Targeted drug delivery approaches for the management of tumors
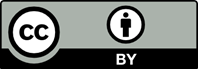
The treatment of tumors requires specific and selective drug delivery approaches capable of efficiently eliminating the root cause of oncogenesis. In this context, targeted drug delivery strategies are the preferred choice due to their exceptional recognition of tumor cell biology and their high transfection efficacy within the tumor microenvironment. The emergence of nanoscale techniques has marked significant milestones in the successful management of various types of tumors. The development of targeted delivery approaches for tumor therapeutics has gained momentum over the past few decades. However, in contrast to the plethora of successful pre-clinical studies, only a limited number of passively targeted nanocarriers have been approved for clinical use, and none of the actively targeted nanocarriers have advanced beyond clinical trials. This review delves into the major molecular principles associated with tumorigenesis, including active targeting, passive targeting, and cell-mediated targeting. It also explores the biological barriers (systemic, biological, and cellular) encountered in tumor drug delivery. Moreover, the review examines various tumor-targeted drug delivery systems that have been developed over the past decade. These systems encompass polymeric nanoparticles (micelles, nanoparticles, dendrimers, and polyplexes), lipid-based carriers (solid lipid nanoparticles, nanostructured lipid carriers, nanoemulsion), vesicular systems (liposomes, niosomes, aquasomes, and phytosomes), and inorganic nanocarriers (iron oxide nanoparticles, quantum dots, carbon-based nanoparticles, and mesoporous silica nanoparticles), all designed for the alleviation of tumors.
- Rankin EB, Giaccia AJ, 2016, Hypoxic control of metastasis. Science, 352: 175–180. https://doi.org/10.1126/science.aaf4405
- Abolhassani H, Wang Y, Hammarström L, et al., 2021, Hallmarks of cancers: Primary antibody deficiency Versus other inborn errors of immunity. Front Immunol, 12: 720025. https://doi.org/10.3389/fimmu.2021.720025
- Mi Y, Mu L, Huang K, et al., 2020, Hypoxic colorectal cancer cells promote metastasis of normoxic cancer cells depending on IL-8/p65 signaling pathway. Cell Death Dis, 11(7): 610. https://doi.org/10.1038/s41419-020-02797-z
- Date AA, Hanes J, Ensign LM, 2016, Nanoparticles for oral delivery: Design, evaluation and state-of-the-art. J Control Release, 240: 504–526. https://doi.org/10.1016/j.jconrel.2016.06.016
- Wang AZ, Langer R, Farokhzad OC, 2012, Nanoparticle delivery of cancer drugs. Annu Rev Med, 63: 185–198. https://doi.org/10.1146/annurev-med-040210-162544
- Gustafson HH, Holt-Casper D, Grainger DW, et al., 2015, Nanoparticle uptake: The phagocyte problem. Nano Today, 10: 487–510. https://doi.org/10.1016/j.nantod.2015.06.006
- Andreone BJ, Chow BW, Tata A, et al., 2017, Blood-brain barrier permeability is regulated by lipid transport-dependent suppression of caveolae-mediated transcytosis. Neuron, 94: 581–594.e5. https://doi.org/10.1016/j.neuron.2017.03.043
- Sanchez-Covarrubias L, Slosky LM, Thompson BJ, et al., 2014, Transporters at CNS barrier sites: Obstacles or opportunities for drug delivery. Curr Pharm Des, 20: 1 422–1449. https://doi.org/10.2174/13816128113199990463
- Wang Y, Cai R, Chen C, 2019, The nano-bio interactions of nanomedicines: Understanding the biochemical driving forces and redox reactions. Acc Chem Res, 52(6): 1507–1518. https://doi.org/10.1021/acs.accounts.9b00126
- Sun B, Sun YY, Li XP, et al., 2019, Particle and bacteria uptake by Japanese flounder (Paralichthys olivaceus) red blood cells: Size dependence and pathway specificity. Tissue Cell, 61: 79–88. https://doi.org/10.1016/j.tice.2019.09.002
- Kutova OM, Guryev EL, Sokolova EA, et al., 2019, Targeted delivery to tumors: Multidirectional strategies to improve treatment efficiency. Cancers (Basel), 11: 68. https://doi.org/10.3390/cancers11010068
- Gu YT, Xue YX, Wang YF, et al., 2012, Role of ROS/RhoA/ PI3K/PKB signaling in NS1619-mediated blood-tumor barrier permeability increase. J Mol Neurosci, 48: 302–312. https://doi.org/10.1007/s12031-012-9789-2
- Deryugina EI, 2016, Chorioallantoic membrane microtumor model to study the mechanisms of tumor angiogenesis, vascular permeability, and tumor cell intravasation. Methods Mol Biol, 1430: 283–298. https://doi.org/10.1007/978-1-4939-3628-1_19
- Uhlen M, Zhang C, Lee S, et al., 2017, A pathology atlas of the human cancer transcriptome. Science, 357: eaan2507. https://doi.org/10.1126/science.aan2507
- Naik H, Sonju JJ, Singh S, et al., 2021, Lipidated peptidomimetic ligand-functionalized HER2 targeted liposome as nano-carrier designed for doxorubicin delivery in cancer therapy. Pharmaceuticals (Basel), 14: 221. https://doi.org/10.3390/ph14030221
- Dvorak HF, 2015, Tumor stroma, tumor blood vessels, and antiangiogenesis therapy. Cancer J, 21: 237–243. https://doi.org/10.1097/PPO.0000000000000124
- Goel S, Chen F, Hong H, et al., 2014, VEGF₁₂₁-conjugated mesoporous silica nanoparticle: A tumor targeted drug delivery system. ACS Appl Mater Interfaces, 6: 21677–21685. https://doi.org/10.1021/am506849p
- Prager GW, Poettler M, Unseld M, et al., 2012, Angiogenesis in cancer: Anti-VEGF escape mechanisms. Transl Lung Cancer Res, 1: 14–25. https://doi.org/10.3978/j.issn.2218-6751.2011.11.02
- Ma X, Xiong Y, Lee LTO, 2018, Application of nanoparticles for targeting G protein-coupled receptors. Int J Mol Sci, 19: 2006. https://doi.org/10.3390/ijms19072006
- Huang B, Abraham WD, Zheng Y, et al., 2015, Active targeting of chemotherapy to disseminated tumors using nanoparticle-carrying T cells. Sci Transl Med, 7: 291ra94. https://doi.org/10.1126/scitranslmed.aaa5447
- Roger M, Clavreul A, Venier-Julienne MC, et al., 2011, The potential of combinations of drug-loaded nanoparticle systems and adult stem cells for glioma therapy. Biomaterials, 32: 2106–2116. https://doi.org/10.1016/j.biomaterials.2010.11.056
- Townsend AR, Chong LC, Karapetis C, et al., 2016, Selective internal radiation therapy for liver metastases from colorectal cancer. Cancer Treat Rev, 50: 148–154. https://doi.org/10.1016/j.ctrv.2016.09.007
- Sangi S, Sree HN, Bawadekji A, et al., 2018, Chemotherapeutic drug targeting to lungs by way of microspheres after intravenous administration. Drug Des Devel Ther, 12: 3051–3060. https://doi.org/10.2147/DDDT.S173485
- Fan R, Li X, Deng J, et al., 2016. Dual drug loaded biodegradable nanofibrous microspheres for improving anti-colon cancer activity. Sci Rep, 6: 28373.
- Shell JR, Gendron LN, LaRochelle EP, et al., 2019, Tumor Targeting Cetuximab Conjugated Europium Microspheres for Cherenkov Excited Luminescence Scanned Imaging (Conference Presentation). In: Proceedings of Molecular- Guided Surgery: Molecules, Devices, and Applications. Vol. 10862. p. 1086217. https://doi.org/10.1117/12.2515383
- Shukla T, Upmanyu N, Agrawal M, et al., 2018, Biomedical applications of microemulsion through dermal and transdermal route. Biomed Pharmacother, 108: 1477–1494. https://doi.org/10.1016/j.biopha.2018.10.021
- Naves LB, Almeida L, Marques MJ, et al., 2017, Emulsions stabilization for topical application. Biomater Med Appl, 1: 1.
- Murthy R, Habbul A, 2006, Trans-arterial hepatic radioembolisation of yttrium-90 microspheres. Biomed Imag Interven J, 2: e43. https://doi.org/10.2349/biij.2.3.e43
- Yehia R, Hathout RM, Attia DA, et al., 2017, Anti-tumor efficacy of an integrated methyl dihydrojasmonate transdermal microemulsion system targeting breast cancer cells: In vitro and in vivo studies. Colloids Surf B Biointerfaces, 1: 512–521. https://doi.org/10.1016/j.colsurfb.2017.04.031
- Enriquez GG, Rizvi SA, D’Souza MJ, et al., 2013, Formulation and evaluation of drug-loaded targeted magnetic microspheres for cancer therapy. Int J Nanomed, 8: 1393–1402. https://doi.org/10.2147/IJN.S43479
- Cordeiro Pedrosa LR, van Tellingen O, Soullié T, et al., 2015, Plasma membrane targeting by short chain sphingolipids inserted in liposomes improves anti-tumor activity of mitoxantrone in an orthotopic breast carcinoma xenograft model. Eur J Pharm Biopharm, 94: 207–219. https://doi.org/10.1016/j.ejpb.2015.05.003
- Han R, Yang YM, Dietrich J, et al., 2008, Systemic 5-fluorouracil treatment causes a syndrome of delayed myelin destruction in the central nervous system. J Biol, 7: 12. https://doi.org/10.1186/jbiol69
- Bardos AP, editor, 2005, Treatment of Ovarian Cancer. New York: Nova Science Publishers Inc., p79–86.
- Woo SM, Joo J, Kim SY, et al., 2016, Efficacy of pancreatic exocrine replacement therapy for patients with unresectable pancreatic cancer in a randomized trial. Pancreatology, 16(6): 1099–1105. https://doi.org/10.1016/j.pan.2016.09.001
- Tamura K, Kikuchi E, Konno T, et al., 2015, Therapeutic effect of intravesical administration of paclitaxel solubilized with poly(2-methacryloyloxyethyl phosphorylcholine-co-n-butyl methacrylate) in an orthotopic bladder cancer model. BMC Cancer, 15: 317. https://doi.org/10.1186/s12885-015-1338-2
- Kapoor DN, Bhatia A, Kaur R, et al., 2015, PLGA: A unique polymer for drug delivery. Ther Deliv, 6: 41–58. https://doi.org/10.4155/tde.14.91
- Florence AT, 2005, Nanoparticle uptake by the oral route: Fulfilling its potential? Drug Discov Today Technol, 2: 75–81. https://doi.org/10.1016/j.ddtec.2005.05.019
- Mittal G, Sahana DK, Bhardwaj V, et al., 2007, Estradiol loaded PLGA nanoparticles for oral administration: Effect of polymer molecular weight and copolymer composition on release behavior in vitro and in vivo. J Control Release, 119: 77–85. https://doi.org/10.1016/j.jconrel.2007.01.016
- Jana S, Maiti S, Jana S, editors, 2017, Biopolymer-Based Composites. United Kingdom: Woodhead Publishing. p221–267.
- He C, Hu Y, Yin L, et al., 2010, Effects of particle size and surface charge on cellular uptake and biodistribution of polymeric nanoparticles. Biomaterials, 31: 3657−3666. https://doi.org/10.1016/j.biomaterials.2010.01.065
- Wang R, Shen Q, Li X, et al., 2018, Efficacy of inverso isomer of CendR peptide on tumor tissue penetration. Acta Pharm Sin B, 8: 825−832. https://doi.org/10.1016/j.apsb.2018.06.006
- Maman S, Witz IP, 2018, A history of exploring cancer in context. Nat Rev Cancer, 18: 359−376. https://doi.org/10.1038/s41568-018-0006-7
- Yu W, Liu R, Zhou Y, et al., 2020, Size-tunable strategies for a tumor targeted drug delivery system. ACS Cent Sci, 6: 100−116.
- Begines B, Ortiz T, Pérez-Aranda M, et al., 2020. Polymeric nanoparticles for drug delivery: Recent developments and future prospects. Nanomaterials (Basel), 10: 1403. https://doi.org/10.3390/nano10071403
- Ni M, Xiong M, Zhang X, et al., 2015, Poly(lactic-co-glycolic acid) nanoparticles conjugated with CD133 aptamers for targeted salinomycin delivery to CD133+ osteosarcoma cancer stem cells. Int J Nanomed, 31: 2537–2554. https://doi.org/10.2147/IJN.S78498
- Banzato A, Bobisse S, Rondina M, et al., 2008, A paclitaxel-hyaluronan bioconjugate targeting ovarian cancer affords a potent in vivo therapeutic activity. Clin Cancer Res, 14: 3598–3606. https://doi.org/10.1158/1078-0432.CCR-07-2019
- Liu Q, Li RT, Qian HQ, et al., 2013, Targeted delivery of miR-200c/DOC to inhibit cancer stem cells and cancer cells by the gelatinases-stimuli nanoparticles. Biomaterials, 34: 7191–7203. https://doi.org/10.1016/j.biomaterials.2013.06.004
- Yang N, Jiang Y, Zhang H, et al., 2015, Active targeting docetaxel-PLA nanoparticles eradicate circulating lung cancer stem-like cells and inhibit liver metastasis. Mol Pharm, 12: 232–239. https://doi.org/10.1021/mp500568z
- Nascimento AV, Singh A, Bousbaa H, et al., 2015, Combinatorial-designed epidermal growth factor receptor-targeted chitosan nanoparticles for encapsulation and delivery of lipid-modified platinum derivatives in wild-type and resistant non-small-cell lung cancer cells. Mol Pharm, 12: 4466–4477. https://doi.org/10.1021/acs.molpharmaceut.5b00642
- Majumder N, Das NG, Das SK, 2020, Polymeric micelles for anticancer drug delivery. Ther Deliv, 11(10): 613–635. https://doi.org/10.4155/tde-2020-0008
- Keskin D, Tezcaner A, 2017, Micelles as delivery system for cancer treatment. Curr Pharm Des, 23(35): 5230–5241. https://doi.org/10.2174/1381612823666170526102757
- Kanamala M, Wilson WR, Yang M, et al., 2016, Mechanisms and biomaterials in pH-responsive tumour targeted drug delivery: A review. Biomaterials, 85: 152–167. https://doi.org/10.1016/j.biomaterials.2016.01.061
- Ge Z, Liu S, 2013, Functional block copolymer assemblies responsive to tumor and intracellular microenvironments for site-specific drug delivery and enhanced imaging performance. Chem Soc Rev, 42: 7289–7325.
- Ke XY, Lin NVW, Gao SJ, et al., 2014, Co-delivery of thioridazine and doxorubicin using polymeric micelles for targeting both cancer cells and cancer stem cells. Biomaterials, 35: 1096–1108. https://doi.org/10.1016/j.biomaterials.2013.10.049
- Bisht S, Feldmann G, Soni S, et al., 2007, Polymeric nanoparticle-encapsulated curcumin (“nanocurcumin”): A novel strategy for human cancer therapy. J Nanobiotechnology, 5: 3. https://doi.org/10.1186/1477-3155-5-3
- Jin X, Mo R, Ding Y, et al., 2014, Paclitaxel-loaded N-octyl- O-sulfate chitosan micelles for superior cancer therapeutic efficacy and overcoming drug resistance. Mol Pharm, 11: 145–157. https://doi.org/10.1021/mp400340k
- Krishnamurthy S, Ng VW, Gao S, et al., 2014, Phenformin-loaded polymeric micelles for targeting both cancer cells and cancer stem cells in vitro and in vivo. Biomaterials, 35: 9177–9186. https://doi.org/10.1016/j.biomaterials.2014.07.018
- Wu P, Jia Y, Qu F, et al., 2017, Ultrasound-responsive polymeric micelles for sonoporation-assisted site-specific therapeutic action. ACS Appl Mater Interfaces, 9: 25706–25716. https://doi.org/10.1021/acsami.7b05469
- Huang R, Wang Y, editors, 2020, New Nanomaterials and Techniques for Tumor-targeted Systems. Berlin: Springer. p337–369.
- Kesharwani P, Iyer AK, 2015, Recent advances in dendrimer-based nanovectors for tumor-targeted drug and gene delivery. Drug Discov Today, 20: 536–547. https://doi.org/10.1016/j.drudis.2014.12.012
- Palmerston ML, Pan J, Torchilin VP, 2017, Dendrimers as nanocarriers for nucleic acid and drug delivery in cancer therapy. Molecules, 22: 1401. https://doi.org/10.3390/molecules22091401
- Liu J, Li J, Liu N, et al., 2017, In vitro studies of phospholipid-modified PAMAM-siMDR1 complexes for the reversal of multidrug resistance in human breast cancer cells. Int J Pharm, 530: 291–299. https://doi.org/10.1016/j.ijpharm.2017.06.026
- Jiang X, Bugno J, Hu C, et al., 2016, Eradication of acute myeloid leukemia with FLT3 ligand-targeted miR-150 nanoparticles. Cancer Res, 76: 4470–4480. https://doi.org/10.1158/0008-5472.CAN-15-2949
- Szulc A, Pulaski, L, Appelhans D, et al., 2016, Sugar-modified poly (propylene imine) dendrimers as drug delivery agents for cytarabine to overcome drug resistance. Int J Pharm, 513: 572–583. https://doi.org/10.1016/j.ijpharm.2016.09.063
- Sheveleva NN, Dolgushev M, Lähderanta E, et al., 2022, Mechanical relaxation of functionalized carbosilane dendrimer melts. Phys Chem Chem Phys, 24: 13049–13056.
- Caminade AM, 2020, Phosphorus dendrimers as nanotools against cancers. Molecules, 25: 3333. https://doi.org/10.3390/molecules25153333
- Chen L, Li J, Fan Y, et al., 2020, Revisiting cationic phosphorus dendrimers as a nonviral vector for optimized gene delivery toward cancer therapy applications. Biomacromolecules, 21: 2502–2511. https://doi.org/10.1021/acs.biomac.0c00458
- Rajasekaran D, Srivastava J, Ebeid K, et al., 2015, Combination of nanoparticle-delivered siRNA for astrocyte elevated gene-1 (AEG-1) and all-trans retinoic acid (ATRA): An effective therapeutic strategy for hepatocellular carcinoma (HCC). Bioconjug Chem, 26: 1651–1661. https://doi.org/10.1021/acs.bioconjchem.5b00254
- Zheng W, Cao C, Liu Y, et al., 2015, Multifunctional polyamidoamine-modified selenium nanoparticles dual-delivering siRNA and cisplatin to A549/DDP cells for reversal multidrug resistance. Acta Biomater, 11: 368–380. https://doi.org/10.1016/j.actbio.2014.08.035
- Sharma A, Gautam SP, Gupta AK, 2011, Surface modified dendrimers: Synthesis and characterization for cancer targeted drug delivery. Bioorg Med Chem, 19: 3341–3346. https://doi.org/10.1016/j.bmc.2011.04.046
- Zhang Y, Thomas TP, Lee KH, et al., 2011, Polyvalent saccharide-functionalized generation 3 poly(amidoamine) dendrimer-methotrexate conjugate as a potential anticancer agent. Bioorg Med Chem, 19: 2557–2564. https://doi.org/10.1016/j.bmc.2011.03.019
- Nam HY, Hahn HJ, Nam K, et al., 2008, Evaluation of generations 2, 3 and 4 arginine modified PAMAM dendrimers for gene delivery. Int J Pharm, 363: 199–205. https://doi.org/10.1016/j.ijpharm.2008.07.021
- Dhakad RS, Tekade RK, Jain NK, 2013, Cancer targeting potential of folate targeted nanocarrier under comparative influence of tretinoin and dexamethasone. Curr Drug Deliv, 10: 477–491. https://doi.org/10.2174/1567201811310040012
- Kesharwani P, Tekade RK, Jain NK, 2014, Formulation development and in vitro-in vivo assessment of the fourth-generation PPI dendrimer as a cancer-targeting vector. Nanomedicine (Lond), 9: 2291–2308. https://doi.org/10.2217/nnm.13.210
- Liu H, Wang Y, Wang M, et al., 2014, Fluorinated poly(propylenimine) dendrimers as gene vectors. Biomaterials, 35: 5407–5413. https://doi.org/10.1016/j.biomaterials.2014.03.040
- Niidome T, Yamauchi H, Takahashi K, et al., 2014, Hydrophobic cavity formed by oligopeptide for doxorubicin delivery based on dendritic poly(L-lysine). J Biomater Sci Polym Ed, 25: 1362–1373. https://doi.org/10.1080/09205063.2014.938979
- Kaminskas LM, McLeod VM, Ryan GM, et al., 2014, Pulmonary administration of a doxorubicin-conjugated dendrimer enhances drug exposure to lung metastases and improves cancer therapy. J Control Release, 183: 18–26. https://doi.org/10.1016/j.jconrel.2014.03.012
- Ma D, Zhao Y, Zhou XY, et al., 2013, Photoenhanced gene transfection by a star-shaped polymer consisting of a porphyrin core and poly(L-lysine) dendron arms. Macromol Biosci, 13: 1221–1227. https://doi.org/10.1002/mabi.201300139
- Zhao J, Zhou R, Fu X, et al., 2014, Cell-penetrable lysine dendrimers for anti-cancer drug delivery: synthesis and preliminary biological evaluation. Arch Pharm (Weinheim), 347: 469–477.
- Ogris M, Wagner E, 2002, Tumor-targeted gene transfer with DNA polyplexes. Somat Cell Mol Genet, 27: 85–95. https://doi.org/10.1023/a:1022988008131
- Lee D, Lee YM, Kim J, et al., 2015, Enhanced tumor-targeted gene delivery by bioreducible polyethylenimine tethering EGFR divalent ligands. Biomater Sci, 3: 1096–1104. https://doi.org/10.1039/c5bm00004a
- Taschauer A, Polzer W, Alioglu F, et al., 2019, Peptide-targeted polyplexes for aerosol-mediated gene delivery to CD49f-overexpressing tumor lesions in lung. Mol Ther Nucleic Acids, 18: 774–786. https://doi.org/10.1016/j.omtn.2019.10.009
- Hattori Y, 2017, Progress in the development of lipoplex and polyplex modified with anionic polymer for efficient gene delivery. J Genet Med Gene Ther, 1: 3–18.
- Chen M, Zeng Z, Qu X, et al., 2015, Biocompatible anionic polyelectrolyte for improved liposome based gene transfection. Int J Pharm, 490: 173–179. https://doi.org/10.1016/j.ijpharm.2015.05.046
- Ito T, Koyama Y, Otsuka M, 2014, Preparation of calcium phosphate nanocapsule including deoxyribonucleic acid-polyethyleneimine-hyaluronic acid ternary complex for durable gene delivery. J Pharm Sci, 103: 179–184.
- Gu J, Chen X, Ren X, et al., 2016, CD44-Targeted hyaluronic acid-coated redox- responsive hyperbranched poly (amido amine)/plasmid DNA ternary nanoassemblies for efficient gene delivery. Bioconjug Chem, 27: 1723–1736. https://doi.org/10.1021/acs.bioconjchem.6b00240
- Mok H, Park JW, Park TG, 2007, Antisense oligodeoxynucleotide-conjugated hyaluronic acid/ protamine nanocomplexes for intracellular gene inhibition. Bioconjug Chem, 18: 1483–1489. https://doi.org/10.1021/bc070111o
- Iwanaga M, Kodama Y, Muro T, et al., 2017, Biocompatible complex coated with glycosaminoglycan for gene delivery. J Drug Target, 25: 370–378. https://doi.org/10.1080/1061186X.2016.1274996
- Liao ZX, Peng SF, Ho YC, et al., 2012, Mechanistic study of transfection of chitosan/DNA complexes coated by anionic poly (gamma-glutamic acid). Biomaterials, 33: 3306–3315. https://doi.org/10.1016/j.biomaterials.2012.01.013
- Kodama Y, Kuramoto H, Mieda Y, et al., 2017, Application of biodegradable dendrigraft poly-l-lysine to a small interfering RNA delivery system. J Drug Target, 25: 49–57. https://doi.org/10.1080/1061186X.2016.1184670
- Boyle WS, Senger K, Tolar J, et al., 2017, Heparin enhances transfection in concert with a trehalose-based polycation with challenging cell types. Biomacromol, 18: 56–67. https://doi.org/10.1021/acs.biomac.6b01297
- Tan GK, Tabata Y, 2014, Chondroitin-6-sulfate attenuates inflammatory responses in murine macrophages via suppression of NF-kappaB nuclear translocation. Acta Biomater, 10: 2684–2692. https://doi.org/10.1016/j.actbio.2014.02.025
- García-Pinel B, Porras-Alcalá C, Ortega-Rodríguez A, et al., 2019, Lipid-based nanoparticles: Application and recent advances in cancer treatment. Nanomaterials (Basel), 9: 638. https://doi.org/10.3390/nano9040638
- Ozpolat B, Sood AK, Lopez-Berestein G, 2014, Liposomal siRNA nanocarriers for cancer therapy. Adv Drug Deliv Rev, 66: 110–116. https://doi.org/10.1016/j.addr.2013.12.008
- Mukherjee S, Ray S, Thakur RS, 2009, Solid lipid nanoparticles: A modern formulation approach in drug delivery system. Indian J Pharm Sci, 71: 349–358. https://doi.org/10.4103/0250-474X.57282
- Iqbal MA, Shadab Md, Sahni JK, et al., 2012, Nanostructured lipid carriers system: Recent advances in drug delivery. J Drug Target, 20: 813–830. https://doi.org/10.3109/1061186X.2012.716845
- Yang R, Gao R, Li F, et al., 2011, The influence of lipid characteristics on the formation, in vitro release, and in vivo absorption of protein-loaded SLN prepared by the double emulsion process. Drug Dev Ind Pharm, 37: 139–148. https://doi.org/10.3109/03639045.2010.497151
- Bhagwat GS, Athawale RB, Gude RP, et al., 2020, Formulation and development of transferrin targeted solid lipid nanoparticles for breast cancer therapy. Front Pharmacol, 11: 614290. https://doi.org/10.3389/fphar.2020.614290
- da Rocha MCO, da Silva PB, Radicchi MA, et al., 2020, Docetaxel-loaded solid lipid nanoparticles prevent tumor growth and lung metastasis of 4T1 murine mammary carcinoma cells. J Nanobiotechnology, 18: 43. https://doi.org/10.1186/s12951-020-00604-7
- Smith T, Affram K, Nottingham EL, et al., 2020, Application of smart solid lipid nanoparticles to enhance the efficacy of 5-fluorouracil in the treatment of colorectal cancer. Sci Rep, 10: 16989. https://doi.org/10.1038/s41598-020-73218-6
- Shuhendler AJ, Prasad P, Leung M, et al., 2012, A novel solid lipid nanoparticle formulation for active targeting to tumor α (v) β(3) integrin receptors reveals cyclic RGD as a double-edged sword. Adv Healthc Mater, 1: 600–608. https://doi.org/10.1002/adhm.201200006
- Chuang CH, Wu PC, Tsai TH, et al., 2017, Development of pH-sensitive cationic PEGylated solid lipid nanoparticles for selective cancer-targeted therapy. J Biomed Nanotechnol, 13: 192–203. https://doi.org/10.1166/jbn.2017.2338
- Wang W, Zhang L, Chen T, et al., 2017, Anticancer effects of resveratrol-loaded solid lipid nanoparticles on human breast cancer cells. Molecules, 22: 1814. https://doi.org/10.3390/molecules22111814
- Miao J, Du Y, Yuan H, et al., 2015, Improved cytotoxicity of paclitaxel loaded in nanosized lipid carriers by intracellular delivery. J Nanopart Res, 17: 10.
- Khosa A, Reddi S, Saha RN, 2018, Nanostructured lipid carriers for site-specific drug delivery. Biomed Pharmacother, 103: 598–613. https://doi.org/10.1016/j.biopha.2018.04.055
- Varshosaz J, Taymouri S, Jahanian-Najafabadi A, et al., 2018, Efavirenz oral delivery via lipid nanocapsules: Formulation, optimisation, and ex-vivo gut permeation study. IET Nanobiotechnol, 12: 795–806. https://doi.org/10.1049/iet-nbt.2018.0006
- Zhang XG, Miao J, Dai YQ, et al., 2008, Reversal activity of nanostructured lipid carriers loading cytotoxic drug in multi-drug resistant cancer cells. Int J Pharm, 361: 239–244. https://doi.org/10.1016/j.ijpharm.2008.06.002
- Wang Y, Zhang H, Hao J, et al., 2016, Lung cancer combination therapy: Co-delivery of paclitaxel and doxorubicin by nanostructured lipid carriers for synergistic effect. Drug Deliv, 23: 1398–1403. https://doi.org/10.3109/10717544.2015.1055619
- Mussi SV, Sawant R, Perche F, et al., 2014, Novel nanostructured lipid carrier co-loaded with doxorubicin and docosahexaenoic acid demonstrates enhanced in vitro activity and overcomes drug resistance in MCF-7/Adr cells. Pharm Res, 31: 1882–1892. https://doi.org/10.1007/s11095-013-1290-2
- Wang JY, Song YQ, Peng J, et al., 2020, Nanostructured lipid carriers delivering sorafenib to enhance immunotherapy induced by doxorubicin for effective esophagus cancer therapy. ACS Omega, 5: 22840–22846. https://doi.org/10.1021/acsomega.0c02072
- Li X, Jia X, Niu H, 2018, Nanostructured lipid carriers co-delivering lapachone and doxorubicin for overcoming multidrug resistance in breast cancer therapy. Int J Nanomedicine, 13: 4107–4119. https://doi.org/10.2147/IJN.S163929
- Liu Q, Li J, Pu G, et al., 2016, Co-delivery of baicalein and doxorubicin by hyaluronic acid decorated nanostructured lipid carriers for breast cancer therapy. Drug Deliv, 23: 1364–1368. https://doi.org/10.3109/10717544.2015.1031295
- Li Z, Zou X, Zhu H, et al., 2018, Inhibitory effect of baicalein combined with gemcitabine in human pancreatic cancer cell lines. Oncol Lett, 15: 5459–5464. https://doi.org/10.3892/ol.2018.8043
- Ni S, Qiu L, Zhang G, et al., 2017, Lymph cancer chemotherapy: Delivery of doxorubicin-gemcitabine prodrug and vincristine by nanostructured lipid carriers. Int J Nanomedicine, 12: 1565–1576. https://doi.org/10.2147/IJN.S120685
- Ding X, Xu X, Zhao Y, et al., 2017, Tumor targeted nanostructured lipid carrier co-delivering paclitaxel and indocyanine green for laser triggered synergetic therapy of cancer. RSC Adv, 7: 35086–35095.
- Yang J, Ju Z, Dong S, 2016, Cisplatin and paclitaxel co-delivered by folate-decorated lipid carriers for the treatment of head and neck cancer. Drug Deliv, 24: 792–799. https://doi.org/10.1080/10717544.2016.1236849
- Sánchez-López E, Guerra M, Dias-Ferreira J, et al., 2019, Current applications of nanoemulsions in cancer therapeutics. Nanomaterials (Basel), 9: 821. https://doi.org/10.3390/nano9060821
- Ganta S, Singh A, Rawal Y, et al., 2016, Formulation development of a novel targeted theranostic nanoemulsion of docetaxel to overcome multidrug resistance in ovarian cancer. Drug Deliv, 23: 968–980. https://doi.org/10.3109/10717544.2014.923068
- Zheng N, Gao Y, Ji H, et al., 2016, Vitamin E derivative-based multifunctional nanoemulsions for overcoming multidrug resistance in cancer. J Drug Target, 24: 663–669. https://doi.org/10.3109/1061186X.2015.1135335
- Ahmad G, El-Sadda R, Botchkina G, et al., 2017, Nanoemulsion formulation of a novel taxoid DHA-SBT-1214 inhibits prostate cancer stem cell-induced tumor growth. Cancer Lett, 406: 71–80. https://doi.org/10.1016/j.canlet.2017.08.004
- Bozzuto G, Molinari A, 2015, Liposomes as nanomedical devices. Int J Nanomedicine, 10: 975–999. https://doi.org/10.2147/IJN.S68861
- Gonda A, Zhao N, Shah J, et al., 2019, Engineering tumor-targeting nanoparticles as vehicles for precision nanomedicine. Med One, 4: e190021. https://doi.org/10.20900/mo.20190021
- Eloy JO, Petrilli R, Chesca DL, et al., 2017, Anti-HER2 immunoliposomes for co-delivery of paclitaxel and rapamycin for breast cancer therapy. Eur J Pharm Biopharm, 115: 159–167. https://doi.org/10.1016/j.ejpb.2017.02.020
- Prasad R, Jain NK, Yadav AS, et al., 2020, Liposomal nanotheranostics for multimode targeted in vivo bioimaging and near-infrared light mediated cancer therapy. Commun Biol, 3: 284. https://doi.org/10.1038/s42003-020-1016-z
- Yin W, Zhao Y, Kang X, et al., 2020, BBB-penetrating codelivery liposomes treat brain metastasis of non-small cell lung cancer with EGFRT790M mutation. Theranostics, 10: 6122–6135. https://doi.org/10.7150/thno.42234
- Gharbavi M, Amani J, Kheiri-Manjili H, et al., 2018, Niosome: A promising nanocarrier for natural drug delivery through blood-brain barrier. Adv Pharmacol Sci, 2018: 6847971.
- Mohamad Saimi NI, Salim N, Ahmad N, et al., 2021, Aerosolized niosome formulation containing gemcitabine and cisplatin for lung cancer treatment: Optimization, characterization and in vitro evaluation. Pharmaceutics, 13: 59. https://doi.org/10.3390/pharmaceutics13010059
- Kulkarni P, Rawtani D, 2019, Application of box-behnken design in the preparation, optimization, and in vitro evaluation of self-assembly-based tamoxifen- and doxorubicin-loaded and dual drug-loaded niosomes for combinatorial breast cancer treatment. J Pharm Sci, 108: 2643–2653. https://doi.org/10.1016/j.xphs.2019.03.020
- Seleci DA, Seleci M, Walter JG, et al., 2016, Niosomes as nanoparticular drug carriers. J Nanomaterials, 2016: 7372306.
- Ruoslahti E, 2012, Peptides as targeting elements and tissue penetration devices for nanoparticles. Adv Mater, 24: 3747–3756. https://doi.org/10.1002/adma.201200454
- Dahir L, Kajol Kaur A, Manocha A, et al., 2020, Aquasomes: Water like bodies vesicular system for therapeutics molecules as robust system for delivery. EJMCM, 7: 2585–2607.
- Gaikwad SS, Morade YY, Kothule AM, et al., 2023, Overview of phytosomes in treating cancer: Advancement, challenges, and future outlook. Heliyon, 9: e16561. https://doi.org/10.1016/j.heliyon.2023.e16561
- Azeez NA, Deepa VS, Sivapriya V, 2018, Phytosomes: Emergent promising nano vesicular drug delivery system for targeted tumor therapy. Adv Nat Sci Nanosci Nanotechnol, 9: 033001.
- Dancey JE, 2005, Inhibitors of the mammalian target of rapamycin. Expert Opin Investig Drugs, 14: 313–328. https://doi.org/10.1517/13543784.14.3.313
- Stark FC, Weeratna RD, Deschatelets L, et al., 2017, An archaeosome-adjuvanted vaccine and checkpoint inhibitor therapy combination significantly enhances protection from murine melanoma. Vaccines (Basel), 5: 38. https://doi.org/10.3390/vaccines5040038
- Lee YK, Lee TS, Song IH, et al., 2015, Inhibition of pulmonary cancer progression by epidermal growth factor receptor-targeted transfection with Bcl-2 and survivin siRNAs. Cancer Gene Ther, 22: 335–343. https://doi.org/10.1038/cgt.2015.18
- Yang Y, Yang Y, Xie X, et al., 2016, Dual stimulus of hyperthermia and intracellular redox environment triggered release of siRNA for tumor-specific therapy. Int J Pharm, 506: 158–173. https://doi.org/10.1016/j.ijpharm.2016.04.035
- Karpuz M, Silindir-Gunay M, Ozer AY, et al., 2021, Diagnostic and therapeutic evaluation of folate-targeted paclitaxel and vinorelbine encapsulating theranostic liposomes for non-small cell lung cancer. Eur J Pharm Sci, 56: 105576. https://doi.org/10.1016/j.ejps.2020.105576
- Tavano L, Mauro L, Naimo GD, et al., 2016, Further evolution of multifunctional niosomes based on pluronic surfactant: Dual active targeting and drug combination properties. Langmuir, 32: 8926–8933. https://doi.org/10.1021/acs.langmuir.6b02063
- Khan DH, Bashir S, Correia A, et al., 2019, Utilization of green formulation technique and efficacy estimation on cell line studies for dual anticancer drug therapy with niosomes. Int J Pharm, 572: 118764. https://doi.org/10.1016/j.ijpharm.2019.118764
- Shaker DS, Shaker MA, Hanafy MS, 2015, Cellular uptake, cytotoxicity and in-vivo evaluation of Tamoxifen citrate loaded niosomes. Int J Pharm, 493: 285–294. https://doi.org/10.1016/j.ijpharm.2015.07.041
- Tan DMY, Fu JY, Wong FS, et al., 2017, Tumor regression and modulation of gene expression via tumor-targeted tocotrienol niosomes. Nanomedicine (Lond), 20: 2487–2502. https://doi.org/10.2217/nnm-2017-0182
- Li Y, Wu H, Jia M, et al., 2014, Therapeutic effect of folate-targeted and PEGylated phytosomes loaded with a mitomycin C-soybean phosphatidyhlcholine complex. Mol Pharm, 11: 3017–3026. https://doi.org/10.1021/mp5001873
- Alhakamy NA, Badr-Eldin SM, Fahmy UA, et al., 2020, Thymoquinone-loaded soy-phospholipid-based phytosomes exhibit anticancer potential against human lung cancer cells. Pharmaceutics, 2: 761. https://doi.org/10.3390/pharmaceutics12080761
- Nazeer AA, Veeraiyan S, Vijaykumar SD, 2017, Anti-cancer potency and sustained release of phytosomal diallyl disulfide containing methanolic allium sativum extract against breast cancer. Int Res J Pharm, 8: 34–40.
- Pasqua L, Leggio A, Sisci D, et al., 2016, Mesoporous silica nanoparticles in cancer therapy: Relevance of the targeting function. Mini Rev Med Chem, 16: 743–753. https://doi.org/10.2174/1389557516666160321113620
- Tang XL, Jing F, Lin BL, et al., 2018, pH-responsive magnetic mesoporous silica-based nanoplatform for synergistic photodynamic therapy/chemotherapy. ACS Appl Mater Interfaces, 10: 15001–15011. https://doi.org/10.1021/acsami.7b19797
- Lin G, Mi P, Chu C, et al., 2016, Inorganic nanocarriers overcoming multidrug resistance for cancer theranostics. Adv Sci (Weinh), 3: 1600134. https://doi.org/10.1002/advs.201600134
- Reczyńska K, Marszałek M, Zarzycki A, et al., 2020, Superparamagnetic iron oxide nanoparticles modified with silica layers as potential agents for lung cancer treatment. Nanomaterials (Basel), 10: 1076. https://doi.org/10.3390/nano10061076
- Zhi D, Yang T, Yang J, et al., 2020, Targeting strategies for superparamagnetic iron oxide nanoparticles in cancer therapy. Acta Biomater, 102: 13–34. https://doi.org/10.1016/j.actbio.2019.11.027
- Wang X, Zhou Z, Wang Z, et al., 2013, Gadolinium embedded iron oxide nanoclusters as T1-T2 dual-modal MRI-visible vectors for safe and efficient siRNA delivery. Nanoscale, 5: 8098–8104. https://doi.org/10.1039/c3nr02797j
- Jin R, Lin B, Li D, et al., 2014, Superparamagnetic iron oxide nanoparticles for MR imaging and therapy: Design considerations and clinical applications. Curr Opin Pharmacol, 18: 18–27. https://doi.org/10.1016/j.coph.2014.08.002
- Ock K, Jeon WI, Ganbold EO, et al., 2012, Real-time monitoring of glutathione-triggered thiopurine anticancer drug release in live cells investigated by surface-enhanced Raman scattering. Anal Chem, 84: 2172–2178. https://doi.org/10.1021/ac2024188
- Lin G, Zhu W, Yang L, et al., 2014, Delivery of siRNA by MRI-visible nanovehicles to overcome drug resistance in MCF-7/ADR human breast cancer cells. Biomaterials, 35: 9495–9507. https://doi.org/10.1016/j.biomaterials.2014.07.049
- Zhou Z, Huang D, Bao J, et al., 2012, A synergistically enhanced T (1) -T(2) dual-modal contrast agent. Adv Mater, 24: 6223–6228.
- Lee SM, Kim HJ, Kim SY, et al., 2014, Drug-loaded gold plasmonic nanoparticles for treatment of multidrug resistance in cancer. Biomaterials, 35: 2272–2282. https://doi.org/10.1016/j.biomaterials.2013.11.068
- Liu G, Wang Z, Lu J, et al., 2011, Low molecular weight alkyl-polycation wrapped magnetite nanoparticle clusters as MRI probes for stem cell labeling and in vivo imaging. Biomaterials, 32: 528–537. https://doi.org/10.1016/j.biomaterials.2010.08.099
- Ren Y, Zhang H, Chen B, et al., 2012, Multifunctional magnetic Fe3O4 nanoparticles combined with chemotherapy and hyperthermia to overcome multidrug resistance. Int J Nanomedicine, 7: 2261–2269. https://doi.org/10.2147/IJN.S29357
- Li JM, Wang YY, Zhao MX, et al., 2011, Multifunctional QD-based co-delivery of siRNA and doxorubicin to HeLa cells for reversal of multidrug resistance and real-time tracking. Biomaterials, 33: 2780–2790. https://doi.org/10.1016/j.biomaterials.2011.12.035
- Zhang MZ, Li C, Fang BY, et al., 2014, High transfection efficiency of quantum dot-antisense oligonucleotide nanoparticles in cancer cells through dual-receptor synergistic targeting. Nanotechnology, 25: 255102. https://doi.org/10.1088/0957-4484/25/25/255102
- Wang Y, Yang C, Hu R, et al., 2015, Assembling Mn: ZnSe quantum dots-siRNA nanoplexes for gene silencing in tumor cells. Biomater Sci, 3: 192–202.
- Wu CH, Cao C, Kim JH, et al., 2012, Trojan-horse nanotube on-command intracellular drug delivery. Nano Lett, 12: 5475–5480. https://doi.org/10.1021/nl301865c
- Gong H, Peng R, Liu Z, 2013, Carbon nanotubes for biomedical imaging: The recent advances. Adv Drug Deliv Rev, 65: 1951–1963. https://doi.org/10.1016/j.addr.2013.10.002
- Cheng J, Meziani MJ, Sun YP, et al., 2011, Poly (ethylene glycol)-conjugated multi-walled carbon nanotubes as an efficient drug carrier for overcoming multidrug resistance. Toxicol Appl Pharmacol, 250: 184–193. https://doi.org/10.1016/j.taap.2010.10.012
- Lee CH, Cheng SH, Wang YJ, et al., 2009, Near-infrared mesoporous silica nanoparticles for optical imaging: Characterization and in vivo biodistribution. Adv Funct Mater, 19: 215–222.
- Gao Y, Chen Y, Ji X, et al., 2011, Controlled intracellular release of doxorubicin in multidrug-resistant cancer cells by tuning the shell-pore sizes of mesoporous silica nanoparticles. ACS Nano, 5: 9788–9798. https://doi.org/10.1021/nn2033105
- Zhao P, Li L, Zhou S, et al., 2018, TPGS functionalized mesoporous silica nanoparticles for anticancer drug delivery to overcome multidrug resistance. Mater Sci Eng C Mater Biol Appl, 84: 108–117. https://doi.org/10.1016/j.msec.2017.11.040
- Liu J, Wang B, Hartono SB, et al., 2012, Magnetic silica spheres with large nanopores for nucleic acid adsorption and cellular uptake. Biomaterials, 33: 970–978. https://doi.org/10.1016/j.biomaterials.2011.10.001
- Wu P, Zhao T, Wang S, et al., 2014, Semiconductor quantum dots-based metal ion probes. Nanoscale, 6: 43–64.
- Nifontova G, Ramos GF, Baryshnikova M, et al., 2019, Cancer cell targeting with functionalized quantum dot-encoded polyelectrolyte microcapsules. Front Chem, 7: 34. https://doi.org/10.3389/fchem.2019.00034
- AbdElhamid AS, Zayed DG, Helmy MW, et al., 2018, Lactoferrin-tagged quantum dots-based theranostic nanocapsules for combined COX-2 inhibitor/herbal therapy of breast cancer. Nanomedicine (Lond), 13: 2637–2656. https://doi.org/10.2217/nnm-2018-0196
- He H, Xie C, Ren J, 2008, Nonbleaching fluorescence of gold nanoparticles and its applications in cancer cell imaging. Anal Chem, 80: 5951–5957. https://doi.org/10.1021/ac8005796
- Albertini B, Mathieu V, ci N, et al., 2019, Tumor targeting by peptide-decorated gold nanoparticles. Mol Pharm, 16: 2430–2444. https://doi.org/10.1021/acs.molpharmaceut.9b00047