SARS-CoV-2 persistence: A potential catalyst for age-associated neurodegenerative diseases
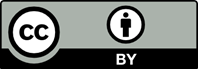
The persistence of RNA viruses in the brain is increasingly recognized as a significant factor in the progression of age-related neurodegenerative diseases. This phenomenon is particularly evident in infections caused by various neurotropic and non-neurotropic viruses, including severe acute respiratory syndrome coronavirus 2 (SARS-CoV-2). The COVID-19 pandemic has underscored the urgent need to explore the complex relationship between viral persistence and neurological decline. Growing evidence indicates that SARS-CoV-2 affects brain structure and function, although the precise molecular mechanisms remain poorly understood. Chronic neuroinflammation induced by viral infections is thought to accelerate age-related neurodegeneration. While the immune system typically clears many viral infections in the brain, some viruses establish chronic infections, leading to restricted viral replication. These persistent infections can exacerbate neuroinflammation and contribute to ongoing neuronal damage, key drivers of age-related neurodegeneration. This review explores current knowledge on how SARS-CoV-2 infiltrates the brain, evades immune defenses, and persists within brain cells, potentially using them as viral reservoirs. As individuals age, the cumulative effects of such viral infections may accelerate cognitive decline and increase vulnerability to neurodegenerative diseases such as Alzheimer’s and Parkinson’s. Understanding the molecular mechanisms of viral persistence and its long-term impact on brain health is crucial for developing targeted therapies to combat these age-related diseases.

- Hiscott J, Alexandridi M, Muscolini M, et al. The global impact of the coronavirus pandemic. Cytokine Growth Factor Rev. 2020;53:1-9. doi: 10.1016/j.cytogfr.2020.05.010
- Li G, Hilgenfeld R, Whitley R, De Clercq E. Therapeutic strategies for COVID-19: Progress and lessons learned. Nat Rev Drug Discov. 2023;22(6):449-475. doi: 10.1038/s41573-023-00672-y
- Wan D, Du T, Hong W, et al. Neurological complications and infection mechanism of SARS-COV-2. Signal Transduct Target Ther. 2021;6(1):406. doi: 10.1038/s41392-021-00818-7
- Nath A, Johnson TP. Mechanisms of viral persistence in the brain and therapeutic approaches. FEBS J. 2022;289(8):2145-2161. doi: 10.1111/febs.15871
- Bedran D, Bedran G, Kote S. A comprehensive review of neurodegenerative manifestations of SARS-CoV-2. Vaccines (Basel). 2024;12(3):222. doi: 10.3390/vaccines12030222
- Javed A, Batra A, Singh M, Sarkar P. Linkage between SARS-CoV-2 infection and neurodegenerative disorders: Review and current update. Adv Neurol. 2024;3(1):2200. doi: 10.36922/an.2200
- Xu E, Xie Y, Al-Aly Z. Long-term neurologic outcomes of COVID-19. Nat Med. 2022;28(11):2406-2415. doi: 10.1038/s41591-022-02001-z
- Zhang W, Xiao D, Mao Q, Xia H. Role of neuroinflammation in neurodegeneration development. Signal Transduct Target Ther. 2023;8(1):267. doi: 10.1038/s41392-023-01486-5
- Saleh J, Peyssonnaux C, Singh KK, Edeas M. Mitochondria and microbiota dysfunction in COVID-19 pathogenesis. Mitochondrion. 2020;54:1-7. doi: 10.1016/j.mito.2020.06.008
- Khomari F, Nabi-Afjadi M, Yarahmadi S, Eskandari H, Bahreini E. Effects of cell proteostasis network on the survival of SARS-CoV-2. Biol Proced Online. 2021;23(1):8. doi: 10.1186/s12575-021-00145-9
- Taeschler P, Cervia C, Zurbuchen Y, et al. Autoantibodies in COVID-19 correlate with antiviral humoral responses and distinct immune signatures. Allergy. 2022;77(8):2415-2430. doi: 10.1111/all.15302
- Chen Z, Li G. Immune response and blood–brain barrier dysfunction during viral neuroinvasion. Innate Immun. 2021;27(2):109-117. doi: 10.1177/1753425920954281
- Krasemann S, Haferkamp U, Pfefferle S, et al. The blood-brain barrier is dysregulated in COVID-19 and serves as a CNS entry route for SARS-CoV-2. Stem Cell Reports. 2022;17(2):307-320. doi: 10.1016/j.stemcr.2021.12.011
- Brown EE, Kumar S, Rajji TK, Pollock BG, Mulsant BH. Anticipating and mitigating the impact of the COVID-19 pandemic on Alzheimer’s disease and related dementias. Am J Geriatr Psychiatry. 2020;28(7):712-721. doi: 10.1016/j.jagp.2020.04.010
- Boura I, Qamar MA, Daddoveri F, et al. SARS-CoV-2 and Parkinson’s disease: A review of where we are now. Biomedicines. 2023;11(9):2524. doi: 10.3390/biomedicines11092524
- Hu B, Guo H, Zhou P, Shi ZL. Characteristics of SARS-CoV-2 and COVID-19. Nat Rev Microbiol. 2021;19(3):141-154. doi: 10.1038/s41579-020-00459-7
- Davis HE, McCorkell L, Vogel JM, Topol EJ. Long COVID: Major findings, mechanisms and recommendations. Nat Rev Microbiol. 2023;21(3):133-146. doi: 10.1038/s41579-022-00846-2
- Li J, Zhou Y, Ma J, et al. The long-term health outcomes, pathophysiological mechanisms and multidisciplinary management of long COVID. Signal Transduct Target Ther. 2023;8(1):416. doi: 10.1038/s41392-023-01640-z
- Blackhurst BM, Funk KE. Viral pathogens increase risk of neurodegenerative disease. Nat Rev Neurol. 2023;19(5):259-260. doi: 10.1038/s41582-023-00790-6
- Raveendran AV, Jayadevan R, Sashidharan S. Long COVID: An overview. Diabetes Metab Syndr. 2021;15(3):869-875. doi: 10.1016/j.dsx.2021.04.007
- Xie Y, Xu E, Bowe B, Al-Aly Z. Long-term cardiovascular outcomes of COVID-19. Nat Med. 2022;28(3):583-590. doi: 10.1038/s41591-022-01689-3
- Xie Y, Al-Aly Z. Risks and burdens of incident diabetes in long COVID: A cohort study. Lancet Diabetes Endocrinol. 2022;10(5):311-321. doi: 10.1016/S2213-8587(22)00044-4
- Hayase Y, Tobita K. Influenza virus and neurological diseases. Psychiatry Clin Neurosci. 1997;51(4):181-184. doi: 10.1111/j.1440-1819.1997.tb02580.x
- Ceban F, Ling S, Lui LMW, et al. Fatigue and cognitive impairment in Post-COVID-19 syndrome: A systematic review and meta-analysis. Brain Behav Immun. 2022;101:93-135. doi: 10.1016/j.bbi.2021.12.020
- Taquet M, Sillett R, Zhu L, et al. Neurological and psychiatric risk trajectories after SARS-CoV-2 infection: An analysis of 2-year retrospective cohort studies including 1 284 437 patients. Lancet Psychiatry. 2022;9(10):815-827. doi: 10.1016/S2215-0366(22)00260-7
- Liu YH, Wu QX, Wang QH, et al. Tracking cognitive trajectories in older survivors of COVID-19 up to 2.5 years post-infection. Nat Aging. 2024;4:1186-1193. doi: 10.1038/s43587-024-00667-3
- Reiken S, Sittenfeld L, Dridi H, Liu Y, Liu X, Marks AR. Alzheimer’s-like signaling in brains of COVID-19 patients. Alzheimers Dement. 2022;18(5):955-965. doi: 10.1002/alz.12558
- Yu B, Chen X, Rich S, Mo Q, Yan H. Dynamics of the coronavirus disease 2019 (COVID-19) epidemic in Wuhan City, Hubei Province and China: A second derivative analysis of the cumulative daily diagnosed cases during the first 85 days. Global Health J. 2021;5(1):4-11. doi: 10.1016/j.glohj.2021.02.001
- Zuo W, He D, Liang C, et al. The persistence of SARS-CoV-2 in tissues and its association with long COVID symptoms: A cross-sectional cohort study in China. Lancet Infect Dis. 2024;24:845-855. doi: 10.1016/S1473-3099(24)00171-3
- Buonsenso D, Tantisira KG. Long COVID and SARS-CoV-2 persistence: New answers, more questions. Lancet Infect Dis. 2024;24:796-798. doi: 10.1016/S1473-3099(24)00216-0
- Ding Q, Zhao HJ. Long-term effects of SARS-CoV-2 infection on human brain and memory. Cell Death Discov. 2023;9(1):196. doi: 10.1038/s41420-023-01512-z
- Guerrero JI, Barragán LA, Martínez JD, et al. Central and peripheral nervous system involvement by COVID-19: A systematic review of the pathophysiology, clinical manifestations, neuropathology, neuroimaging, electrophysiology, and cerebrospinal fluid findings. BMC Infect Dis. 2021;21(1):515. doi: 10.1186/s12879-021-06185-6
- Mao L, Jin H, Wang M, et al. Neurologic manifestations of hospitalized patients with coronavirus disease 2019 in Wuhan, China. JAMA Neurol. 2020;77(6):683-690. doi: 10.1001/jamaneurol.2020.1127
- Almeria M, Cejudo JC, Sotoca J, Deus J, Krupinski J. Cognitive profile following COVID-19 infection: Clinical predictors leading to neuropsychological impairment. Brain Behav Immun Health. 2020;9:100163. doi: 10.1016/j.bbih.2020.100163
- Jacot de Alcântara I, Nuber-Champier A, Voruz P, Cionca A, Assal F, Péron JA. Cognitive deficits in the acute phase of COVID-19: A review and meta-analysis. J Clin Med. 2023;12(3):762. doi: 10.3390/jcm12030762
- Meinhardt J, Radke J, Dittmayer C, et al. Olfactory transmucosal SARS-CoV-2 invasion as a port of central nervous system entry in individuals with COVID-19. Nat Neurosci. 2021;24(2):168-175. doi: 10.1038/s41593-020-00758-5
- Siow I, Lee KS, Zhang JJY, Saffari SE, Ng A, Young B. Stroke as a neurological complication of COVID-19: A systematic review and meta-analysis of incidence, outcomes and predictors: Stroke and COVID-19. J Stroke Cerebrovasc Dis. 2021;30(3):105549. doi:10.1016/j.jstrokecerebrovasdis.2020.105549
- Conway EM, Mackman N, Warren RQ, et al. Understanding COVID-19-associated coagulopathy. Nat Rev Immunol. 2022;22(10):639-649. doi: 10.1038/s41577-022-00762-9
- Qureshi AI, Baskett WI, Huang W, et al. Acute ischemic stroke and COVID-19: An analysis of 27 676 patients. Stroke. 2021;52(3):905-912. doi: 10.1161/STROKEAHA.120.031786
- Douaud G, Lee S, Alfaro-Almagro F, et al. SARS-CoV-2 is associated with changes in brain structure in UK Biobank. Nature. 2022;604(7907):697-707. doi: 10.1038/s41586-022-04569-5
- Manca R, De Marco M, Ince PG, Venneri A. Heterogeneity in regional damage detected by neuroimaging and neuropathological studies in older adults with COVID-19: A cognitive-neuroscience systematic review to inform the long-term impact of the virus on neurocognitive trajectories. Front Aging Neurosci. 2021;13:646908. doi: 10.3389/fnagi.2021.646908
- Hosp JA, Reisert M, Dressing A, et al. Cerebral microstructural alterations in Post-COVID-condition are related to cognitive impairment, olfactory dysfunction and fatigue. Nat Commun. 2024;15(1):4526. doi: 10.1038/s41467-024-48651-0
- Spindler KR, Hsu TH. Viral disruption of the blood-brain barrier. Trends Microbiol. 2012;20(6):282-290. doi: 10.1016/j.tim.2012.03.009
- Ayala-Nunez NV, Gaudin R. A viral journey to the brain: Current considerations and future developments. PLoS Pathog. 2020;16(5):e1008434. doi: 10.1371/journal.ppat.1008434
- Jackson CB, Farzan M, Chen B, Choe H. Mechanisms of SARS-CoV-2 entry into cells. Nat Rev Mol Cell Biol. 2022;23(1):3-20. doi: 10.1038/s41580-021-00418-x
- Erickson MA, Rhea EM, Knopp RC, Banks WA. Interactions of SARS-COV-2 with the blood-brain barrier. Int J Mol Sci. 2021;22(5):2681. doi: 10.3390/ijms22052681
- Lima M, Siokas V, Aloizou AM, et al. Unraveling the possible routes of SARS-COV-2 invasion into the central nervous system. Curr Treat Options Neurol. 2020;22(11):37. doi: 10.1007/s11940-020-00647-z
- Li W, Moore MJ, Vasilieva N, et al. Angiotensin-converting enzyme 2 is a functional receptor for the SARS coronavirus. Nature. 2003;426(6965):450-454. doi: 10.1038/nature02145
- Bourgonje AR, Abdulle AE, Timens W, et al. Angiotensin-converting enzyme 2 (ACE2), SARS-CoV-2 and the pathophysiology of coronavirus disease 2019 (COVID-19). J Pathol. 2020;251(3):228-248. doi: 10.1002/path.5471
- Huang Y, Yang C, Xu XF, Xu W, Liu SW. Structural and functional properties of SARS-CoV-2 spike protein: Potential antivirus drug development for COVID-19. Acta Pharmacol Sin. 2020;41(9):1141-1149. doi: 10.1038/s41401-020-0485-4
- Essalmani R, Jain J, Susan-Resiga D, et al. Distinctive roles of furin and TMPRSS2 in SARS-CoV-2 infectivity. J Virol. 2022;96(8):e0012822. doi: 10.1128/jvi.00128-22
- Cantuti-Castelvetri L, Ojha R, Pedro LD, et al. Neuropilin-1 facilitates SARS-CoV-2 cell entry and infectivity. Science. 2020;370:856-860. doi: 10.1126/science.abd2985
- Butowt R, von Bartheld CS. The route of SARS-CoV-2 to brain infection: Have we been barking up the wrong tree? Mol Neurodegener. 2022;17(1):20. doi: 10.1186/s13024-022-00529-9
- Paniz-Mondolfi A, Bryce C, Grimes Z, et al. Central nervous system involvement by severe acute respiratory syndrome coronavirus-2 (SARS-CoV-2). J Med Virol. 2020;92(7):699-702. doi: 10.1002/jmv.25915
- Bentivoglio M, Kristensson K, Rottenberg ME. Circumventricular organs and parasite neurotropism: Neglected gates to the brain? Front Immunol. 2018;9:1-9. doi: 10.3389/fimmu.2018.02877
- Hernández-Parra H, Reyes-Hernández OD, Figueroa- González G, et al. Alteration of the blood-brain barrier by COVID-19 and its implication in the permeation of drugs into the brain. Front Cell Neurosci. 2023;17:1125109. doi: 10.3389/fncel.2023.1125109
- Greene C, Connolly R, Brennan D, et al. Blood-brain barrier disruption and sustained systemic inflammation in individuals with long COVID-associated cognitive impairment. Nat Neurosci. 2024;27(3):421-432. doi: 10.1038/s41593-024-01576-9
- Yang Q, Wang G, Zhang F. Role of peripheral immune cells-mediated inflammation on the process of neurodegenerative diseases. Front Immunol. 2020;11:582825. doi: 10.3389/fimmu.2020.582825
- Wentworth DE, Tresnan DB, Turner BC, et al. Cells of human aminopeptidase N (CD13) transgenic mice are infected by human coronavirus-229E in vitro, but not in vivo. Virology. 2005;335(2):185-197. doi: 10.1016/j.virol.2005.02.023
- Li YC, Bai WZ, Hirano N, et al. Neurotropic virus tracing suggests a membranous-coating-mediated mechanism for transsynaptic communication. J Comp Neurol. 2013;521(1):203-212. doi: 10.1002/cne.23171
- Swain O, Romano SK, Miryala R, Tsai J, Parikh V, Umanah GKE. SARS-CoV-2 neuronal invasion and complications: Potential mechanisms and therapeutic approaches. J Neurosci. 2021;41(25):5338-5349. doi: 10.1523/JNEUROSCI.3188-20.2021
- Butowt R, Bilinska K, von Bartheld CS. Olfactory dysfunction in COVID-19: New insights into the underlying mechanisms. Trends Neurosci. 2023;46(1):75-90. doi: 10.1016/j.tins.2022.11.003
- Dubé M, Le Coupanec A, Wong AHM, Rini JM, Desforges M, Talbot PJ. Axonal transport enables neuron-to-neuron propagation of human coronavirus OC43. J Virol. 2018;92:e00404-18. doi: 10.1128/JVI.00404-18
- Vakili K, Fathi M, Yaghoobpoor S, et al. The contribution of gut-brain axis to development of neurological symptoms in COVID-19 recovered patients: A hypothesis and review of literature. Front Cell Infect Microbiol. 2022;12:983089. doi: 10.3389/fcimb.2022.983089
- Winston CN, Goetzl EJ, Akers JC, et al. Prediction of conversion from mild cognitive impairment to dementia with neuronally derived blood exosome protein profile. Alzheimers Dement. 2016;3:63-72. doi: 10.1016/j.dadm.2016.04.001
- Strong MJ. SARS-CoV-2, aging, and Post-COVID-19 neurodegeneration. J Neurochem. 2023;165(2):115-130. doi: 10.1111/jnc.15736
- Ahmed SSSJ, Paramasivam P, Kamath M, Sharma A, Rome S, Murugesan R. Genetic exchange of lung-derived exosome to brain causing neuronal changes on COVID-19 infection. Mol Neurobiol. 2021;58(10):5356-5368. doi: 10.1007/s12035-021-02485-9
- Sergio MC, Ricciardi S, Guarino AM, Giaquinto L, De Matteis MA. Membrane remodeling and trafficking piloted by SARS-CoV-2. Trends Cell Biol. 2024;4:1531-1555. doi: 10.1016/j.tcb.2023.12.006
- Wang M, Zhao Y, Liu J, Li T. SARS-CoV-2 modulation of RIG-I-MAVS signaling: Potential mechanisms of impairment on host antiviral immunity and therapeutic approaches. MedComm Futur Med. 2022;1(2):e29. doi: 10.1002/mef2.29
- Chen Y, Cai H, An Pan J’, et al. Functional screen reveals SARS coronavirus nonstructural protein Nsp14 as a novel Cap N7 methyltransferase. Proc Natl Acad Sci U S A. 2009;106:3484-3489. doi: 10.1073/pnas.0808790106
- Wilamowski M, Sherrell DA, Minasov G, et al. 2′-O methylation of RNA cap in SARS-CoV-2 captured by serial crystallography. Proc Natl Acad Sci U S A. 2021;118:e2100170118. doi: 10.1073/pnas.2100170118/-/DCSupplemental
- Chang LJ, Chen TH. Nsp16 2′-o-mtase in coronavirus pathogenesis: Possible prevention and treatments strategies. Viruses. 2021;13(4):538. doi: 10.3390/v13040538
- Siu KL, Kok KH, Ng MHJ, et al. Severe acute respiratory syndrome coronavirus M protein inhibits type I interferon production by impeding theformation of TRAF3·TANK·TBK1/IKKε complex. J Biol Chem. 2009;284(24):16202-16209. doi: 10.1074/jbc.M109.008227
- Zheng Y, Zhuang MW, Han L, et al. Severe acute respiratory syndrome coronavirus 2 (SARS-CoV-2) membrane (M) protein inhibits type I and III interferon production by targeting RIG-I/MDA-5 signaling. Signal Transduct Target Ther. 2020;5(1):299. doi: 10.1038/s41392-020-00438-7
- Cicaloni V, Costanti F, Pasqui A, Bianchini M, Niccolai N, Bongini P. A bioinformatics approach to investigate structural and non-structural proteins in human coronaviruses. Front Genet. 2022;13:891418. doi: 10.3389/fgene.2022.891418
- Kehrer T, Cupic A, Ye C, et al. Impact of SARS-CoV-2 ORF6 and its variant polymorphisms on host responses and viral pathogenesis. Cell Host Microbe. 2023;31(10):1668-1684.e12. doi: 10.1016/j.chom.2023.08.003
- O’Driscoll M, Ribeiro Dos Santos G, Wang L, et al. Age-specific mortality and immunity patterns of SARS-CoV-2. Nature. 2021;590(7844):140-145. doi: 10.1038/s41586-020-2918-0
- Mavrikaki M, Lee JD, Solomon IH, Slack FJ. Severe COVID-19 is associated with molecular signatures of aging in the human brain. Nat Aging. 2022;2(12):1130-1137. doi: 10.1038/s43587-022-00321-w
- Silva J, Patricio F, Patricio-Martínez A, et al. Neuropathological aspects of SARS-CoV-2 infection: Significance for both Alzheimer’s and Parkinson’s disease. Front Neurosci. 2022;16:867825. doi: 10.3389/fnins.2022.867825
- Cao X, Li W, Wang T, et al. Accelerated biological aging in COVID-19 patients. Nat Commun. 2022;13(1):2135. doi: 10.1038/s41467-022-29801-8
- Lee B, Choi HN, Che YH, et al. SARS-CoV-2 infection exacerbates the cellular pathology of Parkinson’s disease in human dopaminergic neurons and a mouse model. Cell Rep Med. 2024;5(5):101570. doi: 10.1016/j.xcrm.2024.101570
- Huang P, Zhang LY, Tan YY, Chen SD. Links between COVID-19 and Parkinson’s disease/Alzheimer’s disease: Reciprocal impacts, medical care strategies and underlying mechanisms. Transl Neurodegener. 2023;12(1):5. doi: 10.1186/s40035-023-00337-1
- Emrani S, Arain HA, DeMarshall C, Nuriel T. APOE4 is associated with cognitive and pathological heterogeneity in patients with Alzheimer’s disease: A systematic review. Alzheimers Res Ther. 2020;12(1):141. doi: 10.1186/s13195-020-00712-4
- Kuo CL, Pilling LC, Atkins JL, et al. APOE e4 genotype predicts severe COVID-19 in the UK biobank community cohort. J Gerontol A Biol Sci Med Sci. 2020;75(11):2231-2232. doi: 10.1093/gerona/glaa131
- Xia X, Wang Y, Zheng J. COVID-19 and Alzheimer’s disease: How one crisis worsens the other. Transl Neurodegener. 2021;10(1):15. doi: 10.1186/s40035-021-00237-2
- Ferini-Strambi L, Salsone M. COVID-19 and neurological disorders: Are neurodegenerative or neuroimmunological diseases more vulnerable? J Neurol. 2021;268(2):409-419. doi: 10.1007/s00415-020-10070-8
- Monje M, Iwasaki A. The neurobiology of long COVID. Neuron. 2022;110(21):3484-3496. doi: 10.1016/j.neuron.2022.10.006
- Schultheiß C, Willscher E, Paschold L, et al. The IL-1β, IL-6, and TNF cytokine triad is associated with post-acute sequelae of COVID-19. Cell Rep Med. 2022;3(6):100663. doi: 10.1016/j.xcrm.2022.100663
- Pszczołowska M, Walczak K, Misków W, et al. Molecular cross-talk between long COVID-19 and Alzheimer’s disease. Geroscience. 2024;46(3):2885-2899. doi: 10.1007/s11357-024-01096-1
- Yin K, Peluso MJ, Luo X, et al. Long COVID manifests with T cell dysregulation, inflammation and an uncoordinated adaptive immune response to SARS-CoV-2. Nat Immunol. 2024;25(2):218-225. doi: 10.1038/s41590-023-01724-6
- Klein J, Wood J, Jaycox JR, et al. Distinguishing features of long COVID identified through immune profiling. Nature. 2023;623(7985):139-148. doi: 10.1038/s41586-023-06651-y
- Ng CT, Sullivan BM, Teijaro JR, et al. Blockade of interferon beta, but not interferon alpha, signaling controls persistent viral infection. Cell Host Microbe. 2015;17(5):653-661. doi: 10.1016/j.chom.2015.04.005
- Uversky VN, Redwan EM, Makis W, Rubio-Casillas A. IgG4 antibodies induced by repeated vaccination may generate immune tolerance to the SARS-CoV-2 spike protein. Vaccines (Basel). 2023;11(5):991. doi: 10.3390/vaccines11050991
- Trougakos IP, Terpos E, Alexopoulos H, et al. Adverse effects of COVID-19 mRNA vaccines: The spike hypothesis. Trends Mol Med. 2022;28(7):542-554. doi: 10.1016/j.molmed.2022.04.007
- Guo CY, Sun L, Chen XP, Zhang DS. Oxidative stress, mitochondrial damage and neurodegenerative diseases. Neural Regen Res. 2013;8(21):2003-2014. doi: 10.3969/j.issn.1673-5374.2013.21.009
- Silwal P, Kim JK, Kim YJ, Jo EK. Mitochondrial reactive oxygen species: Double-edged weapon in host defense and pathological inflammation during infection. Front Immunol. 2020;11:11649. doi: 10.3389/fimmu.2020.01649
- Duan C, Ma R, Zeng X, et al. SARS-CoV-2 achieves immune escape by destroying mitochondrial quality: Comprehensive analysis of the cellular landscapes of lung and blood specimens from patients with COVID-19. Front Immunol. 2022;13:946731. doi: 10.3389/fimmu.2022.946731
- Wu J, Shi Y, Pan X, et al. SARS-CoV-2 ORF9b inhibits RIG-I-MAVS antiviral signaling by interrupting K63-linked ubiquitination of NEMO. Cell Rep. 2021;34(7):108761. doi: 10.1016/j.celrep.2021.108761
- Lin MM, Liu N, Qin ZH, Wang Y. Mitochondrial-derived damage-associated molecular patterns amplify neuroinflammation in neurodegenerative diseases. Acta Pharmacol Sin. 2022;43(10):2439-2447. doi: 10.1038/s41401-022-00879-6
- Soscia SJ, Kirby JE, Washicosky KJ, et al. The Alzheimer’s disease-associated amyloid β-protein is an antimicrobial peptide. PLoS One. 2010;5(3):e9505. doi: 10.1371/journal.pone.0009505
- Ding Q, Shults NV, Gychka SG, Harris BT, Suzuki YJ. Protein expression of angiotensin-converting enzyme 2 (ACE2) is upregulated in brains with Alzheimer’s disease. Int J Mol Sci. 2021;22(4):1687. doi: 10.3390/ijms22041687
- Tian M, Liu W, Li X, et al. HIF-1α promotes SARS-CoV-2 infection and aggravates inflammatory responses to COVID-19. Signal Transduct Target Ther. 2021;6(1):308. doi: 10.1038/s41392-021-00726-w
- Ajaz S, McPhail MJ, Singh KK, et al. Mitochondrial metabolic manipulation by SARS-CoV-2 in peripheral blood mononuclear cells of patients with COVID-19. Am J Physiol Cell Physiol. 2021;320(1):C57-C65. doi: 10.1152/AJPCELL.00426.2020
- Prasada Kabekkodu S, Chakrabarty S, Jayaram P, et al. Severe acute respiratory syndrome coronaviruses contributing to mitochondrial dysfunction: Implications for post-COVID complications. Mitochondrion. 2023;69:43-56. doi: 10.1016/j.mito.2023.01.005
- Zhang W, Wang G, Xu ZG, et al. Lactate is a natural suppressor of RLR signaling by targeting MAVS. Cell. 2019;178(1):176-189.e15. doi: 10.1016/j.cell.2019.05.003
- Carpenè G, Onorato D, Nocini R, et al. Blood lactate concentration in COVID-19: A systematic literature review. Clin Chem Lab Med. 2022;60(3):332-337. doi: 10.1515/cclm-2021-1115
- Noonong K, Chatatikun M, Surinkaew S, et al. Mitochondrial oxidative stress, mitochondrial ROS storms in long COVID pathogenesis. Front Immunol. 2023;14:1275001. doi: 10.3389/fimmu.2023.1275001
- Sindona C, Schepici G, Contestabile V, Bramanti P, Mazzon E. NOX2 activation in Covid-19: Possible implications for neurodegenerative diseases. Medicina (Lithuania). 2021;57(6):604. doi: 10.3390/medicina57060604
- Montezano AC, Camargo LL, Mary S, et al. SARS-CoV-2 spike protein induces endothelial inflammation via ACE2 independently of viral replication. Sci Rep. 2023;13(1):14086. doi: 10.1038/s41598-023-41115-3
- Liochev SI. Reactive oxygen species and the free radical theory of aging. Free Radic Biol Med. 2013;60:1-4. doi: 10.1016/j.freeradbiomed.2013.02.011
- Xue M, Feng L. The role of unfolded protein response in coronavirus infection and its implications for drug design. Front Microbiol. 2021;12:808593. doi: 10.3389/fmicb.2021.808593
- Wang X, Wang W, Wang T, et al. SARS-CoV-2 ORF8 protein induces endoplasmic reticulum stress-like responses and facilitates virus replication by triggering calnexin: An unbiased study. J Virol. 2023;97(3):e0001123. doi: 10.1128/jvi.00011-23
- Kohli E, Causse S, Baverel V, et al. Endoplasmic reticulum chaperones in viral infection: Therapeutic perspectives. Microbiol Mol Biol Rev. 2021;85:e0003521. doi: 10.1128/MMBR.00035-21
- Wang M, Zhang Y, Li C, Chang W, Zhang L. The relationship between gut microbiota and COVID-19 progression: New insights into immunopathogenesis and treatment. Front Immunol. 2023;14:1180336. doi: 10.3389/fimmu.2023.1180336
- Wong AC, Devason AS, Umana IC, et al. Serotonin reduction in post-acute sequelae of viral infection. Cell. 2023;186(22):4851-4867.e20. doi: 10.1016/j.cell.2023.09.013
- Carabotti M, Scirocco A, Antonietta Maselli M, Severi C. The gut-brain axis: Interactions between enteric microbiota, central and enteric nervous systems. Ann Gastroenterol. 2015;28:203-209.
- Denman CR, Park SM, Jo J. Gut-brain axis: Gut dysbiosis and psychiatric disorders in Alzheimer’s and Parkinson’s disease. Front Neurosci. 2023;17:1268419. doi: 10.3389/fnins.2023.1268419
- Vuille-dit-Bille RN, Liechty KW, Verrey F, Guglielmetti LC. SARS-CoV-2 receptor ACE2 gene expression in small intestine correlates with age. Amino Acids. 2020;52(6-7):1063-1065. doi: 10.1007/s00726-020-02870-z
- Zhang Y, Yan R, Zhou Q. ACE2, B0AT1, and SARS-CoV-2 spike protein: Structural and functional implications. Curr Opin Struct Biol. 2022;74:102388. doi: 10.1016/j.sbi.2022.102388
- Abu-Eid R, Ward FJ. Targeting the PI3K/Akt/mTOR pathway: A therapeutic strategy in COVID-19 patients. Immunol Lett. 2021;240:1-8. doi: 10.1016/j.imlet.2021.09.005
- Ghazanfar H, Kandhi S, Shin D, et al. Impact of COVID-19 on the gastrointestinal tract: A clinical review. Cureus. 2022;14:e23333. doi: 10.7759/cureus.23333
- Yamamoto S, Saito M, Tamura A, Prawisuda D, Mizutani T, Yotsuyanagi H. The human microbiome and COVID-19: A systematic review. PLoS One. 2021;16(6):e0253293. doi: 10.1371/journal.pone.0253293
- Huang X, Hussain B, Chang J. Peripheral inflammation and blood–brain barrier disruption: Effects and mechanisms. CNS Neurosci Ther. 2021;27(1):36-47. doi: 10.1111/cns.13569
- Sencio V, Machado MG, Trottein F. The lung-gut axis during viral respiratory infections: The impact of gut dysbiosis on secondary disease outcomes. Mucosal Immunol. 2021;14(2):296-304. doi: 10.1038/s41385-020-00361-8
- Rodrigues L, Cunha RB, Vassilevskaia T, Viveiros M, Cunha C. Drug repurposing for COVID-19: A review and a novel strategy to identify new targets and potential drug candidates. Molecules. 2022;27(9):2723. doi: 10.3390/molecules27092723
- Hashemian SMR, Pourhanifeh MH, Hamblin MR, Shahrzad MK, Mirzaei H. RdRp inhibitors and COVID-19: Is molnupiravir a good option? Biomedicine and Pharmacotherapy. 2022;146:112517. doi: 10.1016/j.biopha.2021.112517
- Malone B, Urakova N, Snijder EJ, Campbell EA. Structures and functions of coronavirus replication-transcription complexes and their relevance for SARS-CoV-2 drug design. Nat Rev Mol Cell Biol. 2022;23(1):21-39. doi: 10.1038/s41580-021-00432-z
- Abd El-Aziz TM, Al-Sabi A, Stockand JD. Human recombinant soluble ACE2 (hrsACE2) shows promise for treating severe COVID-19. Signal Transduct Target Ther. 2020;5(1):258. doi: 10.1038/s41392-020-00374-6
- Copaescu A, Smibert O, Gibson A, Phillips EJ, Trubiano JA. The role of IL-6 and other mediators in the cytokine storm associated with SARS-CoV-2 infection. J Allergy Clin Immunol. 2020;146(3):518-534.e1. doi: 10.1016/j.jaci.2020.07.001
- Zhang X, Shang L, Fan G, et al. The efficacy and safety of Janus kinase inhibitors for patients with COVID-19: A living systematic review and meta-analysis. Front Med (Lausanne). 2022;8:800492. doi: 10.3389/fmed.2021.800492
- Patel S, Wadhwa M. Therapeutic use of specific tumour necrosis factor inhibitors in inflammatory diseases including COVID-19. Biomed Pharmacother. 2021;140:111785. doi: 10.1016/j.biopha.2021.111785
- Wilhelm G, Mertowska P, Mertowski S, et al. The crossroads of the coagulation system and the immune system: Interactions and connections. Int J Mol Sci. 2023;24(16):12563. doi: 10.3390/ijms241612563
- Ashton NJ, Janelidze S, Mattsson-Carlgren N, et al. Differential roles of Aβ42/40, p-tau231 and p-tau217 for Alzheimer’s trial selection and disease monitoring. Nat Med. 2022;28(12):2555-2562. doi: 10.1038/s41591-022-02074-w
- Hanson BA, Visvabharathy L, Ali ST, et al. Plasma biomarkers of neuropathogenesis in hospitalized patients with COVID-19 and those with postacute sequelae of SARS-CoV-2 infection. Neurol Neuroimmunol Neuroinflamm. 2022;9(3):e1151. doi: 10.1212/NXI.0000000000001151
- Altmann DM, Whettlock EM, Liu S, Arachchillage DJ, Boyton RJ. The immunology of long COVID. Nat Rev Immunol. 2023;23(10):618-634. doi: 10.1038/s41577-023-00904-7
- Merad M, Subramanian A, Wang TT. An aberrant inflammatory response in severe COVID-19. Cell Host Microbe. 2021;29(7):1043-1047. doi: 10.1016/j.chom.2021.06.018
- Gusev E, Sarapultsev A, Solomatina L, Chereshnev V. SARS‐COV‐2‐specific immune response and the pathogenesis of COVID‐19. Int J Mol Sci. 2022;23(3):1716. doi: 10.3390/ijms23031716
- Leblanc P, Vorberg IM. Viruses in neurodegenerative diseases: More than just suspects in crimes. PLoS Pathog. 2022;18(8):e1010670. doi: 10.1371/journal.ppat.1010670
- Rohaim MA, El Naggar RF, Clayton E, Munir M. Structural and functional insights into non-structural proteins of coronaviruses. Microb Pathog. 2021; 150:104641. doi: 10.1016/j.micpath.2020.104641