Benefit of noninvasive vagus nerve stimulation in vaccine optimization for young children
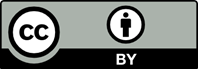
Whether it is mandatory to vaccinate young children against SARS-CoV-2 and respiratory syncytial virus (RSV) is still an ongoing topic of debate. Indeed, vaccine acceptance for young children is either too low (in the case of COVID-19) or, in some cases, unattainable (with the demand far exceeding the capacity of production in the case of RSV vaccines in some countries). In addition, while vaccines do confer immunity, they can be complicated by inflammatory reactions to the vaccine itself. This inflammatory response is controlled by the nervous system, specifically the vagus nerve. Vagal tone optimization in and of itself confers some level of protection against viral infections such as SARS-CoV-2 or RSV, but the degree of protection has not been adequately evaluated. Even though additional studies are needed to validate a strategy of vagal optimization as an alternative to or co-treatment with vaccines, studies of noninvasive vagus nerve stimulation should be supported by public health agencies as an adjunctive tool providing young children with safe, ready-to-use immunization and protection from vaccine reactions. This recommendation is based on scientific, epidemiological, ethical, and economic considerations.
- Pavlov VA, Wang H, Czura CJ, Friedman SG, Tracey KJ. The cholinergic anti-inflammatory pathway: A missing link in neuroimmunomodulation. Mol Med. 2003;9(58):125134.
- Andersson U, Tracey KJ. Vagus nerve SARS‐CoV‐2 infection and inflammatory reflex dysfunction: Is there a causal relationship? J Intern Med. 2024;295(1):91–102. doi: 10.1111/joim.13746
- Johnson RL, Wilson CG. A review of vagus nerve stimulation as a therapeutic intervention. J Inflamm Res. 2018;11:203213. doi: 10.2147/JIR.S163248
- Kawai H, Kishimoto M, Okahisa Y, Sakamoto S, Terada S, Takaki M. Initial outcomes of the safe and sound protocol on patients with adult autism spectrum disorder: Exploratory pilot study. Int J Environ Res Public Health. 2023;20(6):4862. doi: 10.3390/ijerph20064862
- Yap JYY, Keatch C, Lambert E, Woods W, Stoddart PR, Kameneva T. Critical review of transcutaneous vagus nerve stimulation: Challenges for translation to clinical practice. Front Neurosci. 2020;14:284. doi: 10.3389/fnins.2020.00284
- Jenkins DD, Khodaparast N, O’Leary GH, Washburn SN, Covalin A, Badran BW. Transcutaneous auricular neurostimulation (tAN): A novel adjuvant treatment in neonatal opioid withdrawal syndrome. Front Hum Neurosci. 2021;15:648556. doi: 10.3389/fnhum.2021.648556
- Cook DN, Thompson S, Stomberg-Firestein S, et al. Design and validation of a closed-loop, motor-activated auricular vagus nerve stimulation (MAAVNS) system for neurorehabilitation. Brain Stimul. 2020;13(3):800803. doi: 10.1016/j.brs.2020.02.028
- Badran BW, Jenkins DD, Cook D, et al. Transcutaneous auricular vagus nerve stimulation-paired rehabilitation for oromotor feeding problems in newborns: An open-label pilot study. Front Hum Neurosci. 2020;14:77. doi: 10.3389/fnhum.2020.00077
- Agorastos A, Mansueto AC, Hager T, Pappi E, Gardikioti A, Stiedl O. Heart rate variability as a translational dynamic biomarker of altered autonomic function in health and psychiatric disease. Biomedicines. 2023;11(6):1591. doi: 10.3390/biomedicines11061591
- Alen NV, Parenteau AM, Sloan RP, Hostinar CE. Heart rate variability and circulating inflammatory markers in midlife. Brain Behav Immun Health. 2021;15:100273. doi: 10.1016/j.bbih.2021.100273
- Adam J, Rupprecht S, Künstler ECS, Hoyer D. Heart rate variability as a marker and predictor of inflammation, nosocomial infection, and sepsis - a systematic review. Auton Neurosci. 2023;249:103116. doi: 10.1016/j.autneu.2023.103116
- Aragón-Benedí C, Caballero-Lozada AF, Perez- Calatayud AA, et al. Prospective multicenter study of heart rate variability with ANI monitor as predictor of mortality in critically ill patients with COVID-19. Sci Rep. 2022;12(1):21762. doi: 10.1038/s41598-022-25537-z
- Kuo TBJ, Lai CJ, Huang Y, Yang CCH. Regression analysis between heart rate variability and baroreflex‐related vagus nerve activity in rats. J Cardiovasc Electrophysiol. 2005;16(8):864869. doi: 10.1111/j.1540-8167.2005.40656.x
- Machetanz K, Berelidze L, Guggenberger R, Gharabaghi A. Transcutaneous auricular vagus nerve stimulation and heart rate variability: Analysis of parameters and targets. Auton Neurosci. 2021;236:102894. doi: 10.1016/j.autneu.2021.102894
- Geng D, Liu X, Wang Y, Wang J. The effect of transcutaneous auricular vagus nerve stimulation on HRV in healthy young people. PLoS One. 2022;17(2):e0263833. doi: 10.1371/journal.pone.0263833
- Clancy JA, Mary DA, Witte KK, Greenwood JP, Deuchars SA, Deuchars J. Non-invasive vagus nerve stimulation in healthy humans reduces sympathetic nerve activity. Brain Stimul. 2014;7(6):871877. doi: 10.1016/j.brs.2014.07.031
- Yokota H, Edama M, Hirabayashi R, et al. Effects of stimulus frequency, intensity, and sex on the autonomic response to transcutaneous vagus nerve stimulation. Brain Sci. 2022;12(8):1038. doi: 10.3390/brainsci12081038
- Carandina A, Rodrigues GD, Di Francesco P, et al. Effects of transcutaneous auricular vagus nerve stimulation on cardiovascular autonomic control in health and disease. Auton Neurosci. 2021;236:102893. doi: 10.1016/j.autneu.2021.102893
- Jensen MK, Andersen SS, Andersen SS, Liboriussen CH, Kristensen S, Jochumsen M. modulating heart rate variability through deep breathing exercises and transcutaneous auricular vagus nerve stimulation: A study in healthy participants and in patients with rheumatoid arthritis or systemic lupus erythematosus. Sensors (Basel). 2022;22(20):7884. doi: 10.3390/s22207884
- Sloan RP, Cole SW. Parasympathetic neural activity and the reciprocal regulation of innate antiviral and inflammatory genes in the human immune system. Brain Behav Immun. 2021;98:251256. doi: 10.1016/j.bbi.2021.08.217
- Ghazaly M, Nadel S. Characteristics of children admitted to intensive care with acute bronchiolitis. Eur J Pediatr. 2018;177(6):913920. doi: 10.1007/s00431-018-3138-6
- Wildenbeest JG, Billard MN, Zuurbier RP, et al. The burden of respiratory syncytial virus in healthy term-born infants in Europe: A prospective birth cohort study. Lancet Respir Med. 2022;11(4):341-353. doi: 10.1016/S2213-2600(22)00414-3
- Stock C, Teyssier G, Pichot V, Goffaux P, Barthelemy JC, Patural H. Autonomic dysfunction with early respiratory syncytial virus-related infection. Auton Neurosci. 2010;156(12):9095. doi: 10.1016/j.autneu.2010.03.012
- Vismara L, Gianmaria Tarantino A, Bergna A, et al. Correlation between diminished vagal tone and somatic dysfunction severity in very and extremely low birth weight preterm infants assessed with frequency spectrum heart rate variability and salivary cortisol. Medicine. 2022;101(38):e30565. doi: 10.1097/MD.0000000000030565
- Milani GP, Bollati V, Ruggiero L, et al. Bronchiolitis and SARS-CoV-2. Arch Dis Child. 2021;106(10):9991001. doi: 10.1136/archdischild-2020-321108
- Kompaniyets L, Agathis NT, Nelson JM, et al. Underlying medical conditions associated with severe COVID-19 illness among children. JAMA Netw Open. 2021;4(6):e2111182. doi: 10.1001/jamanetworkopen.2021.11182
- Mol MBA, Strous MTA, van Osch FHM, et al. Heart-rate-variability (HRV), predicts outcomes in COVID-19. PLoS One. 2021;16(10):e0258841. doi: 10.1371/journal.pone.0258841
- Viljoen M, Claassen N. Allostatic load and heart rate variability as health risk indicators. Afr Health Sci. 2017;17(2):428-435. doi: 10.4314/ahs.v17i2.17
- Silva AKF, Christofaro DGD, Bernardo AFB, Vanderlei FM, Vanderlei LCM. Sensitivity, specificity and predictive value of heart rate variability indices in type 1 diabetes mellitus. Arq Bras Cardiol. 2017;108(3):255-262. doi: 10.5935/abc.20170024
- Banerjee A, Singh N, Raju A, Gupta R. Central markers of obesity affect heart rate variability independent of physical activity in young adults. J Family Med Prim Care. 2022;11(6):2521. doi: 10.4103/jfmpc.jfmpc_1970_21
- Thayer JF, Yamamoto SS, Brosschot JF. The relationship of autonomic imbalance, heart rate variability and cardiovascular disease risk factors. Int J Cardiol. 2010;141(2):122131. doi: 10.1016/j.ijcard.2009.09.543
- Mulkey SB, Govindan R, Metzler M, et al. Heart rate variability is depressed in the early transitional period for newborns with complex congenital heart disease. Clin Auton Res. 2020;30(2):165172. doi: 10.1007/s10286-019-00616-w
- Javorka K, Lehotska Z, Kozar M, et al. Heart rate variability in newborns. Physiol Res. 2017;S203S214. doi: 10.33549/physiolres.933676
- Scala I, Rizzo PA, Bellavia S, et al. Autonomic dysfunction during acute SARS-CoV-2 infection: A systematic review. J Clin Med. 2022;11(13):3883. doi: 10.3390/jcm11133883
- Bonaz B, Sinniger V, Pellissier S. Targeting the cholinergic anti-inflammatory pathway with vagus nerve stimulation in patients with Covid-19? Bioelectron Med. 2020;6(1):15. doi: 10.1186/s42234-020-00051-7
- Mehranfard D, Speth RC. Cholinergic anti-inflammatory pathway and COVID-19. Bioimpacts. 2022;12(2):171174. doi: 10.34172/bi.2022.23980
- Hajiasgharzadeh K, Jafarlou M, Mansoori B, Dastmalchi N, Baradaran B, Khabbazi A. Inflammatory reflex disruption in COVID ‐19. Clin Exp Neuroimmunol. 2022;13(4):295301.doi: 10.1111/cen3.12703
- Rangon CM, Niezgoda A. Understanding the pivotal role of the vagus nerve in health from pandemics. Bioengineering. 2022;9(8):352. doi: 10.3390/bioengineering9080352
- Wang Q, Iketani S, Li Z, et al. Alarming antibody evasion properties of rising SARS-CoV-2 BQ and XBB subvariants. Cell. 2023;186(2):279-286.e8. doi: 10.1016/j.cell.2022.12.018
- Terliesner N, Unterwalder N, Edelmann A, et al. Viral infections in hospitalized children in Germany during the COVID-19 pandemic: Association with non-pharmaceutical interventions. Front Pediatr. 2022;10:935483. doi: 10.3389/fped.2022.935483
- Jacinto CP, Gastaldi AC, Aguiar DY, Maida KD, Souza HCD. Physical therapy for airway clearance improves cardiac autonomic modulation in children with acute bronchiolitis. Braz J Phys Ther. 2013;17(6):533540. doi: 10.1590/S1413-35552012005000120
- Staats P, Giannakopoulos G, Blake J, Liebler E, Levy RM. The use of non-invasive vagus nerve stimulation to treat respiratory symptoms associated with COVID-19: A theoretical hypothesis and early clinical experience. Neuromodulation. 2020;23(6):784788. doi: 10.1111/ner.13172
- Seitz T, Szeles JC, Kitzberger R, et al. Percutaneous auricular vagus nerve stimulation reduces inflammation in critical Covid-19 patients. Front Physiol. 2022;13:897257. doi: 10.3389/fphys.2022.897257
- Tornero C, Pastor E, Garzando MM, et al. Non-invasive vagus nerve stimulation for COVID-19: Results from a randomized controlled trial (SAVIOR I). Front Neurol. 2022;13:820864. doi: 10.3389/fneur.2022.820864
- Rangon CM, Barruet R, Mazouni A, et al. Auricular neuromodulation for mass vagus nerve stimulation: Insights from SOS COVID-19 a multicentric, randomized, controlled, double-blind French pilot study. Front Physiol. 2021;12:704599. doi: 10.3389/fphys.2021.704599
- Boezaart AP, Botha DA. Treatment of stage 3 COVID-19 with transcutaneous auricular vagus nerve stimulation drastically reduces interleukin-6 blood levels: A report on two cases. Neuromodulation. 2021;24(1):166167. doi: 10.1111/ner.13293
- Uehara L, Corrêa JCF, Ritti R, et al. Transcutaneous auricular vagus nerve stimulation effects on inflammatory markers and clinical evolution of patients with COVID-19: A pilot randomized clinical trial. Expert Rev Med Devices. 2022;19(11):915920. doi: 10.1080/17434440.2022.2154147
- Jankauskaite L, Malinauskas M, Mickeviciute GC. HMGB1: A potential target of nervus vagus stimulation in pediatric SARS-CoV-2-induced ALI/ARDS. Front Pediatr. 2022;10:884539. doi: 10.3389/fped.2022.884539
- Chrestia JF, Oliveira AS, Mulholland AJ, Gallagher T, Bermúdez I, Bouzat C. A Functional interaction between Y674-R685 region of the SARS-CoV-2 Spike protein and the human α7 nicotinic receptor. Mol Neurobiol. 2022;59(10):60766090. doi: 10.1007/s12035-022-02947-8
- Doria D, Santin AD, Tuszynski JA, Scheim DE, Aminpour M. Omicron SARS-CoV-2 Spike-1 protein’s decreased binding affinity to α7nAChr: Implications for autonomic dysregulation of the parasympathetic nervous system and the cholinergic anti-inflammatory pathway-an in silico analysis. BioMedInformatics. 2022;2(4):553564. doi: 10.3390/biomedinformatics2040035
- Woo MS, Shafiq M, Fitzek A, et al. Vagus nerve inflammation contributes to dysautonomia in COVID-19. Acta Neuropathol. 2023;146(3):387394. doi: 10.1007/s00401-023-02612-x
- Tanmay S, Labrou D, Farsalinos K, Poulas K. Is SARS-CoV-2 spike glycoprotein impairing macrophage function via α7-nicotinic acetylcholine receptors? Food Chem Toxicol. 2021;152:112184. doi: 10.1016/j.fct.2021.112184
- Godellas NE, Cymes GD, Grosman C. An experimental test of the nicotinic hypothesis of COVID-19. Proc Natl Acad Sci U S A. 2022;119(44):e2204242119. doi: 10.1073/pnas.2204242119
- Zoli M, Pucci S, Vilella A, Gotti C. Neuronal and extraneuronal nicotinic acetylcholine receptors. Curr Neuropharmacol. 2018;16(4):338349. doi: 10.2174/1570159X15666170912110450
- Bulfamante G, Bocci T, Falleni M, Campiglio L, Coppola S, Tosi D, et al. Brainstem neuropathology in two cases of COVID-19: SARS-CoV-2 trafficking between brain and lung. J Neurol. 2021;268(12):4486‑91. doi: 10.1007/s00415-021-10604-8
- Basha S, Surendran N, Pichichero M. Immune responses in neonates. Expert Rev Clin Immunol. 2014;10(9):11711184. doi: 10.1586/1744666X.2014.942288
- Cooper DM, Afghani B, Byington CL, et al. SARS-CoV-2 vaccine testing and trials in the pediatric population: Biologic, ethical, research, and implementation challenges. Pediatr Res. 2021;90(5):966970. doi: 10.1038/s41390-021-01402-z
- Farber DL, Netea MG, Radbruch A, Rajewsky K, Zinkernagel RM. Immunological memory: Lessons from the past and a look to the future. Nat Rev Immunol. 2016;16(2):124128. doi: 10.1038/nri.2016.13
- Netea MG, Schlitzer A, Placek K, Joosten LAB, Schultze JL. Innate and adaptive immune memory: An evolutionary continuum in the host’s response to pathogens. Cell Host Microbe. 2019;25(1):1326. doi: 10.1016/j.chom.2018.12.006
- Pavlov VA, Tracey KJ. Bioelectronic medicine: Preclinical insights and clinical advances. Neuron. 2022;110(21):36273244. doi: 10.1016/j.neuron.2022.09.003
- Westman M, Saha S, Morshed M, Lampa J. Lack of acetylcholine nicotine alpha 7 receptor suppresses development of collagen-induced arthritis and adaptive immunity. Clin Exp Immunol. 2010;162(1):6267. doi: 10.1111/j.1365-2249.2010.04204.x
- Wu YJ, Wang L, Ji CF, Gu SF, Yin Q, Zuo J. The role of α7nAChR-mediated cholinergic anti-inflammatory pathway in immune cells. Inflammation. 2021;44(3):821834. doi: 10.1007/s10753-020-01396-6
- Pang X, Chen L, Xu G. New awareness of the interplay between the gut microbiota and circadian rhythms. Pol J Microbiol. 2023;72(4):355363. doi: 10.33073/pjm-2023-046
- Ince LM, Barnoud C, Lutes LK, et al. Influence of circadian clocks on adaptive immunity and vaccination responses. Nat Commun. 2023;14(1):476. doi: 10.1038/s41467-023-35979-2
- Waggoner SN. Circadian rhythms in immunity. Curr Allergy Asthma Rep. 2020;20(1):2. doi: 10.1007/s11882-020-0896-9
- Patton AP, Hastings MH. The mammalian circadian time-keeping system. J Huntingtons Dis. 2023;12(2):91104. doi: 10.3233/JHD-230571
- García-García A, Méndez-Ferrer S. The autonomic nervous system pulls the strings to coordinate circadian HSC functions. Front Immunol. 2020;11:956. doi: 10.3389/fimmu.2020.00956
- García-García A, Korn C, García-Fernández M, et al. Dual cholinergic signals regulate daily migration of hematopoietic stem cells and leukocytes. Blood. 2019;133(3):224236. doi: 10.1182/blood-2018-08-867648
- Groff J, Vasudevan S, Yaghouby F. Vagus nerve stimulation unequally disturbs circadian variation of cardiac rhythms in male and female rats. Annu Int Conf IEEE Eng Med Biol Soc. 2020;2020:33843387. doi: 10.1109/EMBC44109.2020.9176140
- Geng D, Yang K, Fu Z, Zhang Y, Wang C, An H. Circadian stage-dependent and stimulation duration effects of transcutaneous auricular vagus nerve stimulation on heart rate variability. PLoS One. 2022;17(11):e0277090. doi: 10.1371/journal.pone.0277090
- Ragozzino FJ, Peterson BA, Karatsoreos IN, Peters JH. Circadian regulation of glutamate release pathways shapes synaptic throughput in the brainstem nucleus of the solitary tract (NTS). J Physiol. 2023;601(10):18811896. doi: 10.1113/JP284370
- Chrobok L, Northeast RC, Myung J, Cunningham PS, Petit C, Piggins HD. Timekeeping in the hindbrain: A multi-oscillatory circadian centre in the mouse dorsal vagal complex. Commun Biol. 2020;3(1):225. doi: 10.1038/s42003-020-0960-y
- Ledford H. Do vaccines protect against long COVID? What the data say. Nature. 2021;599(7886):546548. doi: 10.1038/d41586-021-03495-2
- Akinosoglou K, Tzivaki I, Marangos M. Covid-19 vaccine and autoimmunity: Awakening the sleeping dragon. Clin Immunol. 2021;226:108721. doi: 10.1016/j.clim.2021.108721
- Al-Beltagi M, Saeed NK, Bediwy AS. COVID-19 disease and autoimmune disorders: A mutual pathway. World J Methodol. 2022;12(4):200223. doi: 10.5662/wjm.v12.i4.200
- Fernández-de-las-Peñas C, Raveendran AV, Giordano R, Arendt-Nielsen L. Long COVID or post-COVID-19 condition: Past, present and future research directions. Microorganisms. 2023;11(12):2959. doi: 10.3390/microorganisms11122959
- Dotan A, Muller S, Kanduc D, David P, Halpert G, Shoenfeld Y. The SARS-CoV-2 as an instrumental trigger of autoimmunity. Autoimmun Rev. 2021;20(4):102792. doi: 10.1016/j.autrev.2021.102792
- Katz-Agranov N, Zandman-Goddard G. Autoimmunity and COVID-19 - the microbiotal connection. Autoimmun Rev. 2021;20(8):102865. doi: 10.1016/j.autrev.2021.102865
- Spragge F, Bakkeren E, Jahn MT, et al. Microbiome diversity protects against pathogens by nutrient blocking. Science. 2023;382(6676):eadj3502. doi: 10.1126/science.adj3502
- Liu Y, Forsythe P. Vagotomy and insights into the microbiota-gut-brain axis. Neurosci Res. 2021;168:2027. doi: 10.1016/j.neures.2021.04.001
- Korenblik V, Brouwer ME, Korosi A, et al. Are neuromodulation interventions associated with changes in the gut microbiota? A systematic review. Neuropharmacology. 2023;223:109318. doi: 10.1016/j.neuropharm.2022.109318
- Fu Q, Xie H, Zhou L, et al. Auricular acupressure for adverse events following immunization after COVID-19 vaccine injection: A multicentre, blinded, randomized controlled trial. Complement Ther Med. 2022;71:102900. doi: 10.1016/j.ctim.2022.102900
- Badran BW, Huffman SM, Dancy M, et al. A pilot randomized controlled trial of supervised, at-home, self-administered transcutaneous auricular vagus nerve stimulation (taVNS) to manage long COVID symptoms. Bioelectron Med. 2022;8(1):13. doi: 10.1186/s42234-022-00094-y
- Larsen NW, Stiles LE, Miglis MG. Preparing for the long-haul: Autonomic complications of COVID-19. Auton Neurosci. 2021;235:102841. doi: 10.1016/j.autneu.2021.102841
- Dani M, Dirksen A, Taraborrelli P, et al. Autonomic dysfunction in “long COVID”: Rationale, physiology and management strategies. Clin Med. 2021;21(1):e63e67. doi: 10.7861/clinmed.2020-0896
- Lo YL. COVID‐19, fatigue, and dysautonomia. J Med Virol. 2021;93(3):1213. doi: 10.1002/jmv.26552
- Barizien N, Le Guen M, Russel S, Touche P, Huang F, Vallée A. Clinical characterization of dysautonomia in long COVID-19 patients. Sci Rep. 2021;11(1):14042. doi: 10.1038/s41598-021-93546-5
- Agergaard J, Gunst JD, Schiøttz-Christensen B, Østergaard L, Wejse C. Long-term prognosis at 1.5 years after infection with wild-type strain of SARS-CoV-2 and alpha, Delta, as well as omicron variants. Int J Infect Dis. 2023;137:126133. doi: 10.1016/j.ijid.2023.10.022
- Broxmeyer HE, Yoder KK, Wu YC, Hutchins GD, Cooper SH, Farag SS. The brain: Is it a next frontier to better understand the regulation and control of hematopoiesis for future modulation and treatment? Stem Cell Rev Rep. 2021;17(4):10831090. doi: 10.1007/s12015-021-10203-0