Parkinson’s disease in a dish: The emerging role of organoids in research and therapy
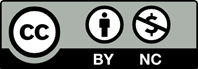
Parkinson’s disease (PD) is a progressive neurodegenerative disorder marked by the degeneration of dopaminergic (DA) neurons in the substantia nigra and the accumulation of α-synuclein aggregates, leading to motor and non-motor dysfunctions. The pathogenesis of PD involves a complex interplay of genetic mutations, environmental factors, and cellular mechanisms, including mitochondrial dysfunction, impaired proteostasis, neuroinflammation, and gut-brain axis dysregulation. Traditional research models, such as animal models and two-dimensional cell cultures, have provided valuable insights but often fall short in replicating the multifaceted and progressive nature of PD, especially in sporadic cases. The emergence of organoid technology offers a transformative approach to PD research. This technology enables the generation of three-dimensional structures that closely mimic the architecture, cellular composition, and functionality of the human midbrain. Midbrain organoids have become pivotal models for investigating disease mechanisms, including DA neuron degeneration, α-synuclein aggregation, and neuroinflammatory responses. Moreover, organoids enable high-throughput drug screening and the identification of potential therapeutic targets. Beyond modeling, recent advancements have demonstrated the feasibility of organoid transplantation as a therapeutic strategy. This review summarizes the current progression of organoid technology in PD research, focusing on its application in modeling pathomechanisms, drug discovery, and therapeutic applications. Despite being in its early stages, organoid technology holds significant promise for advancing our understanding of PD pathogenesis and developing translational therapies.
- Parkinson J. An essay on the shaking palsy. 1817. J Neuropsychiatry Clin Neurosci. 2002;14(2):223-236; discussion 222. doi: 10.1176/jnp.14.2.223
- Goetz CG. The history of parkinson’s disease: Early clinical descriptions and neurological therapies. Cold Spring Harb Perspect Med. 2011;1(1):a008862. doi: 10.1101/cshperspect.a008862
- Sveinbjornsdottir S. The clinical symptoms of Parkinson’s disease. J Neurochem. 2016;139 Suppl 1:318-324. doi: 10.1111/jnc.13691
- German DC, Manaye K, Smith WK, Woodward DJ, Saper CB. Midbrain dopaminergic cell loss in Parkinson’s disease: Computer visualization. Ann Neurol. 1989;26(4):507-514. doi: 10.1002/ana.410260403
- Cookson MR. Alpha-synuclein and neuronal cell death. Mol Neurodegener. 2009;4:9. doi: 10.1186/1750-1326-4-9
- Pickrell AM, Youle RJ. The roles of PINK1, parkin, and mitochondrial fidelity in Parkinson’s disease. Neuron. 2015;85(2):257-273. doi: 10.1016/j.neuron.2014.12.007
- Hirsch EC, Hunot S. Neuroinflammation in Parkinson’s disease: A target for neuroprotection? Lancet Neurol. 2009;8(4):382-397. doi: 10.1016/S1474-4422(09)70062-6
- Pang SY, Lo RC, Ho PW, et al. LRRK2, GBA and their interaction in the regulation of autophagy: Implications on therapeutics in Parkinson’s disease. Transl Neurodegener. 2022;11(1):5. doi: 10.1186/s40035-022-00281-6
- Tan AH, Lim SY, Lang AE. The microbiome-gut-brain axis in Parkinson disease - from basic research to the clinic. Nat Rev Neurol. 2022;18(8):476-495. doi: 10.1038/s41582-022-00681-2
- Schober A. Classic toxin-induced animal models of Parkinson’s disease: 6-OHDA and MPTP. Cell Tissue Res. 2004;318(1):215-224. doi: 10.1007/s00441-004-0938-y
- Dovonou A, Bolduc C, Soto Linan V, Gora C, Peralta Iii MR, Levesque M. Animal models of Parkinson’s disease: Bridging the gap between disease hallmarks and research questions. Transl Neurodegener. 2023;12(1):36. doi: 10.1186/s40035-023-00368-8
- De Haas R, Heltzel LC, Tax D, et al. To be or not to be pink(1): Contradictory findings in an animal model for Parkinson’s disease. Brain Commun. 2019;1(1):fcz016. doi: 10.1093/braincomms/fcz016
- Giraldez-Perez R, Antolin-Vallespin M, Munoz M, Sanchez- Capelo A. Models of α-synuclein aggregation in Parkinson’s disease. Acta Neuropathol Commun. 2014;2:176. doi: 10.1186/s40478-014-0176-9
- Li X, Patel JC, Wang J, et al. Enhanced striatal dopamine transmission and motor performance with LRRK2 overexpression in mice is eliminated by familial Parkinson’s disease mutation G2019S. J Neurosci. 2010;30(5):1788-1797. doi: 10.1523/JNEUROSCI.5604-09.2010
- Cebrian C, Loike JD, Sulzer D. Neuroinflammation in Parkinson’s disease animal models: A cell stress response or a step in neurodegeneration? Curr Top Behav Neurosci. 2015;22:237-270. doi: 10.1007/7854-2014-356
- Sato T, Vries RG, Snippert HJ, et al. Single Lgr5 stem cells build crypt-villus structures in vitro without a mesenchymal niche. Nature. 2009;459(7244):262-265. doi: 10.1038/nature07935
- Smits LM, Reinhardt L, Reinhardt P, et al. Modeling Parkinson’s disease in midbrain-like organoids. NPJ Parkinsons Dis. 2019;5:5. doi: 10.1038/s41531-019-0078-4
- Polymeropoulos MH, Lavedan C, Leroy E, et al. Mutation in the alpha-synuclein gene identified in families with Parkinson’s disease. Science. 1997;276(5321):2045-2047. doi: 10.1126/science.276.5321.2045
- Oliveira LM, Falomir-Lockhart LJ, Botelho MG, et al. Elevated α-synuclein caused by SNCA gene triplication impairs neuronal differentiation and maturation in Parkinson’s patient-derived induced pluripotent stem cells. Cell Death Dis. 2015;6(11):e1994. doi: 10.1038/cddis.2015.318
- Mohamed NV, Sirois J, Ramamurthy J, et al. Midbrain organoids with an SNCA gene triplication model key features of synucleinopathy. Brain Commun. 2021;3(4):fcab223. doi: 10.1093/braincomms/fcab223
- Becerra-Calixto A, Mukherjee A, Ramirez S, et al. Lewy body-like pathology and loss of dopaminergic neurons in midbrain organoids derived from familial Parkinson’s disease patient. Cells. 2023;12(4):625. doi: 10.3390/cells12040625
- Muwanigwa MN, Modamio-Chamarro J, Antony PM, et al. Alpha-synuclein pathology is associated with astrocyte senescence in a midbrain organoid model of familial Parkinson’s disease. Mol Cell Neurosci. 2024;128:103919. doi: 10.1016/j.mcn.2024.103919
- Patikas N, Ansari R, Metzakopian E. Single-cell transcriptomics identifies perturbed molecular pathways in midbrain organoids using α-synuclein triplication Parkinson’s disease patient-derived iPSCs. Neurosci Res. 2023;195:13-28. doi: 10.1016/j.neures.2023.06.001
- Chandra R, Sokratian A, Chavez KR, et al. Gut mucosal cells transfer α-synuclein to the vagus nerve. JCI Insight. 2023;8(23):e172192. doi: 10.1172/jci.insight.172192
- Zhang Q, Liu M, Xu Y, et al. Tilorone mitigates the propagation of α-synucleinopathy in a midbrain-like organoid model. J Transl Med. 2024;22(1):816. doi: 10.1186/s12967-024-05551-7
- Zimprich A, Biskup S, Leitner P, et al. Mutations in LRRK2 cause autosomal-dominant parkinsonism with pleomorphic pathology. Neuron. 2004;44(4):601-607. doi: 10.1016/j.neuron.2004.11.005
- Cherian A, Divya KP, Vijayaraghavan A. Parkinson’s disease - genetic cause. Curr Opin Neurol. 2023;36(4): 292-301. doi: 10.1097/WCO.0000000000001167
- Kim H, Park HJ, Choi H, et al. Modeling G2019S-LRRK2 sporadic Parkinson’s disease in 3D midbrain organoids. Stem Cell Reports. 2019;12(3):518-531. doi: 10.1016/j.stemcr.2019.01.020
- Zagare A, Barmpa K, Smajic S, et al. Midbrain organoids mimic early embryonic neurodevelopment and recapitulate LRRK2-p.Gly2019Ser-associated gene expression. Am J Hum Genet. 2022;109(2):311-327. doi: 10.1016/j.ajhg.2021.12.009
- Zhou ZD, Saw WT, Ho PG, et al. The role of tyrosine hydroxylase-dopamine pathway in Parkinson’s disease pathogenesis. Cell Mol Life Sci. 2022;79(12):599. doi: 10.1007/s00018-022-04574-x
- Su CJ, Feng Y, Liu TT, et al. Thioredoxin-interacting protein induced α-synuclein accumulation via inhibition of autophagic flux: Implications for Parkinson’s disease. CNS Neurosci Ther. 2017;23(9):717-723. doi: 10.1111/cns.12721
- Foliaki ST, Schwarz B, Groveman BR, et al. Neuronal excitatory-to-inhibitory balance is altered in cerebral organoid models of genetic neurological diseases. Mol Brain. 2021;14(1):156. doi: 10.1186/s13041-021-00864-w
- Ohtonen S, Giudice L, Jantti H, et al. Human iPSC-derived microglia carrying the LRRK2-G2019S mutation show a Parkinson’s disease related transcriptional profile and function. Sci Rep. 2023;13(1):22118. doi: 10.1038/s41598-023-49294-9
- Valente EM, Abou-Sleiman PM, Caputo V, et al. Hereditary early-onset Parkinson’s disease caused by mutations in PINK1. Science. 2004;304(5674):1158-1160. doi: 10.1126/science.1096284
- Lucking CB, Durr A, Bonifati V, et al. Association between early-onset Parkinson’s disease and mutations in the parkin gene. N Engl J Med. 2000;342(21):1560-1567. doi: 10.1056/NEJM200005253422103
- Narendra D, Tanaka A, Suen DF, Youle RJ. Parkin is recruited selectively to impaired mitochondria and promotes their autophagy. J Cell Biol. 2008;183(5):795-803. doi: 10.1083/jcb.200809125
- Matsuda N, Sato S, Shiba K, et al. PINK1 stabilized by mitochondrial depolarization recruits parkin to damaged mitochondria and activates latent Parkin for mitophagy. J Cell Biol. 2010;189(2):211-221. doi: 10.1083/jcb.200910140
- Brown SJ, Boussaad I, Jarazo J, et al. PINK1 deficiency impairs adult neurogenesis of dopaminergic neurons. Sci Rep. 2021;11(1):6617. doi: 10.1038/s41598-021-84278-7
- Eldeeb MA, Bayne AN, Fallahi A, et al. Tom20 gates PINK1 activity and mediates its tethering of the TOM and TIM23 translocases upon mitochondrial stress. Proc Natl Acad Sci U S A. 2024;121(10):e2313540121. doi: 10.1073/pnas.2313540121
- Kano M, Takanashi M, Oyama G, et al. Reduced astrocytic reactivity in human brains and midbrain organoids with PRKN mutations. NPJ Parkinsons Dis. 2020;6(1):33. doi: 10.1038/s41531-020-00137-8
- Bus C, Zizmare L, Feldkaemper M, et al. Human dopaminergic neurons lacking PINK1 exhibit disrupted dopamine metabolism related to vitamin B6 co-factors. iScience. 2020;23(12):101797. doi: 10.1016/j.isci.2020.101797
- Ahfeldt T, Ordureau A, Bell C, et al. Pathogenic pathways in early-onset autosomal recessive Parkinson’s disease discovered using isogenic human dopaminergic neurons. Stem Cell Reports. 2020;14(1):75-90. doi: 10.1016/j.stemcr.2019.12.005
- Olgiati S, Quadri M, Fang M, et al. DNAJC6 mutations associated with early-onset Parkinson’s disease. Ann Neurol. 2016;79(2):244-256. doi: 10.1002/ana.24553
- Edvardson S, Cinnamon Y, Ta-Shma A, et al. A deleterious mutation in DNAJC6 encoding the neuronal-specific clathrin-uncoating co-chaperone auxilin, is associated with juvenile parkinsonism. PLoS One. 2012;7(5):e36458. doi: 10.1371/journal.pone.0036458
- Wulansari N, Darsono WH, Woo HJ, et al. Neurodevelopmental defects and neurodegenerative phenotypes in human brain organoids carrying Parkinson’s disease-linked dnajc6 mutations. Sci Adv. 2021;7(8):eabb1540. doi: 10.1126/sciadv.abb1540
- Abela L, Gianfrancesco L, Tagliatti E, et al. Neurodevelopmental and synaptic defects in DNAJC6 Parkinsonism, amenable to gene therapy. Brain. 2024;147(6):2023-2037. doi: 10.1093/brain/awae020
- Bonifati V, Rizzu P, Van Baren MJ, et al. Mutations in the DJ-1 gene associated with autosomal recessive early-onset Parkinsonism. Science. 2003;299(5604):256-259. doi: 10.1126/science.1077209
- Morrone Parfitt G, Coccia E, Goldman C, et al. Disruption of lysosomal proteolysis in astrocytes facilitates midbrain organoid proteostasis failure in an early-onset Parkinson’s disease model. Nat Commun. 2024;15(1):447. doi: 10.1038/s41467-024-44732-2
- Boussaad I, Obermaier CD, Hanss Z, et al. A patient-based model of RNA mis-splicing uncovers treatment targets in Parkinson’s disease. Sci Transl Med. 2020;12(560):eaau3960. doi: 10.1126/scitranslmed.aau3960
- Sidransky E, Samaddar T, Tayebi N. Mutations in GBA are associated with familial Parkinson disease susceptibility and age at onset. Neurology. 2009;73(17):1424-1425, author reply 1425-1426. doi: 10.1212/WNL.0b013e3181b28601
- Nichols WC, Pankratz N, Marek DK, et al. Mutations in GBA are associated with familial Parkinson disease susceptibility and age at onset. Neurology. 2009;72(4):310-316. doi: 10.1212/01.wnl.0000327823.81237.d1
- Rosety I, Zagare A, Saraiva C, et al. Impaired neuron differentiation in GBA-associated Parkinson’s disease is linked to cell cycle defects in organoids. NPJ Parkinsons Dis. 2023;9(1):166. doi: 10.1038/s41531-023-00616-8
- Zagare A, Hemedan A, Almeida C, et al. Insulin resistance is a modifying factor for Parkinson’s disease. Mov Disord. 2024;40(1):67-76. doi: 10.1002/mds.30039
- Frattini E, Faustini G, Lopez G, et al. Lewy pathology formation in patient-derived GBA1 Parkinson’s disease midbrain organoids. Brain. 2025;148(4):1242-1257. doi: 10.1093/brain/awae365
- Baden P, Perez MJ, Raji H, et al. Glucocerebrosidase is imported into mitochondria and preserves complex I integrity and energy metabolism. Nat Commun. 2023;14(1):1930. doi: 10.1038/s41467-023-37454-4
- Jo J, Yang L, Tran HD, et al. Lewy body-like inclusions in human midbrain organoids carrying glucocerebrosidase and α-synuclein mutations. Ann Neurol. 2021;90(3):490-505. doi: 10.1002/ana.26166
- Mazzulli JR, Xu YH, Sun Y, et al. Gaucher disease glucocerebrosidase and α-synuclein form a bidirectional pathogenic loop in synucleinopathies. Cell. 2011; 146(1):37-52. doi: 10.1016/j.cell.2011.06.001
- Ysselstein D, Nguyen M, Young TJ, et al. LRRK2 kinase activity regulates lysosomal glucocerebrosidase in neurons derived from Parkinson’s disease patients. Nat Commun. 2019;10(1):5570. doi: 10.1038/s41467-019-13413-w
- Sanyal A, DeAndrade MP, Novis HS, et al. Lysosome and inflammatory defects in GBA1-mutant astrocytes are normalized by LRRK2 inhibition. Mov Disord. 2020;35(5):760-773. doi: 10.1002/mds.27994
- Li ZF, Cui L, Jin MM, et al. A matrigel-based 3D construct of SH-SY5Y cells models the α-synuclein pathologies of Parkinson’s disease. Dis Model Mech. 2022;15(3):dmm049125. doi: 10.1242/dmm.049125
- Chlebanowska P, Tejchman A, Sulkowski M, Skrzypek K, Majka M. Use of 3D organoids as a model to study idiopathic form of Parkinson’s disease. Int J Mol Sci. 2020;21(3):694. doi: 10.3390/ijms21030694
- Elitt MS, Barbar L, Tesar PJ. Drug screening for human genetic diseases using iPSC models. Hum Mol Genet. 2018;27(R2):R89-R98. doi: 10.1093/hmg/ddy186
- Cetin S, Knez D, Gobec S, Kos J, Pislar A. Cell models for Alzheimer’s and Parkinson’s disease: At the interface of biology and drug discovery. Biomed Pharmacother. 2022;149:112924. doi: 10.1016/j.biopha.2022.112924
- Zhang QS, Heng Y, Mou Z, Huang JY, Yuan YH, Chen NH. Reassessment of subacute MPTP-treated mice as animal model of Parkinson’s disease. Acta Pharmacol Sin. 2017;38(10):1317-1328. doi: 10.1038/aps.2017.49
- Cannon JR, Tapias V, Na HM, Honick AS, Drolet RE, Greenamyre JT. A highly reproducible rotenone model of Parkinson’s disease. Neurobiol Dis. 2009;34(2):279-290. doi: 10.1016/j.nbd.2009.01.016
- Giasson BI, Duda JE, Quinn SM, Zhang B, Trojanowski JQ, Lee VM. Neuronal alpha-synucleinopathy with severe movement disorder in mice expressing A53T human alpha-synuclein. Neuron. 2002;34(4):521-533. doi: 10.1016/s0896-6273(02)00682-7
- Jackson-Lewis V, Przedborski S. Protocol for the MPTP mouse model of Parkinson’s disease. Nat Protoc. 2007;2(1):141-151. doi: 10.1038/nprot.2006.342
- Kim MS, Ra EA, Kweon SH, et al. Advanced human iPSC-based preclinical model for Parkinson’s disease with optogenetic alpha-synuclein aggregation. Cell Stem Cell. 2023;30(7):973-986.e11. doi: 10.1016/j.stem.2023.05.015
- Zhu W, Tao M, Hong Y, et al. Dysfunction of vesicular storage in young-onset Parkinson’s patient-derived dopaminergic neurons and organoids revealed by single cell electrochemical cytometry. Chem Sci. 2022;13(21):6217-6223. doi: 10.1039/d2sc00809b
- Jarazo J, Barmpa K, Modamio J, et al. Parkinson’s disease phenotypes in patient neuronal cultures and brain organoids improved by 2-hydroxypropyl-β-cyclodextrin treatment. Mov Disord. 2022;37(1):80-94. doi: 10.1002/mds.28810
- Sai M, Hank EC, Tai HM, et al. Development of nurr1 agonists from amodiaquine by scaffold hopping and fragment growing. Commun Chem. 2024;7(1):149. doi: 10.1038/s42004-024-01224-0
- Jin Y, Li F, Li Z, et al. Modeling lewy body disease with SNCA triplication iPSC-derived cortical organoids and identifying therapeutic drugs. Sci Adv. 2024;10(37):eadk3700. doi: 10.1126/sciadv.adk3700
- Quadrato G, Nguyen T, Macosko EZ, et al. Cell diversity and network dynamics in photosensitive human brain organoids. Nature. 2017;545(7652):48-53. doi: 10.1038/nature22047
- Pollen AA, Bhaduri A, Andrews MG, et al. Establishing cerebral organoids as models of human-specific brain evolution. Cell. 2019;176(4):743-756 e17. doi: 10.1016/j.cell.2019.01.017
- Brancati G, Treutlein B, Camp JG. Resolving neurodevelopmental and vision disorders using organoid single-cell multi-omics. Neuron. 2020;107(6):1000-1013. doi: 10.1016/j.neuron.2020.09.001
- Phan N, Hong JJ, Tofig B, et al. A simple high-throughput approach identifies actionable drug sensitivities in patient-derived tumor organoids. Commun Biol. 2019;2:78. doi: 10.1038/s42003-019-0305-x
- Zhou C, Wu Y, Wang Z, et al. Standardization of organoid culture in cancer research. Cancer Med. 2023;12(13): 14375-14386. doi: 10.1002/cam4.5943
- Revah O, Gore F, Kelley KW, et al. Maturation and circuit integration of transplanted human cortical organoids. Nature. 2022;610(7931):319-326. doi: 10.1038/s41586-022-05277-w
- Westerling-Bui AD, Fast EM, Soare TW, et al. Transplanted organoids empower human preclinical assessment of drug candidate for the clinic. Sci Adv. 2022;8(27):eabj5633. doi: 10.1126/sciadv.abj5633
- Jgamadze D, Harary PM, Castellanos M, et al. Protocol for human brain organoid transplantation into a rat visual cortex to model neural repair. STAR Protoc. 2023; 4(3):102470. doi: 10.1016/j.xpro.2023.102470
- Zheng X, Han D, Liu W, et al. Human iPSC-derived midbrain organoids functionally integrate into striatum circuits and restore motor function in a mouse model of Parkinson’s disease. Theranostics. 2023;13(8):2673-2692. doi: 10.7150/thno.80271
- Fu CL, Jiang X, Dong BC, Li D, She XY, Yao J. Protocol for transplantation of cells derived from human midbrain organoids into a Parkinson’s disease mouse model to restore motor function. STAR Protoc. 2024;5(3):103251. doi: 10.1016/j.xpro.2024.103251
- Fu CL, Dong BC, Jiang X, Li D, Yao J. A cell therapy approach based on iPSC-derived midbrain organoids for the restoration of motor function in a Parkinson’s disease mouse model. Heliyon. 2024;10(2):e24234. doi: 10.1016/j.heliyon.2024.e24234
- Daadi MM, Grueter BA, Malenka RC, Redmond DE Jr., Steinberg GK. Dopaminergic neurons from midbrain-specified human embryonic stem cell-derived neural stem cells engrafted in a monkey model of Parkinson’s disease. PLoS One. 2012;7(7):e41120. doi: 10.1371/journal.pone.0041120
- Nam YR, Kang M, Kim M, et al. Preparation of human astrocytes with potent therapeutic functions from human pluripotent stem cells using ventral midbrain patterning. J Adv Res. 2024;69:181-196. doi: 10.1016/j.jare.2024.03.012
- Andrews MG, Kriegstein AR. Challenges of organoid research. Annu Rev Neurosci. 2022;45:23-39. doi: 10.1146/annurev-neuro-111020-090812
- Bose S, Clevers H, Shen X. Promises and challenges of organoid-guided precision medicine. Med. 2021;2(9): 1011-1026. doi: 10.1016/j.medj.2021.08.005
- Ahammed B, Kalangi SK. A decade of organoid research: Progress and challenges in the field of organoid technology. ACS Omega. 2024;9(28):30087-30096. doi: 10.1021/acsomega.4c03683
- Hong SJ, Bock M, Zhang S, An SB, Han I. Therapeutic transplantation of human central nervous system organoids for neural reconstruction. Int J Mol Sci. 2024;25(15):8540. doi: 10.3390/ijms25158540
- Bellotti C, Samudyata S, Thams S, Sellgren CM, Rostami E. Organoids and chimeras: The hopeful fusion transforming traumatic brain injury research. Acta Neuropathol Commun. 2024;12(1):141. doi: 10.1186/s40478-024-01845-5