Targeting the interplay between biomolecular condensates and regulatory RNAs in cancer
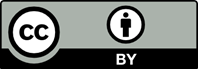
Biomolecular condensates (BCs), RNA–protein complexes, have emerged as potential therapeutic targets for various cellular pathologies, including cancer. The mechanisms underlying liquid–liquid phase separation rely on the properties of proteins with intrinsically disordered regions and prion-like domains, which drive phase separation in conjunction with their RNA partners. These ribonucleoprotein complexes are distinct in their localization, compartmentalization, epigenetic regulation, and dynamics. BCs are categorized as either nuclear—such as promyelocytic leukemia nuclear bodies, speckles, paraspeckles, and Cajal bodies—or cytosolic, including stress granules (SGs), P bodies, and U bodies. Regulatory RNAs, assembled with protein partners through phase separation, exhibit oncogenic properties and perform key biological functions, including gene transcription, euchromatin/heterochromatin formation, mRNA splicing, mRNA translation, protein compartmentalization, and degradation. Epitranscriptome-modifying enzymes regulate the stability and oncogenic potential of noncoding RNAs (ncRNAs). These RNAs, along with their associated epitranscriptomic and protein modifications, play critical roles in the functioning and dissolution of condensates. Recent advancements in cancer therapy have focused on developing drugs targeting the epitranscriptomic machinery, including the writers, readers, and erasers of RNA modifications. Therapeutic strategies aim to target oncogenic RNAs, tumor-promoting proteins, and RNA–protein interaction domains. Several cancer therapeutic compounds have been developed using the PROTAC and RIBOTAC approaches. Moreover, small molecules targeting protein–RNA interactions and antisense oligonucleotides have been developed. Promising avenues in cancer therapeutics involve the inhibition of ncRNAs and their associated protein complexes, modulation of BCs, regulation of SGs and paraspeckles, and development of small molecule compounds with potential applications across various cancer types.
- Boeynaems S, Alberti S, Fawzi NL, et al. Protein phase separation: A new phase in cell biology. Trends Cell Biol. 2018;28(6) 420-435. doi: 10.1016/j.tcb.2018.02.004
- Banani SF, Lee HO, Hyman AA, Rosen MK. Biomolecular condensates: Organizers of cellular biochemistry. Nat Rev Mol Cell Biol. 2017;18(5):285-298. doi: 10.1038/nrm.2017.7
- Guillén-Boixet J, Kopach A, Holehouse AS, et al. RNA-induced conformational switching and clustering of G3BP drive stress granule assembly by condensation. Cell. 2020;181:346-361.e17. doi: 10.1016/j.cell.2020.03.049
- Rhine K, Odeh HM, Shorter J, Myong S. Regulation of biomolecular condensates by Poly(ADP-ribose). Chem Rev. 2023;123(14):9065-9093. doi: 10.1021/acs.chemrev.2c00851
- Fay MM, Anderson PJ. The role of RNA in biological phase separations. J Mol Biol. 2018;430(23):4685-4701. doi: 10.1016/j.jmb.2018.05.003
- Lavalée M, Curdy N, Laurent C, Fournié JJ, Franchini DM. Cancer cell adaptability: Turning ribonucleoprotein granules into targets. Trends Cancer. 2021;7(10):902-915. doi: 10.1016/j.trecan.2021.05.006
- Taniue K, Akimitsu N. Aberrant phase separation and cancer. FEBS J. 2022;289(1):17-39. doi: 10.1111/febs.15765
- Mullard A. Biomolecular condensates pique drug discovery curiosity. Nat Rev Drug Discov. 2019;18:324-326. doi: 10.1038/d41573-019-00069-w
- Conti BA, Oppikofer M. Biomolecular condensates: New opportunities for drug discovery and RNA therapeutics. Trends Pharmacol Sci. 2022;43(10):820-837. doi: 10.1016/j.tips.2022.07.001
- Chakravarty AK, Mcgrail DJ, Lozanoski TM, et al. Biomolecular condensation: A new phase in cancer research. Cancer Discov. 2022;12:2031-2043. doi: 10.1158/2159-8290.CD-21-1605
- Silva JL, Foguel D, Ferreira VF, et al. Targeting biomolecular condensation and protein aggregation against cancer. Chem Rev. 2023;123(14):9094-9138. doi: 10.1021/acs.chemrev.3c00131
- Kilgore HR, Young RA. Learning the chemical grammar of biomolecular condensates. Nat Chem Biol. 2022;18(12):1298-1306. doi: 10.1038/s41589-022-01046-y
- Maqsood Q, Sumrin A, Saleem MZ, et al. An insight into cancer from biomolecular condensates. Cancer Screen Prevent. 2023;2(3):177-190. doi: 10.14218/CSP.2023.00018
- Ginell GM, Holehouse AS. An introduction to the stickers-and-spacers framework applied to biomolecular condensates. Methods Mol Biol. 2023;2563:95-116. doi: 10.1007/978-1-0716-2663-4_4
- Otis JP, Mowry KL. Hitting the mark: Localization of mRNA and biomolecular condensates in health and disease. Wiley Interdiscipl Rev RNA. 2023;14(6):e1807. doi: 10.1002/wrna.1807
- Villegas JA, Heidenreich M, Levy ED. Molecular and environmental determinants of biomolecular condensate formation. Nat Chem Biol. 2022;18(12):1319-1329. doi: 10.1038/s41589-022-01175-4
- Schmidt HB, Görlich D. Transport selectivity of the nuclear pores, phase separation, and membraneless organelles. Trends Biochem Sci. 2016;41(1):46-61. doi: 10.1016/j.tibs.2015.11.001
- Forman-Kay JD, Ditlev JA, Nosella ML, Lee HO. What are the distinguishing features and size requirements of biomolecular condensates and their implications for RNA-containing condensates? RNA. 2022;28:36-47. doi: 10.1261/rna.079026.121
- Kwon I, Kato M, Xiang S, et al. Phosphorylation-regulated binding of RNA polymerase II to fibrous polymers of low-complexity domains. Cell. 2013;155(5):1049. doi: 10.1016/j.cell.2013.10.033
- Peran I, Mittag T. Molecular structure of the biomolecular condensates. Curr Opin Struct Biol. 2020;60:17-26. doi: 10.1016/j.sbi.2019.09.007
- Holmes KJ, Klass DM, Guiney EL, Cyert MS. Whi3, an S. cerevisiae RNA-binding protein, is a component of stress granules that regulates levels of its target mRNAs. PLoS One. 2013;8(12):e84060. doi: 10.1371/journal.pone.0084060
- Ukmar-Godec T, Wegmann S, Zweckstetter M. Biomolecular condensation of the microtubule-associated protein tau. Semin Cell Dev Biol. 2020;99:202-214. doi: 10.1016/j.semcdb.2019.06.007
- Ukmar-Godec T, Hutten S, Grieshop MP, et al. Lysine/RNA-interactions drive and regulate biomolecular condensation. Nat Commun. 2019;10(1):2909. doi: 10.1038/s41467-019-10792-y
- Boyko S, Surewicz WK. Tau liquid-liquid phase separation in neurodegenerative diseases. Trends Cell Biol. 2022;32(7):611-623. doi: 10.1016/j.tcb.2022.01.011
- Abasi LS, Elathram N, Movva M, Deep A, Corbett KD, Debelouchina GT. Phosphorylation regulates tau’s phase separation behavior and interactions with chromatin. Commun Biol. 2024;7(1):251. doi: 10.1038/s42003-024-05920-4
- Parolini F, Tira R, Barracchia CG, et al. Ubiquitination of Alzheimer’s-related tau protein affects the liquid-liquid phase separation in a site- and cofactor-dependent manner. Int J Biol Macromol. 2022;201:173-181. doi: 10.1016/j.ijbiomac.2021.12.191
- Farooqi AA, Fayyaz S, Poltronieri P, Calin G, Mallardo M. Epigenetic deregulation in cancer: Enzyme players and noncoding RNAs. Semin Cancer Biol. 2022;83:197-207. doi: 10.1016/j.semcancer.2020.07.013
- Mattick JS, Amaral PP, Carninci P, et al. Long noncoding RNAs: Definitions, functions, challenges and recommendations. Nat Rev Mol Cell Biol. 2023;24:430-447. doi: 10.1038/s41580-022-00566-8
- Poltronieri P. Regulatory RNAs: Role as scaffolds assembling protein complexes and their epigenetic deregulation. Explor Target Antitumor Ther. 2024;5(4):841-876. doi: 10.37349/etat.2024.00252
- Cusenza VY, Tameni A, Neri A, Frazzi R. lncRNA epigenetics: The significance of m6A and m5C lncRNA modifications in cancer. Front Oncol. 2023;13:1063636. doi: 10.3389/fonc.2023.1063636
- Bure IV, Nemtsova MV, Kuznetsova EB. Histone modifications and non-coding RNAs: Mutual epigenetic regulation and role in pathogenesis. Int J Mol Sci. 2022;23:5801. doi: 10.3390/ijms23105801
- Yang X, Liu M, Li M, et al. Epigenetic modulations of noncoding RNA: A novel dimension of Cancer biology. Mol Cancer. 2020;19:64. doi: 10.1186/s12943-020-01159-9
- Gibson BA, Doolittle LK, Schneider WG, Gerlich DW, Redding S, Rosen MK. Organization of chromatin by intrinsic and regulated phase separation. Cell. 2019;179:470-484. doi: 10.1016/j.cell.2019.08.037
- Nylund P, Garrido-Zabala B, Kalushkova A, Wiklund HJ. The complex nature of lncRNA-mediated chromatin dynamics in multiple myeloma. Front Oncol. 2023;13:1303677. doi: 10.3389/fonc.2023.1303677
- Hu WL, Jin L, Xu A, et al. GUARDIN is a p53-responsive long noncoding RNA that is essential for genomic stability. Nat Cell Biol. 2018;20:492-502. doi: 10.1038/s41556-018-0066-7
- Kurup JT, Kidder BL. Identification of H4K20 me3-and H3K4 me3-associated RNAs using CARIP-Seq expands the transcriptional and epigenetic networks of embryonic stem cells. J Biol Chem. 2018;293(39):15120-15135. doi: 10.1074/jbc.RA118.004974
- Agredo A, Kasinski AL. Histone 4 lysine 20 tri-methylation: A key epigenetic regulator of chromatin structure and disease. Front Genet. 2023;14:1243395. doi: 10.3389/fgene.2023.1243395
- Fan L, Sun W, Lyu Y, et al. Chrom-seq identifies RNAs at chromatin marks. Sci Adv. 2024;10(31):eadn1397. doi: 10.1126/sciadv.adn1397
- Ahmad A, Poltronieri P, Uddin S. Editorial: LncRNAs in cancer metastasis and therapy resistance. Front Oncol. 2021;11:813274. doi: 10.3389/fonc.2021.813274
- Zang C, Nie FQ, Wang Q, et al. Long noncoding RNA LINC01133 represses KLF2, P21 and E-cadherin transcription through binding with EZH2 and LSD1 in non-small cell lung cancer. Oncotarget. 2016;7:11696-11707. doi: 10.18632/oncotarget.7077
- Kong Y, Hsieh CH, Alonso LC. ANRIL: A lncRNA at the CDKN2A/B locus with roles in cancer and metabolic disease. Front Endocrinol (Lausanne). 2018;9:405. doi: 10.3389/fendo.2018.00405
- Lian Y, Yan C, Ding J, et al. A novel lncRNA, LL22NC03- N64E9.1, represses KLF2 transcription by binding with EZH2 in colorectal cancer. Oncotarget. 2017;8:59435-59445. doi: 10.18632/oncotarget.19738
- Quagliata L, Matter MS, Piscuoglio S, et al. Long noncoding RNA HOTTIP/HOXA13 expression is associated with disease progression and predicts the outcome in hepatocellular carcinoma patients. Hepatology. 2014;59:911-923. doi: 10.1002/hep.26740
- Zhang G, Xu Y, Zou C, et al. The long noncoding RNA ARHGAP27P1 inhibits gastric cancer cell proliferation and cell cycle progression by epigenetically regulating p15 and p16. Aging (Albany NY). 2019;11:9090-9110. doi: 10.18632/aging.102377
- Bai Z, Wu Y, Bai S, et al. Long noncoding RNA SNHG7 Is activated by SP1 and exerts oncogenic properties by interacting with EZH2 in ovarian cancer. J Cell Mol Med. 2020;24:7479-7489. doi: 10.1111/jcmm.15373
- Huang MD, Chen WM, Qi FZ, et al. Long noncoding RNA TUG1 is upregulated in hepatocellular carcinoma and promotes cell growth and apoptosis by epigenetically silencing KLF2. Mol Cancer. 2015;14:165. doi: 10.1186/s12943-015-0431-0
- Ruan J, Zheng L, Hu N, et al. Long noncoding RNA SNHG6 promotes osteosarcoma cell proliferation by regulating p21 and KLF2. Arch Biochem Biophys. 2018;646:128-136. doi: 10.1016/j.abb.2018.03.036
- Lian Y, Yang J, Lian Y, Xiao C, Hu X, Xu H. DUXAP8, a pseudogene-derived lncRNA, promotes the growth of pancreatic carcinoma cells by epigenetically silencing CDKN1A and KLF2. Cancer Commun (Lond). 2018;38:64. doi: 10.1186/s40880-018-0333-9
- Chen Z, Chen X, Chen P, et al. Long noncoding RNA SNHG20 promotes non-small cell lung cancer cell proliferation and migration by epigenetically silencing of P21 expression. Cell Death Dis. 2017;8:e3092. doi: 10.1038/cddis.2017.484
- Statello L, Guo CJ, Chen LL, Huarte M. Gene regulation by long noncoding RNAs and its biological functions. Nat Rev Mol Cell Biol. 2021;22:96-118. doi: 10.1038/s41580-020-00315-9
- Cai Z, Cao C, Ji L, et al. RIC-seq for global in situ profiling of RNA-RNA spatial interactions. Nature. 2020;582(7812):432-437. doi: 10.1038/s41586-020-2249-1
- Huang J, Zhou N, Watabe K, et al. Long noncoding RNA UCA1 promotes breast tumor growth by suppressing p27 (Kip1). Cell Death Dis. 2014;5:e1008. doi: 10.1038/cddis.2013.541
- Luo J, Xiang H. LncRNA MYLK-AS1 acts as an oncogene by epigenetically silencing large tumor suppressor 2 (LATS2) in gastric cancer. Bioengineered. 2021;12:3101-3112. doi: 10.1080/21655979.2021.1944019
- Ding J, Xie M, Lian Y, et al. Long noncoding RNA HOXA-AS2 represses P21 and KLF2 expression transcription by binding with EZH2 and LSD1 in colorectal cancer. Oncogenesis. 2017;6:e288. doi: 10.1038/oncsis.2016.84
- Zhang G, Xu Y, Wang S, et al. LncRNA SNHG17 promotes gastric cancer progression by epigenetically silencing of p15 and p57. J Cell Physiol. 2019;234(4):5163-5174. doi: 10.1002/jcp.27320
- Rom A, Melamed L, Gil N, et al. Regulation of CHD2 expression by the Chaserr long noncoding RNA gene is essential for cell viability. Nat Commun. 2019;10:5092. doi: 10.1038/s41467-019-13075-8
- Jiang X, Li Q, Zhang S, Song C, Zheng P. Long noncoding RNA GIHCG induces cancer progression and chemoresistance and indicates poor prognosis in colorectal cancer. Onco Targets Ther. 2019;12:1059-1070. doi: 10.2147/OTT.S192290
- Li L, Chen J, Wang A, Yi K. ALKBH5 regulates ovarian cancer growth via demethylating long noncoding RNA PVT1 in ovarian cancer. J Cell Mol Med. 2023;28(2):e18066. doi: 10.1111/jcmm.18066
- Ma P, Pan Y, Yang F, et al. KLF5-Modulated lncRNA NEAT1 contributes to tumorigenesis by acting as a scaffold for BRG1 to silence GADD45A in gastric cancer. Mol Ther Nucleic Acids. 2020;22:382-395. doi: 10.1016/j.omtn.2020.09.003
- Abildgaard C, do Canto LM, Rainho CA, et al. The long non-coding RNA SNHG12 as a mediator of carboplatin resistance in ovarian cancer via epigenetic mechanisms. Cancers (Basel). 2022;14(7):1664. doi: 10.3390/cancers14071664
- Huang X, Chen J, Li H, et al. LncRNA SNHG12 suppresses adipocyte inflammation and insulin resistance by regulating the HDAC9/Nrf2 axis. FASEB J. 2024;38(13):e23794. doi: 10.1096/fj.202400236RR
- Zhang KJ, Tan XL, Guo L. The long noncoding RNA DANCR regulates the inflammatory phenotype of breast cancer cells and promotes breast cancer progression via the EZH2-dependent suppression of SOCS3 transcription. Mol Oncol. 2020;14(2):309-328. doi: 10.1002/1878-0261.12622
- Xie F, Huang Q, Wang C, et al. Downregulation of the long noncoding RNA SNHG14 suppresses cell proliferation and invasion by regulating EZH2 in pancreatic ductal adenocarcinoma (PDAC). Cancer Biomark. 2020;27(3):357-364. doi: 10.3233/CBM-190908
- Cable J, Heard E, Hirose T, et al. Noncoding RNAs: Biology and applications: A Keystone symposia report. Ann N Y Acad Sci. 2021;1506:118-141. doi: 10.1111/nyas.14713
- Liu YW, Xia R, Lu K, et al. LincRNA FEZF1-AS1 represses p21 expression to promote gastric cancer proliferation through LSD1-Mediated H3K4me2 demethylation. Mol Cancer. 2017;16(1):39. doi: 10.1186/s12943-017-0588-9
- Lian Y, Yan C, Lian Y, et al. Long intergenic non-protein-coding RNA 01446 facilitates the proliferation and metastasis of gastric cancer cells by interacting with the histone lysine-specific demethylase LSD1. Cell Death Dis. 2020;11(7):522. doi: 10.1038/s41419-020-2729-0
- Mao X, Ji T, Liu A, Weng Y. ELK4-mediated lncRNA SNHG22 promotes gastric cancer progression through interacting with EZH2 and regulating miR-200c-3p/Notch1 axis. Cell Death Dis. 2021;12(11):957. doi: 10.1038/s41419-021-04228-z
- Sarma K, Cifuentes-Rojas C, Ergun A, et al. ATRX directs binding of PRC2 to Xist RNA and polycomb targets. Cell. 2014;159:869-883. Erratum in Cell. 2014;159:1228. Erratum in Cell. 2015;160:568-569. doi: 10.1016/j.cell.2014.10.019
- McHugh CA, Chen CK, Chow A, et al. The Xist lncRNA interacts directly with SHARP to silence transcription through HDAC3. Nature. 2015;521:232-236. doi: 10.1038/nature14443
- Lu Y, Liu X, Xie M, et al. The NF-κB-responsive long noncoding RNA FIRRE regulates the posttranscriptional regulation of inflammatory gene expression by interacting with hnRNPU. J Immunol. 2017;199(10):3571-3582. doi: 10.4049/jimmunol.1700091
- Wang B, Xu W, Hu C, et al. Critical roles of the lncRNA CASC11 in tumor progression and cancer metastasis: The biomarker and therapeutic target potential. Genes Dis. 2020;9(2):325-333. doi: 10.1016/j.gendis.2020.11.016
- Mou K, Zhang X, Mu X, et al. LNMAT1 promotes invasion-metastasis cascade in malignant melanoma by epigenetically suppressing CADM1 expression. Front Oncol. 2019;9:569. doi: 10.3389/fonc.2019.00569
- Wozniak M, Czyz M. lncRNAs-EZH2 interaction as promising therapeutic target in cutaneous melanoma. Front Mol Biosci. 2023;10:1170026. doi: 10.3389/fmolb.2023.1170026
- Zhang F, Ruan X, Ma J, et al. DGCR8/ZFAT-AS1 promotes CDX2 transcription in a PRC2 complex-dependent manner to facilitate the malignant biological behavior of glioma cells. Mol Ther. 2020;28(2):613-630. doi: 10.1016/j.ymthe.2019.11.015
- Chang KC, Diermeier SD, Yu AT, et al. MaTAR25 lncRNA regulates the Tensin1 gene to impact breast cancer progression. Nat Commun. 2020;11(1):6438. doi: 10.1038/s41467-020-20207-y
- Somasundaram S, Forrest ME, Moinova H, et al. DNMT1- associated lincRNA DACOR1 reprograms genome-wide DNA methylation in colon cancer. Clin Epigenet. 2018;10:127. doi: 10.1186/s13148-018-0555-3
- Wang Q, Mao X, Luo F, Wang J. LINC00511 promotes gastric cancer progression by regulating SOX4 and epigenetically repressing PTEN to activate PI3K/AKT pathway. J Cell Mol Med. 2021;25:9112-9127. doi: 10.1111/jcmm.16656
- Dai Z, Liu X, Zeng H, Chen Y. Long noncoding RNA HOTAIR facilitates pulmonary vascular endothelial cell apoptosis via DNMT1-mediated hypermethylation of the Bcl-2 promoter in COPD. Respir Res. 2022;23(1):356. doi: 10.1186/s12931-022-02234-z
- Lu GH, Zhao HM, Liu ZY, Cao Q, Shao RD, Sun G. LncRNA SAMD12-AS1 promotes the progression of gastric cancer via DNMT1/p53 axis. Arch Med Res. 2021;52(7):683-691. doi: 10.1016/j.arcmed.2021.04.004
- Yu Y, Lu X, Yan Y, et al. lncRNA KIF9-AS1 accelerates hepatocellular carcinoma growth by recruiting DNMT1 to promote RAI2 DNA methylation. J Oncol. 2022;2022:3888798. doi: 10.1155/2022/3888798
- Hao A, Wang Y, Stovall DB, Wang Y, Sui G. Emerging roles of LncRNAs in the EZH2-regulated oncogenic network. Int J Biol Sci. 2021;17(13):3268-3280. doi: 10.7150/ijbs.63488
- Wang X, Wang Y, Li L, et al. lncRNA coordinates with Ezh2 to inhibit HIF-1α transcription and suppress cancer cell adaptation to hypoxia. Oncogene. 2020;39(9):1860-1874. doi: 10.1038/s41388-019-1123-9
- Aguilo F, Di Cecilia S, Walsh MJ. Long non-coding RNA ANRIL and polycomb in human cancers and cardiovascular disease. Curr Top Microbiol Immunol. 2016;394:29-39. doi: 10.1007/82_2015_455
- Sun TT, He J, Liang Q, et al. LncRNA GClnc1 promotes gastric carcinoma and may act as a modular scaffold of WDR5 and KAT2A complexes to specify the histone modification pattern. Cancer Discov. 2016;6(7):784-801. doi: 10.1158/2159-8290.CD-15-0921
- Ma M, Zhang Y, Weng M, et al. lncRNA GCAWKR promotes gastric cancer development by scaffolding the chromatin modification factors WDR5 and KAT2A. Mol Ther. 2018;26(11):2658-2668. doi: 10.1016/j.ymthe.2018.09.002
- Dimitrova N, Zamudio JR, Jong RM, et al. LincRNA-p21 activates p21 in cis to promote Polycomb target gene expression and to enforce the G1/S checkpoint. Mol Cell. 2014;54(5):777-790. doi: 10.1016/j.molcel.2014.04.025
- Luo M, Xie L, Su Y, et al. TM4SF19-AS1 facilitates the proliferation of lung squamous cell carcinoma by recruiting WDR5 to mediate TM4SF19. Mol Cell Probes. 2022;65:101849. doi: 10.1016/j.mcp.2022.101849
- Arab K, Park YJ, Lindroth AM, et al. Long noncoding RNA TARID directs the demethylation and activation of the tumor suppressor TCF21 via GADD45A. Mol Cell. 2014;55:604-614. doi: 10.1016/j.molcel.2014.06.031
- Ghafouri-Fard S, Dashti S, Taheri M. The HOTTIP (HOXA transcript at the distal tip) lncRNA: Review of oncogenic roles in humans. Biomed Pharmacother. 2020;127:110158. doi: 10.1016/j.biopha.2020.110158
- Xiang JF, Yin QF, Chen T, et al. Human CRC-specific CCAT1-L lncRNA regulates long-range chromatin interactions at the MYC locus. Cell Res. 2014;24(5):513-531. doi: 10.1038/cr.2014.35
- Hakami MA, Hazazi A, Khan FR, et al. PVT1 lncRNA in lung cancer: A key player in tumorigenesis and therapeutic opportunities. Pathol Res Pract. 2024;253:155019. doi: 10.1016/j.prp.2023.155019
- Li W, Notani D, Ma Q, et al. Functional roles of enhancer RNAs for estrogen-dependent transcriptional activation. Nature. 2013;498(7455):516-520. doi: 10.3390/ijms21103711
- Ferrer J, Dimitrova N. Transcription regulation by long noncoding RNAs: Mechanisms and disease relevance. Nat Rev Mol Cell Biol. 2024;25(5):396-415. doi: 10.1038/s41580-023-00694-9
- Beucher A, Miguel-Escalada I, Balboa D, et al. The HASTER lncRNA promoter is a cis-acting transcriptional stabilizer of HNF1A. Nat Cell Biol. 2022;24(10):1528-1540. doi: 10.1038/s41556-022-00996-8
- Jain AK, Xi Y, McCarthy R, et al. LncPRESS1 is a p53- regulated LncRNA that safeguards pluripotency by disrupting SIRT6-mediated de-acetylation of histone H3K56. Mol Cell. 2016; 64:967-981. doi: 10.1016/j.molcel.2016.10.039
- An H, Elvers KT, Gillespie JA, et al. A toolkit for the identification of the NEAT1_2/paraspeckle modulators. Nucleic Acids Res. 2022;50(20):e119. doi: 10.1093/nar/gkac771
- Li Z, Chao TC, Chang KY, et al. The long noncoding RNA THRIL regulates TNFα expression through its interaction with hnRNPL. Proc Natl Acad Sci U S A. 2014;111(3):1002-1007. doi: 10.1073/pnas.1313768111
- Li Y, Wang Z, Shi H, et al. HBXIP and LSD1 Scaffolded by lncRNA hotair mediate transcriptional activation by c-Myc. Cancer Res. 2016;7:293-304. doi: 10.1158/0008-5472.CAN-14-3607
- Gomez JA, Wapinski OL, Yang YW, et al. The NeST long ncRNA controls microbial susceptibility and epigenetic activation of the interferon-γ locus. Cell. 2013;152(4):743-754. doi: 10.1016/j.cell.2013.01.015
- Swaminathan G, Rogel-Ayala DG, Armich A, Barreto G. Implications in cancer of nuclear micro RNAs, long non-coding RNAs, and circular RNAs bound by PRC2 and FUS. Cancers (Basel). 2024;16(5):868. doi: 10.3390/cancers16050868
- Lei P, Guo Q, Hao J, et al. Exploring the evolving roles and clinical significance of circRNAs in head and neck squamous cell carcinomas. J Cancer. 2024;15:3984-3994. doi: 10.7150/jca.96614
- Chen R, Liu Y, Zhuang H, et al. Quantitative proteomics revealed that the long noncoding RNA MALAT1 interacts with DBC1 to regulate p53 acetylation. Nucleic Acids Res. 2017;45(17):9947-9959. doi: 10.1093/nar/gkx600
- Yamazaki T, Souquere S, Chujo T, et al. Functional domains of NEAT1 architectural lncRNA induce paraspeckle assembly through phase separation. Mol Cell. 2018;70(6):1038-1053.e7. doi: 10.1016/j.molcel.2018.05.019
- Nakagawa S, Yamazaki T, Hirose T. Molecular dissection of nuclear paraspeckles: Toward understanding the emerging world of the RNP milieu. Open Biol. 2018;8:180150. doi: 10.1098/rsob.180150
- Hirose T, Yamazaki T, Nakagawa S. Molecular anatomy of the architectural NEAT1 noncoding RNA: The domains, interactors, and biogenesis pathway required to build phase-separated nuclear paraspeckles. Wiley Interdiscipl Rev RNA. 2019;10(6):e1545. doi: 10.1002/wrna.1545
- Fox AH, Nakagawa S, Hirose T, Bond CS. Paraspeckles: Where long noncoding RNA meets phase separation. Trends Biochem Sci. 2018;43(2):124-135. doi: 10.1016/j.tibs.2017.12.001
- Todorovski V, McCluggage F, Li Y, et al. Confined environments induce polarized paraspeckle condensates. Commun Biol. 2023;6(1):145. doi: 10.1038/s42003-023-04528-4
- Mello SS, Sinow C, Raj N, et al. Neat1 is a p53-inducible lincRNA essential for transformation suppression. Genes Dev. 2017;31(11):1095-1108. doi: 10.1101/gad.284661.116
- Taiana E, Favasuli V, Ronchetti D, et al. Long noncoding RNA NEAT1 targeting impairs the DNA repair machinery and triggers antitumor activity in multiple myeloma. Leukemia. 2020;34:234-244. doi: 10.1038/s41375-019-0542-5
- Smith NE, Spencer-Merris P, Fox AH, Petersen J, Michael MZ. The long and the short of it: NEAT1 and cancer cell metabolism. Cancers (Basel). 2022;14(18):4388. doi: 10.3390/cancers14184388
- Kessler SM, Hosseini K, Hussein UK, et al. Hepatocellular carcinoma and nuclear paraspeckles: Induction of chemoresistance and prediction for poor survival. Cell Physiol Biochem. 2019;52(4):787-801. doi: 10.33594/000000055
- Takakuwa H, Yamazaki T, Souquere S, et al. The shell protein composition specified by the lncRNA NEAT1 domains dictates the formation of paraspeckles as distinct membraneless organelles. Nat Cell Biol. 2023;25(11):1664-1675. doi: 10.1038/s41556-023-01254-1
- Long F, Li X, Pan J, et al. The role of lncRNA NEAT1 in human cancer chemoresistance. Cancer Cell Int. 2024;24(1):236. doi: 10.1186/s12935-024-03426-x
- Jia X, Wei L, Zhang Z. NEAT1 overexpression indicates a poor prognosis and induces chemotherapy resistance via the miR-491-5p/SOX3 signaling pathway in ovarian cancer. Front Genet. 2021;12:616220. doi: 10.3389/fgene.2021.616220
- Ma F, Lei YY, Ding MG, Luo LH, Xie YC, Liu XL. LncRNA NEAT1 interacted with DNMT1 to regulate malignant phenotype of cancer cell and cytotoxic T cell infiltration via epigenetic inhibition of p53, cGAS, and STING in lung cancer. Front Genet. 2020;11:250. doi: 10.3389/fgene.2020.00250
- Mamontova V, Trifault B, Gribling-Burrer AS, et al. NEAT1 promotes genome stability via m6A methylation-dependent regulation of CHD4. Genes Dev. 2024; 38(17-20):915-930. doi: 10.1101/gad.351913.124
- Shin VY, Chen J, Cheuk IWY, et al. Long noncoding RNA NEAT1 confers an oncogenic role in triple-negative breast cancer by modulating chemoresistance and cancer stemness. Cell Death Dis. 2019;10(4):270. doi: 10.1038/s41419-019-1513-5
- Dong P, Xiong Y, Yue J, et al. Long non-coding RNA NEAT1: A novel target for diagnosis and therapy in human tumors. Front Genet. 2018;9:471. doi: 10.3389/fgene.2018.00471
- Peng X, Zhang K, Ma L, Xu J, Chang W. The role of long non-coding RNAs in thyroid cancer. Front Oncol. 2020;10:941. doi: 10.3389/fonc.2020.00941
- Sun W, Qin Y, Wang Z, et al. The NEAT1_2/miR-491 axis modulates papillary thyroid cancer invasion and metastasis through TGM2/NFκb/FN1 signaling. Front Oncol. 2021;11:610547. doi: 10.3389/fonc.2021.610547
- Sun W, Lan X, Zhang H, et al. NEAT1_2 functions as a competing endogenous RNA to regulate ATAD2 expression by sponging microRNA-106b-5p in papillary thyroid cancer. Cell Death Dis. 2018;9(3):1-15. doi: 10.1038/s41419-018-0418-z
- Schell B, Legrand P, Fribourg S. Crystal structure of SFPQ-NONO heterodimer. Biochimie. 2022;198:1-7. doi: 10.1016/j.biochi.2022.02.011
- Zhang S, Cooper JA, Chong YS, et al. NONO enhances mRNA processing of super-enhancer-associated GATA2 and HAND2 genes in neuroblastoma. EMBO Rep. 2023;24(2):e54977. doi: 10.15252/embr.202254977
- Engreitz JM, Sirokman K, McDonel P, et al. RNA-RNA interactions enable specific targeting of noncoding RNAs to nascent Pre-mRNAs and chromatin sites. Cell. 2014;159(1):188-199. doi: 10.1016/j.cell.2014.08.018
- Lee PW, Marshall AC, Knott GJ, et al. Paraspeckle subnuclear bodies depend on the dynamic heterodimerisation of DBHS RNA-binding proteins via their structured domains. J Biol Chem. 2022;298(11):102563. doi: 10.1016/j.jbc.2022.102563
- Jen HW, Gu DL, Lang YD, Jou YS. PSPC1 potentiates IGF1R expression to augment cell adhesion and motility. Cells. 2020;9(6):1490. doi: 10.3390/cells9061490
- Yang L, Yang J, Jacobson B, et al. SFPQ promotes lung cancer malignancy via regulation of CD44 v6 expression. Front Oncol. 2022;12:862250. doi: 10.3389/fonc.2022.862250
- Lin Y, Zhong W, Lin Q, et al. SFPQ promotes the proliferation, migration and invasion of hepatocellular carcinoma cells and is associated with poor prognosis. Am J Cancer Res. 2023;13(6):2269-2284.
- Xiao M, Wang F, Chen N, et al. Smad4 sequestered in SFPQ condensates prevents TGF-β tumor-suppressive signaling. Dev Cell. 2024;59(1):48-63.e8. doi: 10.1016/j.devcel.2023.11.020
- Ji Q, Zhang L, Liu X, et al. Long noncoding RNA MALAT1 promotes tumor growth and metastasis in colorectal cancer by binding to SFPQ and releasing the oncogene PTBP2 from the SFPQ/PTBP2 complex. Br J Cancer. 2014;111:736-748. doi: 10.1038/bjc.2014.383
- Klotz-Noack K, Klinger B, Rivera M, et al. SFPQ depletion is synthetically lethal with BRAFV600E in colorectal cancer cells. Cell Rep. 2020;32(12):108184. doi: 10.1016/j.celrep.2020.108184
- Zhang XL, Chen XH, Xu B, et al. K235 acetylation couples with PSPC1 to regulate the m6A demethylation activity of ALKBH5 and tumorigenesis. Nat Commun. 2023;14(1):3815. doi: 10.1038/s41467-023-39414-4
- Lemster AL, Weingart A, Bottner J, et al. Elevated PSPC1 and KDM5C expression indicates poor prognosis in prostate cancer. Hum Pathol. 2023;138:1-11. doi: 10.1016/j.humpath.2023.05.007
- Tyzack GE, Manferrari G, Newcombe J, Luscombe NM, Luisier R, Patani R. Paraspeckle components NONO and PSPC1 are not mislocalized from motor neuron nuclei in sporadic ALS. Brain. 2020;143(8):e66. doi: 10.1093/brain/awaa205
- Harley J, Hagemann C, ASerio A, Patani R. TDP-43 and FUS mislocalization in VCP mutant motor neurons is reversed by pharmacological inhibition of the VCP D2 ATPase domain. Brain Commun. 2021;3:fcab166. doi: 10.1093/braincomms/fcab166
- Shao W, Bi X, Pan Y, et al. Phase separation of RNA-binding protein promotes polymerase binding and transcription. Nat Chem Biol. 2022;18(1):70-80. doi: 10.1038/s41589-021-00904-5
- Yeh HW, Hsu EC, Lee SS, et al. PSPC1 mediates TGF- β1 autocrine signaling and Smad2/3 target switching to promote EMT, stemness and metastasis. Nat Cell Biol. 2018;20(4):479-491. doi: 10.1038/s41556-018-0062-y.
- Li J, Cui P, Sun Q, et al. PSPC1 regulates CHK1 phosphorylation through phase separation and participates in mouse oocyte maturation. Acta Biochim Biophys Sin (Shanghai). 2021;53(11):1527-1537. doi: 10.1093/abbs/gmab123
- Zhan T, Cheng X, Zhu Q, et al. LncRNA LOC105369504 inhibits tumor proliferation and metastasis in colorectal cancer by regulating PSPC1. Cell Death Discov. 2023;9(1):89. doi: 10.1038/s41420-023-01384-3
- Mohankumar K, Shrestha R, Safe S. Nuclear receptor 4A1 (NR4A1) antagonists target paraspeckle component 1 (PSPC1) in cancer cells. Mol Carcinog. 2022;61(1):73-84. doi: 10.1002/mc.23362
- Zhang H, Su X, Burley SK, Zheng XFS. mTOR regulates aerobic glycolysis through NEAT1 and nuclear paraspeckle-mediated mechanisms in hepatocellular carcinoma. Theranostics. 2022;12:3518-3533. doi: 10.7150/thno.72581
- Hussein MA, Valinezhad K, Adel E, Munirathinam G. MALAT-1 is a key regulator of epithelial-mesenchymal transition in cancer: A potential therapeutic target for metastasis. Cancers (Basel). 2024;16(1):234. doi: 10.3390/cancers16010234
- Nakagawa S, Ip JY, Shioi G, et al. Malat1 is not an essential component of nuclear speckles in mice. RNA. 2012;18:1487-1499. doi: 10.1261/rna.033217.112
- Arratia F, Fierro C, Blanco A, et al. Selective concurrence of the long non-coding RNA MALAT1 and the polycomb repressive complex 2 to the promoter regions of active genes in MCF7 breast cancer cells. Curr Issues Mol Biol. 2023;45(6):4735-4748. doi: 10.3390/cimb45060301
- Frappier L. Viral disruption of promyelocytic leukemia (PML) nuclear bodies by hijacking host PML regulators. Virulence. 2011;2(1):58-62. doi: 10.4161/viru.2.1.14610
- Huang WH, Su WM, Wang CW, et al. The Momordica anti-HIV protein MAP30 abrogates the Epstein-Barr virus nuclear antigen 1-dependent functions in host cells. Heliyon. 2023;9(11):e21486. doi: 10.1016/j.heliyon.2023.e21486
- D’Amico F, Mukhopadhyay R, Ovaa H, Mulder MPC. Targeting TRIM proteins: A quest toward drugging an emerging protein class. Chembiochem. 2021;22(12):2011-2031. doi: 10.1002/cbic.202000787
- Dodel M, Guiducci G, Dermit M, et al. TREX reveals proteins that bind to specific RNA regions in living cells. Nat Methods. 2024;21(3):423-434. doi: 10.1038/s41592-024-02181-1
- Sullivan KD, Galbraith MD, Andrysik Z, Espinosa JM. Mechanisms of transcriptional regulation by p53. Cell Death Differ. 2018;25(1):133-143. doi: 10.1038/cdd.2017.174
- Wang S, Zhao Y, Aguilar A, Bernard D, Yang CY. Targeting the MDM2-p53 protein-protein interaction for new cancer therapy: Progress and challenges. Cold Spring Harb Perspect Med. 2017;7(5):a026245. doi: 10.1101/cshperspect.a026245
- Stubbs FE, Flynn BP, Rivers CA, et al. Identification of a novel GR-ARID1a-P53BP1 protein complex involved in DNA damage repair and cell cycle regulation. Oncogene. 2022;41(50):5347-5360. doi: 10.1038/s41388-022-02516-2
- Kozłowska J, Kolenda T, Poter P, et al. Long intergenic non-coding RNAs in HNSCC: From “Junk DNA” to important prognostic factor. Cancers (Basel). 2021;13(12):2949. doi: 10.3390/cancers13122949
- Lin T, Hou PF, Meng S, et al. Emerging roles of p53-related lncRNAs in cancer progression: A systematic review. Int J Biol Sci. 2019;15(6):1287-1298. doi: 10.7150/ijbs.33218
- Adriaens C, Standaert L, Barra J, et al. p53 induces formation of NEAT1 lncRNA-containing paraspeckles that modulate replication stress response and chemosensitivity. Nat Med. 2016;22(8):861-868. doi: 10.1038/nm.4135
- Cheng Y, Huang N, Yin Q, et al. LncRNA TP53TG1 plays an anti-oncogenic role in cervical cancer by synthetically regulating transcriptome profile in HeLa cells. Front Genet. 2022;13:981030. doi: 10.3389/fgene.2022.981030
- Shao M, Ma H, Wan X, Liu Y. Survival analysis for long noncoding RNAs identified TP53TG1 as an antioncogenic target for breast cancer. J Cell Physiol. 2020;235:6574-6581. doi: 10.1002/jcp.29517
- Liao D, Liu X, Yuan X, et al. Long non-coding RNA tumor protein 53 target gene 1 promotes cervical cancer development via regulating microRNA-33a-5p to target forkhead box K2. Cell Cycle. 2022;21(6):572-584. doi: 10.1080/15384101.2022.2026705
- Zhao K, Wang X, Xue X, Li L, Hu Y. A long noncoding RNA sensitizes genotoxic treatment by attenuating ATM activation and homologous recombination repair in cancers. PLoS Biol. 2020;18:e3000666. doi: 10.1371/journal.pbio.3000666
- Unfried JP, Marín-Baquero M, Rivera-Calzada Á, et al. Long noncoding RNA NIHCOLE promotes the ligation efficiency of DNA double-strand breaks in hepatocellular carcinoma. Cancer Res. 2021;81(19):4910-4925. doi: 10.1158/0008-5472.CAN-21-0463
- Sharma V, Khurana S, Kubben N, et al. A BRCA1-interacting lncRNA regulates homologous recombination. EMBO Rep. 2015;16:1520-1534. doi: 10.15252/embr.201540437
- Yadav A, Biswas T, Praveen A, et al. Targeting MALAT1 augments sensitivity to PARP inhibition by impairing homologous recombination in prostate cancer. Cancer Res Commun. 2023;3:2044-2061. doi: 10.1158/2767-9764.CRC-23-0089
- Hu Y, Lin J, Fang H, et al. Targeting the MALAT1/PARP1/ LIG3 complex induces DNA damage and apoptosis in multiple myeloma. Leukemia. 2018;32:2250-2262. doi: 10.1038/s41375-018-0104-2
- Zhao J, Xu J, Wu M, et al. LncRNA H19 regulates breast cancer DNA damage response and sensitivity to PARP inhibitors via binding to ILF2. Int J Mol Sci. 2023;24:9157. doi: 10.3390/ijms24119157
- Wang Y, Nie H, He X, et al. The emerging role of super enhancer--derived noncoding RNAs in human cancer. Theranostics. 2020;10(24):11049. doi: 10.7150/thno.49168
- Saldaña-Meyer R, Rodriguez-Hernaez J, Escobar T, et al. RNA interactions are essential for CTCF-mediated genome organization. Mol Cell. 2019;76(3):412-422.e5. doi: 10.1016/j.molcel.2019.08.015
- Diot C, Richard CA, Risso-Ballester J, et al. Hardening of respiratory syncytial virus inclusion bodies by cyclopamine proceeds through perturbation of the interactions of the M2-1 protein with RNA and the P protein. Int J Mol Sci. 2023;24(18):13862. doi: 10.3390/ijms241813862
- Etibor TA, Vale-Costa S, Sridharan S, et al. Defining basic rules for hardening influenza A virus liquid condensates. Elife. 2023;12:e85182. doi: 10.7554/eLife.85182
- Liboy-Lugo JM, Espinoza CA, Sheu-Gruttadauria J, et al. Protein-protein interactions with G3BPs drive stress granule condensation and gene expression changes under cellular stress. bioRxiv [Preprint]. 2024. doi: 10.1101/2024.02.06.579149
- Alam U, Kennedy D. Rasputin a decade on and more promiscuous than ever? A review of G3BPs. Biochim Biophys Acta Mol Cell Res. 2019;1866(3):360-370. doi: 10.1016/j.bbamcr.2018.09.001
- Shih JW, Wang WT, Tsai TY, Kuo CY, Li HK, Wu Lee YH. Critical roles of the RNA helicase DDX3 and its interactions with eIF4E/PABP1 in stress granule assembly and stress response. Biochem J. 2012;441(1):119-129. doi: 10.1042/BJ20110739
- Aditi, Folkmann AW, Wente SR. Cytoplasmic hGle1A regulates stress granules by modulation of translation. Mol Biol Cell. 2015;26(8):1476-1490. doi: 10.1091/mbc.E14-11-1523
- Zhan Y, Chen X, Zheng H, et al. YB1 associates with oncogenetic roles and poor prognosis in nasopharyngeal carcinoma. Sci Rep. 2022;12(1):3699. doi: 10.1038/s41598-022-07636-z
- Cui BC, Sikirzhytski V, Aksenova M, et al. Pharmacological inhibition of DEAD-Box RNA Helicase 3 attenuates stress granule assembly. Biochem Pharmacol. 2020;182:114280. doi: 10.1016/j.bcp.2020.114280
- Kitajima H, Maruyama R, Niinuma T, et al. TM4SF1-AS1 inhibits apoptosis by promoting stress granule formation in cancer cells. Cell Death Dis. 2023;14(7):424. doi: 10.1038/s41419-023-05953-3
- Kang W, Wang Y, Yang W, Zhang J, Zheng H, Li D. Research progress on the structure and function of G3BP. Front Immunol. 2021;12:718548. doi: 10.3389/fimmu.2021.718548
- Freibaum BD, Messing J, Nakamura H, et al. Identification of small molecule inhibitors of G3BP-driven stress granule formation. J Cell Biol. 2024;223(3):e202308083. doi: 10.1083/jcb.202308083
- Mukhopadhyay C, Zhou P. Role(s) of G3BPs in human pathogenesis. J Pharmacol Exp Ther. 2023;387(1):100-110. doi: 10.1124/jpet.122.001538
- Gal J, Chen J, Na DY, Tichacek L, Barnett KR, Zhu H. Acetylation of lysine-376 of G3BP1 regulates RNA binding and stress granule dynamics. Mol Cell Biol. 2019;39(22):e00052-19. doi: 10.1128/MCB.00052-19
- Lee AK, Klein J, Fon Tacer K, et al. Translational repression of G3BP in cancer and germ cells suppresses stress granules and enhances stress tolerance. Mol Cell. 2020;79(4):645-659.e9. doi: 10.1016/j.molcel.2020.06.037
- Li M, Fan X, Zhao J, Wang D. Establishment and validation of a four-stress granule-related gene signature in hepatocellular carcinoma. J Clin Transl Hepatol. 2024;12(1):1-14. doi: 10.14218/JCTH.2023.00019
- Chen S, Cao X, Zhang J, Wu W, Zhang B, Zhao F. circVAMP3 drives CAPRIN1 phase separation and inhibits hepatocellular carcinoma by suppressing c-Myc translation. Adv Sci (Weinh). 2022;9:e2103817. doi: 10.1002/advs.202103817
- Zhang M, Peng S. The association and clinical relevance of the phase-separating protein CAPRIN1 with noncoding RNA. Cell Stress Chaperones. 2023;28(2):125-132. doi: 10.1007/s12192-023-01320-5
- Schneider C, Schneider G. Stress granules-membraneless organelles as therapeutic targets in pancreatic cancer. EMBO Mol Med. 2024;16(3):429-431. doi: 10.1038/s44321-024-00040-2
- Kim A, Shim S, Kim YH, Kim MJ, Park S, Myung JK. Inhibition of Y-Box binding protein 1 suppresses cell growth and motility in colorectal cancer. Mol Cancer Ther. 2020;19(2):479-489. doi: 10.1158/1535-7163.MCT-19-0265
- Wang F, Li J, Fan S, et al. Targeting stress granules: A novel therapeutic strategy for human diseases. Pharmacol Res. 2020;161:105143. doi: 10.1016/j.phrs.2020.105143
- An S, Huang W, Huang X, et al. Integrative network analysis identifies cell-specific trans regulators of m6A. Nucleic Acids Res. 2020;48:1715-1729. doi: 10.1093/nar/gkz1206
- Zhang Y, Jing Y, Wang Y, et al. NAT10 promotes gastric cancer metastasis via N4-acetylated COL5A1. Signal Transduct Target Ther. 2021;6:173. doi: 10.1038/s41392-021-00489-4
- Han J, Liu Q, Zhou Y, Li D, Wang R. Landscape of internal N7-methylguanosine of long noncoding RNA modifications in resistant acute myeloid leukemia. BMC Genomics. 2023;24:425. doi: 10.1186/s12864-023-09526-8
- Kong Z, Lu Y, Yang Y, et al. m6A-Mediated biogenesis of circDDIT4 inhibits prostate cancer progression by sequestrating ELAVL1/HuR. Mol Cancer Res. 2023;21:1342-1355. doi: 10.1158/1541-7786.MCR-22-0271
- Yu XM, Li SJ, Yao ZT, et al. N4-acetylcytidine modification of lncRNA CTC-490G23.2 promotes cancer metastasis through interacting with PTBP1 to increase CD44 alternative splicing. Oncogene. 2023;42:1101-1116. doi: 10.1038/s41388-023-02628-3
- Dou X, Huang L, Xiao Y, et al. METTL14 is a chromatin regulator independent of its RNA N6-methyladenosine methyltransferase activity. Protein Cell. 2023;14(9):683-697. doi: 10.1093/procel/pwad009
- Hu J, Lin H, Wang C, Su Q, Cao B. METTL14-mediated RNA methylation in digestive system tumors. Int J Mol Med. 2023;52(3):86. doi: 10.3892/ijmm.2023.5289
- Wang J, Wang S, Yang H, et al. Methyltransferase like- 14 suppresses growth and metastasis of non-small-cell lung cancer by decreasing LINC02747. Cancer Sci. 2024;115(9):2931-2946. doi: 10.1111/cas.16254
- Lee JH, Wang R, Xiong F, et al. Enhancer RNA m6A methylation facilitates transcriptional condensate formation and gene activation. Mol Cell. 2021;81(16):3368-3385.e9. doi: 10.1016/j.molcel.2021.07.024
- Long X, Wen F, Li J, Huang X. LncRNA FEZF1-AS1 accelerates multiple myeloma progression by regulating IGF2BP1/ BZW2 signaling. Hematol Oncol. 2023;41(4):694-703. doi: 10.1002/hon.3157
- Shen W, Zhu M, Wang Q, et al. DARS-AS1 recruits METTL3/METTL14 to bind and enhance DARS mRNA m(6)A modification and translation for cytoprotective autophagy in cervical cancer. RNA Biol. 2022;19:751-763. doi: 10.1080/15476286.2022.2079889
- Liu H, Xu Y, Yao B, Sui T, Lai L, Li Z. A novel N6-methyladenosine (m6A)-dependent fate decision for the lncRNA THOR. Cell Death Dis. 2020;11:613. doi: 10.1038/s41419-020-02833-y
- Ban Y, Tan P, Cai J, et al. LNCAROD is stabilized by m6A methylation and promotes cancer progression via forming a ternary complex with HSPA1A and YBX1 in head and neck squamous cell carcinoma. Mol Oncol. 2020;14:1282-1296. doi: 10.1002/1878-0261.12676
- Ni W, Yao S, Zhou Y, et al. Long noncoding RNA GAS5 inhibits the progression of colorectal cancer by interacting with and triggering YAP phosphorylation and degradation and is negatively regulated by the m6A reader YTHDF3. Mol Cancer. 2019;18:143. doi: 10.1186/s12943-019-1079-y
- Chen F, Li M, Wang L. LncRNA CASC11 promotes hepatocellular carcinoma progression via upregulation of UBE2T in a m6A-dependent manner. Front Oncol. 2021;11:772671. doi: 10.3389/fonc.2021.772671
- Zhang J, Guo S, Piao HY, et al. ALKBH5 promotes invasion and metastasis of gastric cancer by decreasing methylation of the lncRNA NEAT1. J Physiol Biochem. 2019;75:379-389. doi: 10.1007/s13105-019-00690-8
- Liu T, Wang H, Fu Z, et al. Methyltransferase-like 14 suppresses growth and metastasis of renal cell carcinoma by decreasing long noncoding RNA NEAT1. Cancer Sci. 2022;113:446-458. doi: 10.1111/cas.15212
- Wei H, Huang L, Lu Q, et al. N6-methyladenosine-modified LEAWBIH drives hepatocellular carcinoma progression through epigenetically activating Wnt/β-catenin signaling. J Hepatocellular Carcinoma. 2023;10:1991-2007. doi: 10.2147/JHC.S433070
- Bei M, Hao S, Lin K, et al. Splicing factor TRA2A contributes to esophageal cancer progression via a noncanonical role in lncRNA m6 A methylation. Cancer Sci. 2023;114:3216-3229. doi: 10.1111/cas.15870
- Su T, Liu J, Zhang N, et al. New insights into the interplay between m6A modifications and microRNA or lncRNA in gastrointestinal cancers. Front Cell Dev Biol. 2023;11:1157797. doi: 10.3389/fcell.2023.1157797
- Hu X, Peng WX, Zhou H, et al. IGF2BP2 regulates DANCR by serving as an N6-methyladenosine reader. Cell Death Differ. 2020;27(6):1782-1794. doi: 10.1038/s41418-019-0461-z
- Xu Y, Weng X, Qiu J, Wang S. Biogenesis of circRBM33 mediated by N6-methyladenosine and its function in abdominal aortic aneurysm. Epigenetics. 2024;19(1):2392401. doi: 10.1080/15592294.2024.2392401
- Zhang Y, Chen Z, Song J, Qian H, Wang Y, Liang Z. The role of m6A modified circ0049271 induced by MNNG in precancerous lesions of gastric cancer. Heliyon. 2024;10(16):e35654. doi: 10.1016/j.heliyon.2024.e35654
- Liu F, Gu W, Shao Y. Cross-talk between circRNAs and m6A modifications in solid tumors. J Transl Med. 2024;22(1):694. doi: 10.1186/s12967-024-05500-4
- Shu G, Zhao Z, Zhao T, et al. N6-methyladenosine modification of circMARK2 enhances cytoplasmic export and stabilizes LIN28B, contributing to the progression of Wilms tumor. J Exp Clin Cancer Res. 2024;43(1):191. doi: 10.1186/s13046-024-03113-9
- Yankova E, Blackaby W, Albertella M, et al. Small-molecule inhibition of METTL3 as a strategy against myeloid leukemia. Nature. 2021;593:597-601. doi: 10.1038/s41586-021-03536-w
- Fiorentino F, Menna M, Rotili D, Valente S, Mai A. METTL3 from target validation to the first small-molecule inhibitors: A medicinal chemistry journey. J Med Chem. 2023;66(3):1654-1677. doi: 10.1021/acs.jmedchem.2c01601
- Qing Y, Dong L, Gao L, et al. R-2-hydroxyglutarate attenuates aerobic glycolysis in leukemia by targeting the FTO/m6A/ PFKP/LDHB axis. Mol Cell. 2021;81:922-939.e9. doi: 10.1016/j.molcel.2020.12.026
- Ofir-Rosenfeld Y, Rausch O, McMahon J, et al. STC-15, an oral small molecule inhibitor of the RNA methyltransferase METTL3, inhibits tumor growth through activation of anti-cancer immune responses and synergises with immune checkpoint blockade. J Immunother Cancer. 2022;10:A1-A1603.
- Guan Q, Lin H, Miao L, et al. Functions, mechanisms, and therapeutic implications of METTL14 in human cancer. J Hematol Oncol. 2022;15:13. doi: 10.1186/s13045-022-01231-5
- Albert L, Xu J, Wan R, Srinivasan V, Dou Y, Vázquez O. Controlled inhibition of methyltransferases using photoswitchable peptidomimetics: Toward an epigenetic regulation of leukemia. Chem Sci. 2017;8:4612-4618. doi: 10.1039/c7sc00137a
- Lee JH, Choi N, Kim S, Jin MS, Shen H, Kim YC. Eltrombopag as an allosteric inhibitor of the METTL3-14 complex affecting the m6A methylation of RNA in acute myeloid leukemia cells. Pharmaceuticals (Basel). 2022;15(4):440. doi: 10.3390/ph15040440
- Nai F, Flores Espinoza MP, Invernizzi A, et al. Small-molecule inhibitors of the m7G-RNA writer METTL1. ACS Bio Med Chem Au. 2023;4(2):100-110. doi: 10.1021/acsbiomedchemau.3c00030
- Li F, Li W. Readers of RNA modification in cancer and their anticancer inhibitors. Biomolecules. 2024;14(7):881. doi: 10.3390/biom14070881
- Dahlem C, Abuhaliema A, Kessler SM, et al. First small-molecule inhibitors targeting the RNA-binding protein IGF2BP2/IMP2 for cancer therapy. ACS Chem Biol. 2022;17(2):361-375. doi: 10.1021/acschembio.1c00833
- Wallis N, Oberman F, Shurrush K, et al. A small molecule inhibitor of Igf2bp1 represses Kras and a prooncogenic phenotype in cancer cells. RNA Biol. 2022;19(1):26-43. doi: 10.1080/15476286.2021.2010983
- Feng P, Chen D, Wang X, et al. Inhibition of the m6A reader IGF2BP2 as a strategy against T-cell acute lymphoblastic leukemia. Leukemia. 2022;36(9):2180-2188. doi: 10.1038/s41375-022-01651-9
- Bao Y, Zhai J, Chen H, et al. Targeting m6A reader YTHDF1 augments antitumour immunity and boosts anti-PD-1 efficacy in colorectal cancer. Gut. 2023;72:1497-1509. doi: 10.1136/gutjnl-2022-328845
- Chanda S, Lepikhov K, Dahlem C, et al. Gene editing and small molecule inhibitors of the RNA binding protein IGF2BP2/IMP2 show its potential as an anti-cancer drug target. Front Biosci (Landmark Ed). 2024;29(1):41. doi: 10.31083/j.fbl2901041
- Huang Y, Su R, Sheng Y, et al., Small-Molecule Targeting of Oncogenic FTO Demethylase in Acute Myeloid Leukemia. Cancer Cell. 2019, 35(4):677-691.e10. doi: 10.1016/j.ccell.2019.03.006
- Feng G, Wu Y, Hu Y, et al. Small molecule inhibitors targeting m6A regulators. J Hematol Oncol. 2024;17(1):30. doi: 10.1186/s13045-024-01546-5
- Zálešák F, Nai F, Herok M, et al. Structure-based design of a potent and selective YTHDC1 ligand. J Med Chem. 2023;67:9516-9535. doi: 10.1021/acs.jmedchem.4c00599
- Hollebecque A, Salvagni S, Plummer R, et al. Clinical activity of CC-90011, an oral, potent, and reversible LSD1 inhibitor, in advanced malignancies. Cancer (Basel). 2022;128(17):3185-3195. doi: 10.1002/cncr.34366
- Sahafnejad Z, Ramazi S, Allahverdi A. An update of epigenetic drugs for the treatment of cancers and brain diseases: A comprehensive review. Genes (Basel). 2023;14(4):873. doi: 10.3390/genes14040873
- Li Y, Ren Y, Wang Y, et al. A compound AC1Q3QWB selectively disrupts HOTAIR-mediated recruitment of PRC2 and enhances the cancer therapy of DZNep. Theranostics. 2019;9:4608-4623. doi: 10.7150/thno.35188
- Yang L, Tang L, Min Q, et al. Emerging role of RNA modification and long noncoding RNA interaction in cancer. Cancer Gene Ther. 2024;31(6):816-830. doi: 10.1038/s41417-024-00734-2
- Poole RM. Belinostat: First global approval. Drugs. 2014;74:1543-1554. doi: 10.1007/s40265-014-0275-8
- Mann BS, Johnson JR, Cohen MH, Justice R, Pazdur R. FDA approval summary: Vorinostat for treatment of advanced primary cutaneous T-cell lymphoma. Oncologist. 2007;12:1247-1252. doi: 10.1634/theoncologist.12-10-1247
- VanderMolen KM, McCulloch W, Pearce CJ, Oberlies NH. Romidepsin (Istodax, NSC 630176, FR901228, FK228, depsipeptide): A natural product recently approved for cutaneous T-cell lymphoma. J Antibiotics (Tokyo). 2011;64:525-531. doi: 10.1038/ja.2011.35
- Lu X, Ning Z, Li Z, Cao H, Wang X. Development of chidamide for peripheral T-cell lymphoma, the first orphan drug approved in China. Intractable Rare Dis Res. 2016;5:185-191. doi: 10.5582/irdr.2016.01024
- Garnock-Jones KP. Panobinostat: First global approval. Drugs. 2015;75:695-704. Erratum in Drugs. 2015;75:929. doi: 10.1007/s40265-015-0388-8
- Ghasemi S. Cancer’s epigenetic drugs: Where they are in the cancer medicines? Pharmacogen J. 2020;20:367-379. doi: 10.1038/s41397-019-0138-5
- Zhou Z, Li HQ, Liu F. DNA methyltransferase inhibitors and their therapeutic potential. Curr Top Med Chem. 2018;18:2448-2457. doi: 10.2174/1568026619666181120150122
- Kantarjian HM, Roboz GJ, Kropf PL, et al. Guadecitabine (SGI-110) in treatment-naive patients with acute myeloid leukemia: Phase 2 results from a multicenter, randomized, phase 1/2 trial. Lancet Oncol. 2017;18:1317-1326. doi: 10.1016/S1470-2045(17)30576-4
- Poltronieri P, Joardar S. Unravelling the interplay between biomolecular condensates and RNA in cancer and diseases. J Biol Regul Homeost Agents, 2024;38(8):5627-5652. doi: 10.23812/j.biol.regul.homeost.agents.20243808.453
- Chen XH, Guo KX, Li J, Xu SH, Zhu H, Yan GR. Regulations of m6A and other RNA modifications and their roles in cancer. Front Med. 2024;18(4):622-648. doi: 10.1007/s11684-024-1064-8
- Gilbert LA, Horlbeck MA, Adamson B, et al. Genome-scale CRISPR-mediated control of gene repression and activation. Cell. 2014;159:647-661. doi: 10.1016/j.cell.2014.09.029
- Shamloo S, Kloetgen A, Petroulia S, et al. Integrative CRISPR activation and small molecule inhibitor screening for lncRNA mediating BRAF inhibitor resistance in melanoma. Biomedicines. 2023;11:2054. doi: 10.3390/biomedicines11072054
- Liu C, Tang H, Hu N, et al. Methylomics and cancer: The current state of methylation profiling and marker development for clinical care. Cancer Cell Int. 2023;23:242. doi: 10.1186/s12935-023-03074-7
- Zhen S, Lu J, Chen W, Zhao L, Li X. Synergistic antitumor effect on bladder cancer by rational combination of programed cell death 1 blockade and CRISPR-Cas9- mediated long non-coding RNA urothelial carcinoma associated 1 knockout. Hum Gene Ther. 2018;29:1352-1363. doi: 10.1089/hum.2018.048
- Gao J, Hou B, Zhu Q, et al. Engineered bioorthogonal POLY-PROTAC nanoparticles for tumor-specific protein degradation and precise cancer therapy. Nat Commun. 2022;13(1):4318. doi: 10.1038/s41467-022-32050-4
- Wang C, Zhang Y, Chen W, Wu Y, Xing D. New-generation advanced PROTACs as potential therapeutic agents in cancer therapy. Mol Cancer. 2024;23(1):110. doi: 10.1186/s12943-024-02024-9
- Zhao LP, Rao XN, Zheng RR, et al. Carrier-free nano- PROTACs to amplify photodynamic therapy induced DNA damage through BRD4 degradation. Nano Lett. 2023;23(13):6193-6201. doi: 10.1021/acs.nanolett.3c01812
- Alabi S, Jaime-Figueroa S, Yao Z, et al. Mutant-selective degradation by BRAF-targeting PROTACs. Nat Commun. 2021;12:920. doi: 10.1038/s41467-021-21159-7
- Haj-Yahia S, Nandi A, Benhamou RI. Targeted degradation of structured RNAs via ribonuclease-targeting chimeras (RiboTacs). Expert Opin Drug Discov. 2023;18(8):929-942. doi: 10.1080/17460441.2023.2224960
- Tong Y, Lee Y, Liu X, et al. Programing inactive RNA-binding small molecules into bioactive degraders. Nature. 2023;618(7963):169-179. doi: 10.1038/s41586-023-06091-8
- Ly HH, Daniel S, Soriano SKV, Kis Z, Blakney AK. Optimization of lipid nanoparticles for saRNA expression and cellular activation using a design-of-experiment approach. Mol Pharmacol. 2022;19(6):1892-1905. doi: 10.1021/acs.molpharmaceut.2c00032
- Kovachka S, Panosetti M, Grimaldi B, Azoulay S, Di Giorgio A, Duca M. Small-molecule approaches to targeting RNA. Nat Rev Chem. 2024;8:120-135. doi: 10.1038/s41570-023-00569-9
- Hargrove AE. Small molecule-RNA targeting: Starting with the fundamentals. Chem Commun (Camb). 2020;56:14744-14756. doi: 10.1039/d0cc06796b
- Berdnikova DV. Photoswitches for controllable RNA binding: A future approach in RNA-targeting therapy. Chem Commun (Camb). 2021;57:10819-10826. doi: 10.1039/d1cc04241f
- Chen B, Dragomir MP, Fabris L, et al. The long noncoding RNA CCAT2 induces chromosomal instability through BOP1-AURKB signaling. Gastroenterology. 2020;159:2146-2162. doi: 10.1053/j.gastro.2020.08.018
- Sha S, Yuan D, Liu Y, Han B, Zhong N. Targeting long noncoding RNA DANCR inhibits triple-negative breast cancer progression. Biol Open. 2017;6(9):1310-1316. doi: 10.1242/bio.023135
- Ehmann F, Kuhn A, Pasmooij AMG, et al. Report of the European medicines agency conference on RNA-based medicines. Nucleic Acid Ther. 2024;34:4-11. doi: 10.1089/nat.2023.0021
- Benhamou RI, Suresh BM, Tong Y, et al. DNA-encoded library versus RNA-encoded library selection enables design of an oncogenic noncoding RNA inhibitor. Proc Natl Acad Sci U S A. 2022;119:e2114971119. doi: 10.1073/pnas.2114971119
- Bouchard JJ, Otero JH, Scott DC, et al. Cancer mutations in the tumor suppressor SPOP disrupt the formation of active, phase-separated compartments. Mol Cell. 2018;72(1):19-36.e8. doi: 10.1016/j.molcel.2018.08.027
- Grabocka E, Bar-Sagi D. Mutant KRAS enhances tumor cell fitness by upregulating stress granules. Cell. 2016;167(7):1803-1813.e12. doi: 10.1016/j.cell.2016.11.035
- Zhang J, Zeng Y, Xing Y, et al. Myristoylation-mediated phase separation of EZH2 compartmentalizes STAT3 to promote lung cancer growth. Cancer Lett. 2021;516:84-98. doi: 10.1016/j.canlet.2021.05.035
- Xu WX, Qu Q, Zhuang HH, et al. The burgeoning significance of liquid-liquid phase separation in the pathogenesis and therapeutics of cancers. Int J Biol Sci. 2024;20(5):1652-1668. doi: 10.7150/ijbs.92988
- Li J, Zhang Y, Gu J, et al. Stress granule core protein-derived peptides inhibit assembly of stress granules and improve sorafenib sensitivity in cancer cells. Molecules (Basel). 2024;29(9):2134. doi: 10.3390/molecules29092134
- Bechara C, Sagan S. Cell-penetrating peptides: 20 years later, where do we stand? FEBS Lett. 2013;587:1693-1702. doi: 10.1016/j.febslet.2013.04.031
- Chen Y, Lan T. N-terminal domain of androgen receptor is a major therapeutic barrier and potential pharmacological target for treating castration resistant prostate cancer: A comprehensive review. Front Pharmacol. 2024;15:1451957. doi: 10.3389/fphar.2024.1451957
- Kamagata K, Ariefai M, Takahashi H, et al. Rational peptide design for regulating the liquid-liquid phase separation on the basis of the residue-residue contact energy. Sci Rep. 2022;12(1):13718. doi: 10.1038/s41598-022-17829-1
- Lemos C, Schulze L, Weiske J, et al. Identification of small molecules that modulate mutant p53 condensation. iScience. 2020;23(9):101517. doi: 10.1016/j.isci.2020.101517
- Donlic A, Zafferani M, Padroni G, et al. Regulation of the MALAT1 triple helix stability and in vitro degradation by diphenylfuran. Nucleic Acids Res. 2020;48:7653-7664. doi: 10.1093/nar/gkaa585
- Meyer SM, Tanaka T, Taghavi A, Baisden JT, Grefe M, Disney MD. Optimization of a protein-targeted medicine into an RNA-specific small molecule. ACS Chem Biol. 2023;18(11):2336-2342. doi: 10.1021/acschembio.3c00476
- False JP, Donlic A, Hargrove AE. Targeting RNA with small molecules: From fundamental principles toward the clinic. Chem Soc Rev. 2021;50(4):2224-2243. doi: 10.1039/d0cs01261k
- Xiao H, Yang X, Zhang Y, Zhang Z, Zhang G, Zhang BT. RNA-targeted small-molecule drug discoveries: a machine-learning perspective. RNA Biol. 2023;20(1):384-397. doi: 10.1080/15476286.2023.2223498
- Kim T, Kim TK. Regulatory RNA: From molecular insights to therapeutic frontiers. Exp Mol Med. 2024;56(6):1233-1234. doi: 10.1038/s12276-024-01267-2
- Li K, Wang H, Jiang B, Jin X. TRIM28 in cancer and cancer therapy. Front Genet. 2024;15:1431564. doi: 10.3389/fgene.2024.1431564
- Zhu Y, Li J, Li S, et al. ZMAT2 condensates regulate the alternative splicing of TRIM28 to reduce cellular ROS accumulation, thereby promoting the proliferation of HCC cells. Cell Commun Signal. 2024;22(1):407. doi: 10.1186/s12964-024-01790-9
- Christen KE, Davis RA, Kennedy D. Psammaplysin F increases the efficacy of bortezomib and sorafenib through the regulation of stress granule formation. Int J Biochem Cell Biol. 2019;112:24-38. doi: 10.1016/j.biocel.2019.04.008
- Mitrea DM, Mittasch M, Ferreira Gomes B, Klein IA, Murcko MA. Modulation of biomolecular condensates: A novel approach to drug discovery. Nat Rev Drug Discov. 2022;21(11):841-862. doi: 10.1038/s41573-022-00505-4
- Wheeler RJ, Lee OH, Poser I, et al. Small Molecules for Modulating Protein Driven Liquid-Liquid Phase Separation in Treating Neurodegenerative Disease. BioRixv preprint. doi: 10.1101/721001
- Qu M, He Q, Bao H, et al. The multiple roles of arsenic compounds in phase separation and membraneless organelle formation determine their therapeutic efficacy in tumors. J Pharm Anal. 2024;14(8):100957. doi: 10.1016/j.jpha.2024.02.011
- Li X, Yu Z. Role of liquid-liquid phase separation in cancer: Mechanisms and therapeutic implications. Cancer Innov. 2024;3(5):e144. doi: 10.1002/cai2.144
- Zhang W, Li Z, Wang X, Sun T. Phase separation is regulated by posttranslational modifications and participates in the development of human diseases. Heliyon. 2024;10(13):e34035. doi: 10.1016/j.heliyon.2024.e34035
- Liu Z, Qin Z, Liu Y, et al. Liquid-liquid phase separation: roles and implications in future cancer treatment. Int J Biol Sci. 2023;19(13):4139-4156. doi: 10.7150/ijbs.81521
- Balavaishnavi B, Kamaraj M, Nithya TG, Santhosh P, GokilaLakshmi S, Shaik MR. Regulation of hippo signaling-mediated apoptosis by Rauvolfia tetraphylla in triple-negative breast cancer. Med Oncol. 2024;41(5):103. doi: 10.1007/s12032-024-02341-5
- Wang J, Hu L, Zhang H, Fang Y, Wang T, Wang H. Intracellular condensates of oligopeptides for targeting lysosomes and addressing multiple drug resistance of cancer. Adv Mater. 2022;34(1):e2104704. doi: 10.1002/adma.202104704
- Grosch M, Ittermann S, Rusha E, et al. Nucleus size and DNA accessibility are linked to the regulation of paraspeckle formation in cellular differentiation. BMC Biol. 2020;18:42. doi: 10.1186/s12915-020-00770-y
- Jia Y, Jia R, Dai Z, et al. Stress granules in cancer: Adaptive dynamics and therapeutic implications. iScience. 2024;27(8):110359. doi: 10.1016/j.isci.2024.110359
- Dinh NTM, Nguyen TM, Park MK, Lee CH. Y-box binding protein 1: Unraveling the multifaceted role in cancer development and therapeutic potential. Int J Mol Sci. 2024;25(2):717. doi: 10.3390/ijms25020717
- Shen X, Peng X, Guo Y, et al. YAP/TAZ enhances P-body formation to promote tumorigenesis. Elife. 2024;12:RP88573. doi: 10.7554/eLife.88573
- Tong F, Hu H, Xu Y, et al. Hollow copper sulfide nanoparticles carrying ISRIB for the sensitized photothermal therapy of breast cancer and brain metastases by inhibiting stress granule formation and reprograming tumor-associated macrophages. Acta Pharmacol Sin B. 2023;13:3471-3488. doi: 10.1016/j.apsb.2022.11.003
- Zyryanova AF, Kashiwagi K, Rato C, et al. ISRIB blunts the integrated stress response by allosterically antagonizing the inhibitory effect of phosphorylated eIF2 on eIF2b. Mol Cell. 2021;81:88-103.e6. doi: 10.1016/j.molcel.2020.10.03
- Hou WC, Massey LA, Rhoades D, et al. A PIKfyve modulator combined with an integrated stress response inhibitor to treat lysosomal storage diseases. Proc Natl Acad Sci U S A. 2024;121(34):e2320257121. doi: 10.1073/pnas.2320257121
- Cao X, Zhang Y, Ding Y, Wan Y. Identification of RNA structures and their roles in RNA functions. Nat Rev Mol Cell Biol. 2024;25(10):784-801. doi: 10.1038/s41580-024-00748-6
- He C, Wu CY, Li W, Xu K. Multidimensional super-resolution microscopy unveils nanoscale surface aggregates in the aging of FUS condensates. J Am Chem Soc. 2023;145(44):24240-24248. doi: 10.1021/jacs.3c08674
- Choi AA, Xu K. Single-molecule diffusivity quantification unveils ubiquitous net charge-driven protein-protein interaction. J Am Chem Soc. 2024;146(15):10973-10978. doi: 10.1021/jacs.4c02475
- Yao RW, Rosen MK. Advanced surface passivation for high-sensitivity studies of biomolecular condensates. Proc Natl Acad Sci U S A. 2024;121(22):e2403013121. doi: 10.1101/2024.02.12.580000
- Paul S, Mondal S, Shenogina I, Cui Q. The molecular basis for the increased stability of the FUS-LC fibril at the anionic membrane- and air-water interfaces. Chem Sci. 2024;15(34):13788-13799. doi: 10.1039/d4sc02295e
- Xia C, Colognori D, Jiang X, Xu K, Doudna JA. Single-molecule live-cell RNA imaging with CRISPR-Csm. bioRxiv [Preprint]. 2024:2024.07.14.603457. doi: 10.1101/2024.07.14.603457
- Guan A, Wong JJ. Targeting RNA modifications with pharmacological agents: New frontiers in cancer therapy. Cancer Med. 2024;13(7):e6989.