Biocompatibility of calcitonin receptor fragment peptide-treated 3D-printed bone scaffolds: a muscle pouch implantation study
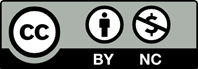
Background and aim: Current synthetic bone graft substitutes (BGS) in development are limited by high resorption, poor load-bearing properties, and stress shielding. These limitations inhibit BGS from complete bio-integration. In this study, we developed calcitonin receptor fragment peptide (CRFP)-treated non-biodegradable MED610 scaffold, seeded with MC3T3 stem cells, and assessed their in vivo biocompatibility and bio-integration.
Methods: Scaffolds were fabricated with Stratasys MED610 (MED610) material, seeded with Mus musculus calvaria cells (MC3T3) and osteogenesis was induced with CRFP after the cells reached confluency and generated bone matrix. Scaffolds with and without bone matrix were implanted in male mice following a muscle pouch implantation protocol. Post-extraction, imaging, staining, and mechanical compression testing was carried after 3 weeks of scaffold implantation in the muscle to measure the ectopic bone formation and compressive strength.
Results: The implanted scaffolds showed significantly higher (p < 0.01) calcium deposits in comparison to the untreated scaffolds. We also found significantly higher (p < 0.001) mineralization on the implanted scaffolds compared to scaffolds before implantation. The mechanical properties of the scaffolds did not vary significantly.
Conclusions: MED610 scaffolds treated with CRFP in vivo do not cause any adverse reaction when implanted in muscle and showed significant ectopic bone formation, indicating biocompatibility and bio-integration.
Relevance for patients: This study will aid in developing biomimetic and biocompatible artificial bones for implantation.
[1] Sohn HS, Oh JK. Review of Bone Graft and Bone Substitutes with an Emphasis on Fracture Surgeries. Biomater Res 2019;23:9.
[2] Myers GJ, Abudu AT, Carter SR, Tillman RM, Grimer RJ. Endoprosthetic Replacement of the Distal Femur for Bone Tumours: Long-term Results. J Bone Joint Surg Br 2007;89:521-6.
[3] Plotz W, Rechl H, Burgkart R, Messmer C, Schelter R, Hipp E, et al. Limb Salvage with Tumor Endoprostheses for Malignant Tumors of the Knee. Clin Orthop Relat Res 2002;405:207-15.
[4] Engh CA, Bobyn JD, Glassman AH. Porous-coated Hip Replacement. The Factors Governing Bone Ingrowth, Stress Shielding, and Clinical Results. J Bone Joint Surg Br 1987;69:45-55.
[5] Epari DR, Taylor WR, Heller MO, Duda GN. Mechanical Conditions in the Initial Phase of Bone Healing. Clin Biomech (Bristol, Avon) 2006;21:646-55.
[6] De Long WG Jr., Einhorn TA, Koval K, McKee M, Smith W, Sanders R, et al. Bone Grafts and Bone Graft Substitutes in Orthopaedic Trauma Surgery. A Critical Analysis. J Bone Joint Surg Am 2007;89:649-58.
[7] Finkemeier CG. Bone-grafting and Bone-graft Substitutes. J Bone Joint Surg Am 2002;84:454-64.
[8] Tomford WW. Transmission of Disease through Transplantation of Musculoskeletal Allografts. J Bone Joint Surg Am 1995;77:1742-54.
[9] Buck BE, Malinin TI, Brown MD. Bone Transplantation and Human Immunodeficiency Virus. An Estimate of Risk of Acquired Immunodeficiency Syndrome (AIDS). Clin Orthop Relat Res 1989;240:129-36.
[10] Boyce T, Edwards J, Scarborough N. Allograft Bone. The Influence of Processing on Safety and Performance. Orthop Clin North Am 1999;30:571-81.
[11] Mroz TE, Joyce MJ, Steinmetz MP, Lieberman IH, Wang JC. Musculoskeletal Allograft Risks and Recalls in the United States. J Am Acad Orthop Surg 2008;16: 559-65.
[12] Ullmark G, Obrant KJ. Histology of Impacted Bone-graft Incorporation. J Arthroplasty 2002;17:150-7.
[13] Fernandez de Grado G, Keller L, Idoux-Gillet Y, Wagner Q, Musset AM, Benkirane-Jessel N, et al. Bone Substitutes: A review of their Characteristics, Clinical Use, and Perspectives for Large Bone Defects Management. J Tissue Eng 2018;9:2 041731418776819.
[14] Wang JC, Alanay A, Mark D, Kanim LE, Campbell PA, Dawson EG, et al. A Comparison of Commercially Available Demineralized Bone Matrix for Spinal Fusion. Eur Spine J 2007;16:1233-40.
[15] Gruskin E, Doll BA, Futrell FW, Schmitz JP, Hollinger JO. Demineralized Bone Matrix in Bone Repair: History and Use. Adv Drug Deliv Rev 2012;64:1063-77.
[16] Campana V, Milano G, Pagano E, Barba M, Cicione C, Salonna G, et al. Bone Substitutes in Orthopaedic Surgery: From Basic Science to Clinical Practice. J Mater Sci Mater Med 2014;25:2445-61.
[17] Russell N, Walsh WR, Lovric V, Kim P, Chen JH, Larson MJ, et al. In-vivo Performance of Seven Commercially Available Demineralized Bone Matrix Fiber and Putty Products in a Rat Posterolateral Fusion Model. Front Surg 2020;7:10.
[18] Chung HJ, Hur JW, Ryu KS, Kim JS, Seong JH. Surgical Outcomes of Anterior Cervical Fusion Using Deminaralized Bone Matrix as Stand-Alone Graft Material: Single Arm, Pilot Study. Korean J Spine 2016;13:114-9.
[19] Saikia KC, Bhattacharya TD, Bhuyan SK, Talukdar DJ, Saikia SP, Jitesh P. Calcium Phosphate Ceramics as Bone Graft Substitutes in Filling Bone Tumor Defects. Indian J Orthop 2008;42:169-72.
[20] Hing KA, Wilson LF, Buckland T. Comparative Performance of Three Ceramic Bone Graft Substitutes. Spine J 2007;7:475-90.
[21] Flatley TJ, Lynch KL, Benson M. Tissue Response toImplants of Calcium Phosphate Ceramic in the Rabbit Spine. Clin Orthop Relat Res 1983;260:246-52.
[22] Koshino T, Murase T, Takagi T, Saito T. New Bone Formation around Porous Hydroxyapatite Wedge Implanted in Opening Wedge High tibial osteotomy in patients with osteoarthritis. Biomaterials 2001;22:1579-82.
[23] Bose S, Vahabzadeh S, Bandyopadhyay A. Bone Tissue Engineering using 3d Printing. Materials Today 2013;16:496-504.
[24] Helguero CG, Mustahsan VM, Parmar S, Pentyala S, Pfail JL, Kao I, et al. Biomechanical Properties of 3d-Printed Bone Scaffolds are Improved by Treatment with Crfp. J Orthop Surg Res 2017;12:195.
[25] Wieding J, Fritsche A, Heinl P, Körner C, Cornelsen M, Seitz H, et al. Biomechanical Behavior of Bone Scaffolds Made of Additive Manufactured Tricalciumphosphate and Titanium Alloy Under Different Loading Conditions. J Appl Biomater Funct Mater 2013;11:e159-66.
[26] Jariwala SH, Lewis GS, Bushman ZJ, Adair JH, Donahue HJ. 3D Printing of Personalized Artificial Bone Scaffolds. 3D Print Addit Manuf 2015;2:56-64.
[27] Konopnicki S, Sharaf B, Resnick C, Patenaude A, PogalSussman T, Hwang KG, et al. Tissue-engineered Bone with 3-Dimensionally Printed β-tricalcium Phosphate and Polycaprolactone Scaffolds and Early Implantation: An In Vivo Pilot Study in a Porcine Mandible Model. J Oral Maxillofac Surg 2015;73:1016.e1-11.
[28] Yoshimoto H, Shin YM, Terai H, Vacanti JP. A Biodegradable Nanofiber Scaffold by Electrospinning and Its Potential for Bone Tissue Engineering. Biomaterials 2003;24:2077-82.
[29] Hutmacher DW, Schantz T, Zein I, Ng KW, Teoh SH, Tan KC. Mechanical Properties and Cell Cultural Response of Polycaprolactone Scaffolds Designed and Fabricated Via Fused Deposition Modeling. J Biomed Mater Res 2001;55:203-16.
[30] Kim J, McBride S, Tellis B, Alvarez-Urena P, Song YH, Dean DD, et al. Rapid-Prototyped PLGA/β-TCP/ Hydroxyapatite Nanocomposite Scaffolds in a Rabbit Femoral Defect Model. Biofabrication 2012;4:025003.
[31] Gunatillake PA, Adhikari R. Biodegradable Synthetic Polymers for Tissue Engineering. Eur Cell Mater 2003; 5:1-16.
[32] Helguero CG, Amaya JL, Ramirez EA, Komatsu DE, Kao I, Pentyala S. A Manufacturing Approach to Functional Biomimetic Three-dimensional-printed Bone implants. Proc Inst Mech Eng Part L J Mater Des Appl 2019;233:383-92.
[33] Mustahsan VM, Anugu A, Komatsu DE, Kao I, Pentyala S. Biocompatible Customized 3D Bone Scaffolds Treated with CRFP, an Osteogenic Peptide. Bioengineering (Basel) 2021;8:199.
[34] Stratasys Technical Report: Biocompatible Clear Med610 Material Data Sheet. 2018. https://www.stratasys.com/ siteassets/materials/materials-catalog/biocompatible/mds_ pj_med610_0720a.pdf?v=48e364
[35] Komatsu DE, Hadjiargyrou M, Udin SM, Trasolini NA, Pentyala S. Identification and Characterization of a Synthetic Osteogenic Peptide. Calcif Tissue Int 2015;97:611-23.
[36] Orcel P, Tajima H, Murayama Y, Fujita T, Krane SM, Ogata E, et al. Multiple Domains Interacting with Gs in the Porcine Calcitonin Receptor. Mol Endocrinol 2000;14: 170-82.
[37] McGovern JA, Griffin M, Hutmacher DW. Animal Models for Bone Tissue Engineering and Modelling Disease. Dis Model Mech 2018;11:dmm.033084.
[38] Ben-David D, Kizhner T, Livne E, Srouji S. A Tissuelike Construct of Human Bone Marrow MSCs Composite Scaffold Support In Vivo ectopic bone formation. J Tissue Eng Regen Med 2010;4:30-7.
[39] Le Nihouannen D, Daculsi G, Saffarzadeh A, Gauthier O, Delplace S, Pilet P, et al. Ectopic Bone Formation by Microporous Calcium Phosphate Ceramic Particles in Sheep Muscles. Bone 2005;36:1086-93.
[40] Yao J, Li X, Bao C, Zhang C, Chen Z, Fan H, et al. Ectopic Bone Formation in Adipose-Derived Stromal Cell-seeded Osteoinductive Calcium Phosphate Scaffolds. J Biomater Appl 2010;24:607-24.
[41] Qu D, Li J, Li Y, Khadka A, Zuo Y, Wang H, et al. Ectopic Osteochondral Formation of Biomimetic Porous PVAn-HA/PA6 Bilayered Scaffold and BMSCs Construct in Rabbit. J Biomed Mater Res B Appl Biomater 2011;96: 9-15.
[42] Scott MA, Levi B, Askarinam A, Nguyen A, Rackohn T, Ting K, et al. Brief Review of Models of Ectopic Bone Formation. Stem Cells Dev 2012;21:655-67.
[43] Asatrian G, Chang L, James AW. Muscle Pouch Implantation: An Ectopic bone Formation Model. Methods Mol Biol 2014;1213:185-91.
[44] Wang D, Christensen K, Chawla K, Xiao G, Krebsbach PH, Franceschi RT. Isolation and Characterization of MC3T3-E1 Preosteoblast Subclones with distinct In Vitro and In Vivo Differentiation/Mineralization Potential. J Bone Miner Res 1999;14:893-903.
[45] Tenenbaum HC, Torontali M, Sukhu B. Effects of Bisphosphonates and Inorganic Pyrophosphate on Osteogenesis In Vitro. Bone 1992;13:249-55.
[46] Franceschi RT, Iyer BS, Cui Y. Effects of Ascorbic Acid on Collagen Matrix Formation and Osteoblast Differentiation in Murine MC3T3-E1 Cells. J Bone Miner Res 1994;9: 843-54.
[47] ISO 604: 2002, Plastics-determination of Compressive Properties. Switzerland: International Organization of Standardization; 2002
[48] Udroiu R, Braga IC. Polyjet Technology Applications for Rapid Tooling. MATEC Web Conf 2017;112:03011.
[49] Chacón J, Caminero MA, García-Plaza E, Núnez PJ. Additive Manufacturing of Pla Structures using Fused Deposition Modelling: Effect of Process Parameters on Mechanical Properties and their Optimal Selection. Mater Design 2017;124:143-57.
[50] DeLustro F, Dasch J, Keefe J, Ellingsworth L. Immune Responses to Allogeneic and Xenogeneic Implants of Collagen and Collagen Derivatives. Clin Orthop Relat Res 1990;260:263-79.
[51] Hasegawa T, Miwa M, Sakai Y, Niikura T, Lee SY, Oe K, et al. Efficient Cell-seeding into Scaffolds Improves Bone Formation. J Dent Res 2010;89:854-9.
[52] Polo-Corrales L, Latorre-Esteves M, Ramirez-Vick JE. Scaffold Design for Bone Regeneration. J Nanosci Nanotechnol 2014;14:15-56.
[53] Chen YW, Fang HY, Shie MY, Shen YF. The Musselinspired Assisted Apatite Mineralized on PolyJet Material for Artificial Bone Scaffold. Int J Bioprint 2019; 5:197.
[54] Amirrah IN, Lokanathan Y, Zulkiflee I, Wee MF, Motta A, Fauzi MB. A Comprehensive Review on Collagen Type I Development of Biomaterials for Tissue Engineering: From Biosynthesis to Bioscaffold. Biomedicines 2022l;10:2307.
[55] Vepari C, Kaplan DL. Silk as a Biomaterial. Prog Polym Sci 2007;32:991-1007.
[56] Ding Z, Cheng W, Mia MS, Lu Q. Silk Biomaterials for Bone Tissue Engineering. Macromol Biosci 2021;21:e2100153.
[57] Sahoo DR, Biswal T. Alginate and its Application to Tissue Engineering. SN Appl Sci 2021;3:30.
[58] Ressler A. Chitosan-Based Biomaterials for Bone Tissue Engineering Applications: A Short Review. Polymers (Basel) 2022;14:3430.
[59] Kozusko SD, Riccio C, Goulart M, Bumgardner J, Jing XL, Konofaos P. Chitosan as a Bone Scaffold Biomaterial. J Craniofac Surg 2018;29:1788-93.
[60] Collins MN, Ren G, Young K, Pina S, Reis RL, Oliveira JM. Scaffold Fabrication Technologies and Structure/ Function Properties in Bone Tissue Engineering. Adv Funct Mater 2021;31:2010609.
[61] Engelberg I, Kohn J. Physico-mechanical Properties of Degradable Polymers used in Medical Applications: A Comparative Study. Biomaterials 1991;12:292-304.
[62] Cheung HY, Lau KT, Lu TP, Hui D. A Critical Review on Polymer-Based Bio-engineered Materials for Scaffold Development. Compos Part B Eng 2006;38:291-300.
[63] Rho JY, Kuhn-Spearing L, Zioupos P. Mechanical Properties and the Hierarchical Structure of Bone. Med Eng Phys 1998;20:92-102.
[64] Ginebra MP, Espanol M, Maazouz Y, Bergez V, Pastorino D. Bioceramics and Bone Healing. EFORT Open Rev 2018;3:173-83.
[65] Bohner M, Santoni BL, Döbelin N. β-Tricalcium Phosphate for Bone Substitution: Synthesis and Properties. Acta Biomater 2020;113:23-41.
[66] Kahar NN, Ahmad N, Mariatti M, Yahaya BH, Sulaiman AR, Hamid ZA. A review of Bioceramics Scaffolds for Bone Defects in Different Types of Animal Models: HA and β -TCP. Biomed Phys Eng Express 2022;8.
[67] Han Q, Wang C, Chen H, Zhao X, Wang J. Porous Tantalum and Titanium in Orthopedics: A Review. ACS Biomater Sci Eng 2019;5:5798-824.
[68] Huang G, Pan ST, Qiu JX. The Clinical Application of Porous Tantalum and its New Development for Bone Tissue Engineering. Materials (Basel) 2021;14:2647.
[69] Crovace AM, Lacitignola L, Forleo DM, Staffieri F, Francioso E, Di Meo A, et al. 3D Biomimetic Porous Titanium (Ti6Al4V ELI) Scaffolds for Large Bone Critical Defect Reconstruction: An Experimental Study in Sheep. Animals (Basel) 2020;10:1389.
[70] Qu H, Fu H, Han Z, Sun Y. Biomaterials for Bone Tissue Engineering Scaffolds: A Review. RSC Adv 2019;9:26252-62.
[71] Alizadeh-Osgouei M, Li Y, Wen C. A Comprehensive Review of Biodegradable synthetic polymer-ceramic composites and their Manufacture for Biomedical Applications. Bioact Mater 2018;4:22-36.
[72] Ferreira FV, Otoni CG, Lopes JH, de Souza LP, Mei LH, Lona LM, et al. Ultrathin Polymer Fibers Hybridized with Bioactive Ceramics: A Review on Fundamental Pathways of Electrospinning Towards Bone Regeneration. Mater Sci Eng C Mater Biol Appl 2021;123:111853.
[73] George SM, Nayak C, Singh I, Balani K. Multifunctional Hydroxyapatite Composites for Orthopedic Applications: A Review. ACS Biomater Sci Eng 2022;8:3162-86.
[74] Kołodziejska B, Kaflak A, Kolmas J. Biologically Inspired Collagen/Apatite Composite Biomaterials for Potential Use in Bone Tissue Regeneration-A Review. Materials 2020;13:1748.
[75] Schulze F, Lang A, Schoon J, Wassilew GI, Reichert J. Scaffold Guided Bone Regeneration for the Treatment of Large Segmental Defects in Long Bones. Biomedicines 2023;11:325.