.1146/annurev.pa.28.040188.000325
- de Lange ECM, Danhof M. Considerations in the use of cerebrospinal fluid pharmacokinetics to predict brain target concentrations in the clinical setting: Implications of the barriers between blood and brain. Clin Pharmacokinet. 2002;41(10):691-703. doi: 10.2165/00003088-200241100-00001
- Tonduru AK, Kumar Adla S, Huttunen KM, Kronenberger T, Poso A. Comparative modelling of organic anion transporting polypeptides: Structural insights and comparison of binding modes. Molecules. 2022;27(23):8531. doi: 10.3390/molecules27238531
- Pastan I, Gottesman MM, Ueda K, Lovelace E, Rutherford AV, Willingham MC. A retrovirus carrying an MDR1 cDNA confers multidrug resistance and polarized expression of P-glycoprotein in MDCK cells. Proc Natl Acad Sci U SA. 1988;85(12):4486-4490. doi: 10.1073/pnas.85.12.4486
- Tang F, Horie K, Borchardt RT. Are MDCK cells transfected with the human MDR1 gene a good model of the human intestinal mucosa? Pharm Res. 2002;19(6):765-772. doi: 10.1023/a:1016140429238
- Kuteykin-Teplyakov K, Luna-Tortós C, Ambroziak K, Löscher W. Differences in the expression of endogenous efflux transporters in MDR1-transfected versus wildtype cell lines affect P-glycoprotein mediated drug transport. Br J Pharmacol. 2010;160(6):1453-1463. doi: 10.1111/j.1476-5381.2010.00801.x
- Karyekar CS, Eddington ND, Garimella TS, Gubbins PO, Dowling TC. Evaluation of P-glycoprotein-mediated renal drug interactions in an MDR1-MDCK model. Pharmacotherapy. 2003;23(4):436-442. doi: 10.1592/phco.23.4.436.32125
- Evers R, Zaman GJ, van Deemter L, et al. Basolateral localization and export activity of the human multidrug resistance-associated protein in polarized pig kidney cells. J Clin Invest. 1996;97(5):1211-1218. doi: 10.1172/JCI118535
- Jeliazkova-Mecheva VV, Bobilya DJ. A porcine astrocyte/ endothelial cell co-culture model of the blood-brain barrier. Brain Res Brain Res Protoc. 2003;12:91-98. doi: 10.1016/j.brainresprot.2003.08.004
- Ledwig V, Reichl S. Isolation and cultivation of porcine endothelial cells, pericytes and astrocytes to develop an in vitro blood-brain barrier model for drug permeation testing. Pharmaceutics. 2023;15:1688. doi: 10.3390/pharmaceutics15061688
- Békés M, Langley DR, Crews CM. PROTAC targeted protein degraders: The past is prologue. Nat Rev Drug Discov. 2022;21(3):181-200. doi: 10.1038/s41573-021-00371-6
- Mares A, Miah AH, Smith IED, et al. Extended pharmacodynamic responses observed upon PROTAC-mediated degradation of RIPK2. Commun Biol. 2020;3(1):140. doi: 10.1038/s42003-020-0868-6
- Haid RTU, Reichel A. Transforming the discovery of targeted protein degraders: The translational power of predictive PK/ PD modeling. Clin Pharmacol Ther. 2024;116(3):770-781. doi: 10.1002/cpt.3273
- David L, Wenlock M, Barton P, Ritzén A. Prediction of chameleonic efficiency. ChemMedChem. 2021;16(17): 2669-2685. doi: 10.1002/cmdc.202100306
- Chen W, Jin D, Shi Y, Zhang Y, Zhou H, Li G. The underlying mechanisms of lorlatinib penetration across the blood‐brain barrier and the distribution characteristics of lorlatinib in the brain. Cancer Med. 2020;9(12):4350-4359. doi: 10.1002/cam4.3061
- Bernatz S, Ilina EI, Devraj K, et al. Impact of docetaxel on blood-brain barrier function and formation of breast cancer brain metastases. J Exp Clin Cancer Res. 2019;38(1):434. doi: 10.1186/s13046-019-1427-1
- Lloyd K, Hornykiewicz O. Parkinson’s disease activity of l-dopa decarboxylase in discrete brain regions. Science. 1970;170(3963):1212-1213. doi: 10.1126/science.170.3963.1212
- Fischer H, Gottschlich R, Seelig A. Blood-brain barrier permeation molecular parameters governing passive diffusion. J Membr Biol. 1998;165(3):201-211. doi: 10.1007/s002329900434
- Clark DE. Rapid calculation of polar molecular surface area and its application to the prediction of transport phenomena. 2. Prediction of blood-brain barrier penetration. J Pharm Sci. 1999;88(8):815-821. doi: 10.1021/js980402t
- Kargbo RB. Treatment of Alzheimer’s by PROTAC-Tau protein degradation. ACS Med Chem Lett. 2019;10(5): 699-700. doi: 10.1021/acsmedchemlett.9b00083
- Gosselet F, Loiola RA, Roig A, Rosell A, Culot M. Central nervous system delivery of molecules across the blood-brain barrier. Neurochem Int. 2021;144:104952. doi: 10.1016/j.neuint.2020.104952
- Xiong B, Wang Y, Chen Y, et al. Strategies for structural modification of small molecules to improve blood-brain barrier penetration: A recent perspective. J Med Chem. 2021;64(18):13152-13173. doi: 10.1021/acs.jmedchem.1c00910
- Hanig JP, Morrison JM Jr., Krop S. Ethanol enhancement of blood-brain barrier permeability to catecholamines in chicks. Eur J Pharmacol. 1972;18(1):79-82. doi: 10.1016/0014-2999(72)90134-3
- Broadwell RD, Salcman M, Kaplan RS. Morphologic effect of dimethyl sulfoxide on the blood-brain barrier. Science. 1982;217(4555):164-166. doi: 10.1126/science.7089551
- Azmin MN, Stuart JF, Florence AT. The distribution and elimination of methotrexate in mouse blood and brain after concurrent administration of polysorbate 80. Cancer Chemother Pharmacol. 1985;14(3):238-242. doi: 10.1007/BF00258124
- Sakane T, Tanaka C, Yamamoto A, et al. The effect of polysorbate 80 on brain uptake and analgesic effect of D-kyotorphin. Int J Pharm. 1989;57(1):77-83. doi: 10.1016/0378-5173(89)90266-4
- Chen J, Escoffre JM, Romito O, et al. Enhanced macromolecular substance extravasation through the blood-brain barrier via acoustic bubble-cell interactions. Ultrason Sonochem. 2024;103:106768. doi: 10.1016/j.ultsonch.2024.106768
- Veszelka S, Mészáros M, Porkoláb G, et al. Effects of hydroxypropyl-beta-cyclodextrin on cultured brain endothelial cells. Molecules. 2022;27(22):7738. doi: 10.3390/molecules27227738
- Zünkeler B, Carson RE, Olson J, et al. Quantification and pharmacokinetics of blood-brain barrier disruption in humans. J Neurosurg. 1996;85(6):1056-1065. doi: 10.3171/jns.1996.85.6.1056
- Bynoe MS, Viret C, Yan A, Kim DG. Adenosine receptor signaling: A key to opening the blood-brain door. Fluids Barriers CNS. 2015;12:20. doi: 10.1186/s12987-015-0017-7
- Spieth L, Berghoff SA, Stumpf SK, et al. Anesthesia triggers drug delivery to experimental glioma in mice by hijacking caveolar transport. Neurooncol Adv. 2021;3(1):vdab140. doi: 10.1093/noajnl/vdab140