3D-Printed disposable nozzles for cost-efficient extrusion-based 3D bioprinting
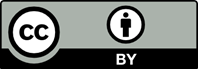
3D bioprinting has significantly impacted tissue engineering with its capability to create intricate structures with complex geometries that were difficult to replicate through traditional manufacturing techniques. Extrusion-based 3D bioprinting methods tend to be limited when creating complex structures using bioinks of low viscosity. However, the capacity for creating multi-material structures that have distinct properties could be unlocked through the mixture of two solutions before extrusion. This could be used to generate architectures with varying levels of stiffness and hydrophobicity, which could be utilized for regenerative medicine applications. Moreover, it allows for combining proteins and other biological materials in a single 3D-bioprinted structure. This paper presents a standardized fabrication method of disposable nozzle connectors (DNC) for 3D bioprinting with hydrogel-based materials. This method entails 3D printing connectors with dual inlets and a single outlet to mix the material internally. The connectors are compatible with conventional Luer lock needles, offering an efficient solution for nozzle replacement. IVZK (Ac-Ile-Val-Cha-Lys-NH2) peptide-based hydrogel materials were used as a bioink with the 3D-printed DNCs. Extrusion-based 3D bioprinting was employed to print shapes of varying complexities, demonstrating potential in achieving high print resolution, shape fidelity, and biocompatibility. Post-printing of human neonatal dermal fibroblasts, cell viability, proliferation, and metabolic activity were observed, which demonstrated the effectiveness of the proposed design and process for 3D bioprinting using low-viscosity bioinks.
Ngo TD, Kashani A, Imbalzano G, et al., 2018, Additive manufacturing (3D printing): A review of materials, methods, applications and challenges. Compos Part B Eng, 143: 172–196. https://doi.org/10.1016/j.compositesb.2018.02.012
Ford S, Minshall T, 2019, Invited review article: Where and how 3D printing is used in teaching and education. Addit Manuf, 25: 131–150. https://doi.org/10.1016/j.addma.2018.10.028
Shahrubudin N, Lee TC, Ramlan R, 2019, An overview on 3D printing technology: Technological, materials, and applications. Procedia Manuf, 35: 1286–1296. https://doi.org/10.1016/j.promfg.2019.06.089
Gao W, Zhang Y, Ramanujan D, et al., 2015, The status, challenges, and future of additive manufacturing in engineering. Comput Aided Des, 69: 65–89. https://doi.org/10.1016/j.cad.2015.04.001
Avila-Ramírez A, Valle-Pérez AU, Susapto HH, et al., 2021, Ecologically friendly biofunctional ink for reconstruction of rigid living systems under wet conditions. Int J Bioprint, 7: 398. https://doi.org/10.18063/ijb.v7i4.398
Albalawi HI, Khan ZN, Valle-Pérez AU, et al., 2021, Sustainable and eco-friendly coral restoration through 3D printing and fabrication. ACS Sustain. Chem Eng, 9: 12634–12645. https://doi.org/10.1021/acssuschemeng.1c04148
Wangpraseurt D, You S, Azam F, et al., 2020, et al. Bionic 3D printed corals. Nat Commun, 11: 1748. https://doi.org/10.1038/s41467-020-15486-4
Melchels FP, Domingos MA, Klein TJ, et al., 2012, Additive manufacturing of tissues and organs. Prog Polym Sci, 37: 1079–1104. https://doi.org/10.1016/j.progpolymsci.2011.11.007
Murphy SV, De Coppi P, Atala A, 2019, Opportunities and challenges of translational 3D bioprinting. Nat Biomed Eng, 4: 370–380. https://doi.org/10.1038/s41551-019-0471-7
Ng WL, Lee JM, Zhou M, et al., 2020, Vat polymerization-based bioprinting-process, materials, applications and regulatory challenges. Biofabrication, 12: 022001. https://doi.org/10.1088/1758-5090/ab6034
Li W, Mille LS, Robledo JA, et al., 2020, Recent advances in formulating and processing biomaterial inks for vat polymerization‐based 3D printing. Adv Healthc Mater, 9: 2000156. https://doi.org/10.1002/adhm.202000156
Elomaa L, Gerbeth L, Almalla A, et al., 2023, Bioactive photocrosslinkable resin solely based on refined decellularized small intestine submucosa for vat photopolymerization of in vitro tissue mimics. Addit Manuf, 64: 103439. https://doi.org/10.26434/chemrxiv-2022-f2hpc
Wang Y, Wang J, Ji Z, et al., 2022, Application of bioprinting in ophthalmology. Int J Bioprint, 8: 552. https://doi.org/10.18063/ijb.v8i2.552
Ozbolat IT, Hospodiuk M, 2016, Current advances and future perspectives in extrusion-based bioprinting. Biomaterials, 76: 321–343. https://doi.org/10.1016/j.biomaterials.2015.10.076
Xing J, Luo X, Bermudez J, et al., 2017, 3D Bioprinting of Scaffold Structure using Micro-Extrusion Technology. In: The Annual International Solid Freeform Fabrication Symposium, Austin. https://doi.org/10.26153/16943
De León EH, Valle-Pérez AU, Khan ZN, et al., 2023, Intelligent and smart biomaterials for sustainable 3D printing applications. Curr Opin Biomed Eng, 26: 100450. https://doi.org/10.1016/j.cobme.2023.100450
Pradeep PV, Paul L, 2022, Review on novel biomaterials and innovative 3D printing techniques in biomedical applications. Mater Today Proc., 58: 96–103. https://doi.org/10.1016/j.matpr.2022.01.072
Xu H, Su Y, Liao Z, et al., 2022, Coaxial bioprinting vascular constructs: A review. Eur Polym J, 179: 111549. https://doi.org/10.1016/j.eurpolymj.2022.111549
Morgan FL, Moroni L, Baker MB, 2020, Dynamic bioinks to advance bioprinting. Adv Healthc Mater, 9: 1901798. https://doi.org/10.1002/adhm.201901798
Colosi C, Shin SR, Manoharan V, et al., 2016, Microfluidic bioprinting of heterogeneous 3D tissue constructs using low-viscosity bioink. Adv Mater, 28: 677–684. https://doi.org/10.1002/adma.201503310
Khan ZN, Albalawi HI, Valle-Pérez AU, et al., 2022, From 3D printed molds to bioprinted scaffolds: A hybrid material extrusion and vat polymerization bioprinting approach for soft matter constructs. Mater Sci Addit Manuf, 1: 7. https://doi.org/10.18063/msam.v1i1.7
Abdelrahman S, Alsanie WF, Khan ZN, et al., 2022, A Parkinson’s disease model composed of 3D bioprinted dopaminergic neurons within a biomimetic peptide scaffold. Biofabrication, 14: 044103. https://doi.org/10.1088/1758-5090/ac7eec
Skylar-Scott MA, Mueller J, Visser CW, et al., 2019, Voxelated soft matter via multimaterial multinozzle 3D printing. Nature, 575: 330–335. https://doi.org/10.1038/s41586-019-1736-8
Alrashoudi AA, Albalawi HI, Aldoukhi AH, et al., 2021, Fabrication of a lateral flow assay for rapid in-field detection of COVID-19 antibodies using additive manufacturing printing technologies. Int J Bioprint, 7: 399. https://doi.org/10.18063/ijb.v7i4.399
Khan Z, Kahin K, Rauf S, et al., 2018, Optimization of a 3D bioprinting process using ultrashort peptide bioinks. Int J Bioprint, 5: 173. https://doi.org/10.18063/ijb.v5i1.173
Susapto HH, Alhattab D, Abdelrahman S, et al., 2021, Ultrashort peptide bioinks support automated printing of large-scale constructs assuring long-term survival of printed tissue constructs. Nano Lett, 21: 2719–2729. https://doi.org/10.1021/acs.nanolett.0c04426
Kahin K, Khan Z, Albagami M, et al., 2019, Development of a robotic 3D bioprinting and microfluidic pumping system for tissue and organ engineering. In: Gray BL, Becker H, editors. Microfluidics, BioMEMS, and Medical Microsystems XVII. 25 (SPIE, 2019). https://doi.org/10.1117/12.2507237
Khan Z, Kahin K, Hauser C, 2021, Time-dependent pulsing of microfluidic pumps to enhance 3D bioprinting of peptide bioinks. In: Gray BL, Becker H, editors. Microfluidics, BioMEMS, and Medical Microsystems XIX, 5 (SPIE, 2021). https://doi.org/10.1117/12.2578830