A possible novel therapeutic targets of selinexor in acute lymphoblastic leukemia: a comprehensive review
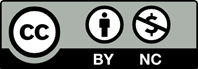
Background and aim: Acute Lymphoblastic Leukemia (ALL) presents a formidable challenge in pediatric and adolescent healthcare due to its aggressive nature and high relapse rates. Despite therapeutic advancements, the demand for more effective treatments remains pressing. In the realm of hematologic malignancies, Selective Inhibitors of Nuclear Export (SINE) have emerged as promising agents, particularly in evading resistance observed with conventional chemotherapy in Acute Myeloid Leukemia (AML). Selinexor, a prominent SINE compound, has exhibited promising anti-leukemic effects in murine models of AML, laying the foundation for its clinical evaluation. Furthermore, Selinexor has been utilized in clinical trials both as a single-agent therapy and in combination with established regimens for a wide range of solid and liquid tumors. However, the precise impact of Selinexor in the context of ALL, specifically as a single agent or in combination therapies, remains unexplored. Unraveling the mechanistic intricacies underlying Selinexor's actions in ALL holds the key to optimizing its efficacy either as a monotherapy or in combination therapies. Notably, within the intricate landscape of ALL pathogenesis, critical factors including the mTOR signaling cascade, aberrations in cancer glucose metabolism, occurrences of alternative splicing, perturbed expressions of dysregulated long noncoding RNAs, and impaired autophagic processes have emerged as pivotal determinants. This comprehensive review undertakes a systematic exploration of potential therapeutic targets that hold the promise of augmenting Selinexor's efficacy within the unique landscape of ALL.
Relevance for patients: This study highlights the possible therapeutic targets of Selinexor in ALL. Understanding the intricate molecular mechanisms, the rational refinement of Selinexor's administration, both as a single agent and as a synergistic component in combination therapies could lead to new avenues for improving the treatment outcomes in ALL patients.
[1] Chen J, Huang C, Zhu Y, Dong L, Cao W, Sun L, et al. Identification of Similarities and Differences Between Myeloid and Lymphoid Acute Leukemias using a Gene-gene Interaction Network. Pathol Res Pract 2015;211:789-96.
[2] Catovsky D. Symposium: Classification of Leukemia. 1. The Classification of Acute Leukemia. Pathology 1982;14:277-81.
[3] Di Nardo CD, Cortes JE. Mutations in AML: Prognostic and Therapeutic Implications. Hematology Am Soc Hematol Educ Program 2016;2016:348-55.
[4] Cortes JE, Kantarjian HM. Acute Lymphoblastic Leukemia. A Comprehensive Review with Emphasis on Biology and Therapy. Cancer 1995;76:2393-417.
[5] Faderl S, Jeha S, Kantarjian HM. The Biology and Therapy of Adult Acute Lymphoblastic Leukemia. Cancer 2003;98:1337-54.
[6] Debela DT, Muzazu SG, Heraro KD, Ndalama MT, Mesele BW, Haile DC, et al. New Approaches and Procedures for Cancer Treatment: Current Perspectives. SAGE Open Med 2021;9:20503121211034366.
[7] Deak D, Gorcea-Andronic N, Sas V, Teodorescu P, Constantinescu C, Iluta S, et al. A Narrative Review of Central Nervous System Involvement in Acute Leukemias. Ann Transl Med 2021;9:68.
[8] Fullmer A, O’Brien S, Kantarjian H, Jabbour E. Novel Therapies for Relapsed Acute Lymphoblastic Leukemia. Curr Hematol Malig Rep 2009;4:148-56.
[9] Hamid AB, Petreaca RC. Secondary Resistant Mutations to Small Molecule Inhibitors in Cancer Cells. Cancers (Basel) 2020;12:927.
[10] Mansoori B, Mohammadi A, Davudian S, Shirjang S, Baradaran B. The Different Mechanisms of Cancer Drug Resistance: A Brief Review. Adv Pharm Bull 2017;7:339-48.
[11] Balasubramanian SK, Azmi AS, Maciejewski J. Selective Inhibition of Nuclear Export: A Promising Approach in the Shifting Treatment Paradigms for Hematological Neoplasms. Leukemia 2022;36:601-12.
[12] Fung HY, Chook YM. Atomic Basis of CRM1-cargo Recognition, Release and Inhibition. Semin Cancer Biol 2014;27:52-61.
[13] Emdal KB, Palacio-Escat N, Wigerup C, Eguchi A, Nilsson H, Bekker-Jensen DB, et al. Phosphoproteomics of Primary AML Patient Samples Reveals Rationale for AKT Combination Therapy and p53 Context to Overcome Selinexor Resistance. Cell Rep 2022;40:111177.
[14] Sellin M, Berg S, Hagen P, Zhang J. The Molecular Mechanism and Challenge of Targeting XPO1 in Treatment of Relapsed and Refractory Myeloma. Transl Oncol 2022;22:101448.
[15] Pinto V, Bergantim R, Caires HR, Seca H, Guimarães JE, Vasconcelos MH. Multiple Myeloma: Available Therapies and Causes of Drug Resistance. Cancers (Basel) 2020;12:407.
[16] Mo CC, Yee AJ, Midha S, Hartley-Brown MA, Nadeem O, O’Donnel EK, et al. Selinexor: Targeting a Novel Pathway in Multiple Myeloma. EJHaem 2023;4:792-810.
[17] Garzon R, Savona M, Baz R, Andreeff M, Gabrail N, Gutierrez M, et al. A Phase 1 Clinical Trial of Singleagent Selinexor in Acute Myeloid Leukemia. Blood 2017;129:3165-74.
[18] Sweet K, Komrokji R, Padron E, Cubitt CL, Turner JG, Zhou J, et al. Phase I Clinical Trial of Selinexor in Combination with Daunorubicin and Cytarabine in Previously Untreated Poor-risk Acute Myeloid Leukemia. Clin Cancer Res 2020;26:54-60.
[19] Fiedler W, Chromik J, Amberg S, Kebenko M, Thol F, Schlipfenbacher V, et al. A Phase II Study of Selinexor Plus Cytarabine and Idarubicin in Patients with Relapsed/ Refractory Acute Myeloid Leukaemia. Br J Haematol 2020;190:e169-73.
[20] Argueta C, Kashyap T, Klebanov B, Unger TJ, Guo C, Harrington S, et al. Selinexor Synergizes with Dexamethasone to Repress mTORC1 Signaling and Induce Multiple Myeloma Cell Death. Oncotarget 2018;9:25529-44.
[21] Long H, Hou Y, Li J, Song C, Ge Z. Azacitidine is Synergistically Lethal with XPO1 Inhibitor Selinexor in Acute Myeloid Leukemia by Targeting XPO1/eIF4E/cMYC Signaling. Int J Mol Sci 2023;24:6816.
[22] Zhao C, Yang ZY, Zhang J, Li O, Liu SL, Cai C, et al. Inhibition of XPO1 with KPT-330 Induces Autophagydependent Apoptosis in Gallbladder Cancer by Activating the p53/mTOR Pathway. J Transl Med 2022;20:434.
[23] Saxton RA, Sabatini DM. mTOR Signaling in Growth, Metabolism, and Disease. Cell 2017;168:960-76.
[24] Laplante M, Sabatini DM. mTOR Signaling at a Glance. J Cell Sci 2009;122:3589-94.
[25] Zoncu R, Efeyan A, Sabatini DM. mTOR: From Growth Signal Integration to Cancer, Diabetes and Ageing. Nat Rev Mol Cell Biol 2011;12:21-35.
[26] Linke M, Fritsch SD, Sukhbaatar N, Hengstschläger M, Weichhart T. mTORC1 and mTORC2 as Regulators of Cell Metabolism in Immunity. FEBS Lett 2017;591:3089-103.
[27] Fruman DA. mTOR Signaling: New Networks for ALL. Blood 2016;127:2658-9.
[28] Simioni C, Martelli AM, Zauli G, Melloni E, Neri LM. Targeting mTOR in Acute Lymphoblastic Leukemia. Cells 2019;8:190.
[29] Neri LM, Cani A, Martelli AM, Simioni C, Junghanss C, Tabellini G, et al. Targeting the PI3K/Akt/mTOR Signaling Pathway in B-precursor Acute Lymphoblastic Leukemia and its Therapeutic Potential. Leukemia 2014;28:739-48.
[30] Liberti MV, Locasale JW. The Warburg Effect: How does it Benefit Cancer Cells? Trends Biochem Sci 2016;41:211-8.
[31] Zheng J. Energy Metabolism of Cancer: Glycolysis Versus Oxidative Phosphorylation (Review). Oncol Lett 2012;4:1151-7.
[32] Hay N. Reprogramming Glucose Metabolism in Cancer: Can it be Exploited for Cancer Therapy? Nat Rev Cancer 2016;16:635-49.
[33] Fadaka A, Ajiboye B, Ojo O, Adewale O, Olayide I, Emuowhochere R. Biology of Glucose Metabolization in Cancer Cells. J Oncol Sci 2017;3:45-51.
[34] Szwed A, Kim E, Jacinto E. Regulation and Metabolic Functions of mTORC1 and mTORC2. Physiol Rev 2021;101:1371-426.
[35] Boag JM, Beesley AH, Firth MJ, Freitas JR, Ford J, Hoffmann K, et al. Altered Glucose Metabolism in Childhood Pre-B Acute Lymphoblastic Leukaemia. Leukemia 2006;20:1731-7.
[36] Chan LN, Chen Z, Braas D, Lee JW, Xiao G, Geng H, et al. Metabolic Gatekeeper Function of B-lymphoid Transcription Factors. Nature 2017;542:479-83.
[37] Arango NP, Yuca E, Zhao M, Evans KW, Scott S, Kim C, et al. Selinexor (KPT-330) Demonstrates Anti-tumor Efficacy in Preclinical Models of Triple-negative Breast Cancer. Breast Cancer Res 2017;19:93.
[38] Kulkoyluoglu-Cotul E, Smith BP, Wrobel K, Zhao YC, Chen KL, Hieronymi K, et al. Combined Targeting of Estrogen Receptor Alpha and XPO1 Prevent Akt Activation, Remodel Metabolic Pathways and Induce Autophagy to Overcome Tamoxifen Resistance. Cancers (Basel) 2019;11:479.
[39] Kawaguchi M, Aoki S, Hirao T, Morita M, Ito K. Autophagy is an Important Metabolic Pathway to Determine Leukemia Cell Survival Following Suppression of the Glycolytic Pathway. Biochem Biophys Res Commun 2016;474:188-92.
[40] Glick D, Barth S, Macleod KF. Autophagy: Cellular and Molecular Mechanisms. J Pathol 2010;221:3-12.
[41] Rosenfeldt MT, Ryan KM. The Multiple Roles of Autophagy in Cancer. Carcinogenesis 2011;32:955-63.
[42] Galluzzi L, Pietrocola F, Pedro JM, Amaravadi RK, Baehrecke EH, Cecconi F, et al. Autophagy in Malignant Transformation and Cancer Progression. EMBO J 2015;34:856-80.
[43] Yang ZJ, Chee CE, Huang S, Sinicrope FA. The Role of Autophagy in Cancer: Therapeutic Implications. Mol Cancer Ther 2011;10:1533-41.
[44] Chen N, Karantza V. Autophagy as a Therapeutic Target in Cancer. Cancer Biol Ther 2011;11:157-68.
[45] Sui X, Chen R, Wang Z, Huang Z, Kong N, Zhang M, et al. Autophagy and Chemotherapy Resistance: A Promising Therapeutic Target for Cancer Treatment. Cell Death Dis 2013;4:e838.
[46] Losa JH, Cobo CP, Viniegra JG, Lobo VJ, Cajal SR, Sánchez-Prieto R. Role of the p38 MAPK Pathway in Cisplatin-based Therapy. Oncogene 2003;22:3998-4006.
[47] Levy JM, Towers CG, Thorburn A. Targeting Autophagy in Cancer. Nat Rev Cancer 2017;17:528-42.
[48] Nilsen TW, Graveley BR. Expansion of the Eukaryotic Proteome by Alternative Splicing. Nature 2010;463:457-63.
[49] Wang Y, Liu J, Huang BO, Xu YM, Li J, Huang LF, et al. Mechanism of Alternative Splicing and its Regulation. Biomed Rep 2015;3:152-8.
[50] Urbanski LM, Leclair N, Anczuków O. Alternativesplicing Defects in Cancer: Splicing Regulators and their Downstream Targets, Guiding the Way to Novel Cancer Therapeutics. Wiley Interdiscip Rev RNA 2018;9:e1476.
[51] Anczukow O, Krainer AR. Splicing-factor Alterations in Cancers. RNA 2016;22:1285-301.
[52] Will CL, Lührmann R. Spliceosome Structure and Function. Cold Spring Harb Perspect Biol 2011;3:a003707.
[53] Fredericks AM, Cygan KJ, Brown BA, Fairbrother WG. RNA-binding Proteins: Splicing Factors and Disease. Biomolecules 2015;5:893-909.
[54] Zhao W, Li Y, Yao C, Zhang G, Zhao KY, Chen W, et al. Detection of Pathogenic Isoforms of IKZF1 in Leukemic Cell Lines and Acute Lymphoblastic Leukemia Samples: Identification of a Novel Truncated IKZF1 Transcript in SUP-B15. Cancers (Basel) 2020;12:3161.
[55] Lacobucci I, Lonetti A, Messa F, Cilloni D, Arruga F, Ottaviani E, et al. Expression of Spliced Oncogenic Ikaros Isoforms in Philadelphia-positive Acute Lymphoblastic Leukemia Patients Treated with Tyrosine Kinase Inhibitors: Implications for a New Mechanism of Resistance. Blood 2008;112:3847-55.
[56] Oh L, Hainaut P, Blanchet S, Ariffin H. Expression of p53 N-terminal Isoforms in B-cell Precursor Acute Lymphoblastic Leukemia and its Correlation with Clinicopathological Profiles. BMC Cancer 2020;20:110.
[57] De Necochea-Campion R, Shouse GP, Zhou Q, Mirshahidi S, Chen CS. Aberrant Splicing and Drug Resistance in AML. J Hematol Oncol 2016;9:85.
[58] Housman G, Byler S, Heerboth S, Lapinska K, Longacre M, Snyder N, et al. Drug Resistance in Cancer: An Overview. Cancers (Basel) 2014;6:1769-92.
[59] Siegfried Z, Karni R. The Role of Alternative Splicing in Cancer Drug Resistance. Curr Opin Genet Dev 2018;48:16-21.
[60] Jeromin S, Weissmann S, Haferlach C, Dicker F, Bayer K, Grossmann V, et al. SF3B1 Mutations Correlated to Cytogenetics and Mutations in NOTCH1, FBXW7, MYD88, XPO1 and TP53 in 1160 Untreated CLL Patients. Leukemia 2013;28:108-17.
[61] Taylor J, Mi X, Penson AV, Paffenholz SV, Alvarez K, Sigler A, et al. Safety and Activity of Selinexor in Patients with Myelodysplastic Syndromes or Oligoblastic Acute Myeloid Leukaemia Refractory to Hypomethylating Agents: A Single-centre, Single-arm, Phase 2 Trial. Lancet Haematol 2020;7:e566-74.
[62] Malakar P, Shilo A, Mogilevsky A, Stein I, Pikarsky E, Nevo Y, et al. Long Noncoding RNA MALAT1 Promotes Hepatocellular Carcinoma Development by SRSF1 Upregulation and mTOR Activation. Cancer Res 2017;77:1155-67.
[63] Ouimet M, Drouin S, Lajoie M, Caron M, St-Onge P, Gioia R,et al. AChildhood Acute Lymphoblastic Leukemia-specific lncRNA Implicated in Prednisolone Resistance, Cell Proliferation, and Migration. Oncotarget 2017;8:7477-88.
[64] Pouyanrad S, Rahgozar S, Ghodousi ES. Dysregulation of miR-335-3p, Targeted by NEAT1 and MALAT1 Long Non-coding RNAs, is Associated with Poor Prognosis in Childhood Acute Lymphoblastic Leukemia. Gene 2019;692:35-43.
[65] Chen W, Zhao W, Zhang L, Wang L, Wang J, Wan Z, et al. MALAT1-miR-101-SOX9 Feedback Loop Modulates the Chemo-resistance of Lung Cancer Cell to DDP via Wnt Signaling Pathway. Oncotarget 2017;8:94317-29.
[66] Chen W, Tan X, Yang Q, Fang Z, Xu Y. MALAT1 Enhances Gemcitabine Resistance in Non-small Cell Lung Cancer Cells by Directly Affecting miR-27a-5p/PBOV1 Axis. Cell Signal 2022;94:110326.
[67] Feng C, Zhao Y, Li Y, Zhang T, Ma Y, Liu Y. LncRNA MALAT1 Promotes Lung Cancer Proliferation and Gefitinib Resistance by Acting as a miR-200a Sponge. Arch Bronconeumol (Engl Ed) 2019;55:627-33.
[68] Fang Z, Chen W, Yuan Z, Liu X, Jiang H. LncRNAMALAT1 Contributes to the Cisplatin-resistance of Lung Cancer by Upregulating MRP1 and MDR1 via STAT3 Activation. Biomed Pharmacother 2018;101:536-42.
[69] Jiang Q, Liu S, Hou L, Guan Y, Yang S, Luo Z. The Implication of LncRNA MALAT1 in Promoting Chemoresistance of Laryngeal Squamous Cell Carcinoma Cells. J Clin Lab Anal 2020;34:e23116.
[70] Shi C, Ren S, Zhao X, Li Q. lncRNA MALAT1 Regulates the Resistance of Breast Cancer Cells to Paclitaxel via the miR-497-5p/SHOC2 Axis. Pharmacogenomics 2022;23:973-85.
[71] Huarte M, Guttman M, Feldser D, Garber M, Koziol MJ, Kenzelmann-Broz D, et al. A Large Intergenic Noncoding RNA Induced by p53 Mediates Global Gene Repression in the p53 Response. Cell 2010;142:409-19.
[72] Hung T, Wang Y, Lin MF, Koegel AK, Kotake Y, Grant GD, et al. Extensive and Coordinated Transcription of Noncoding RNAs within Cell-cycle Promoters. Nat Genet 2011;43:621-9.
[73] Schmitt AM, Garcia JT, Hung T, Flynn RA, Shen Y, Qu K, et al. An Inducible Long Noncoding RNA Amplifies DNA Damage Signaling. Nat Genet 2016;48:1370-6.
[74] Li XL, Subramanian M, Jones MF, Chaudhary R, Singh DK, Zong X, et al. Long Noncoding RNA PURPL Suppresses Basal p53 Levels and Promotes Tumorigenicity in Colorectal Cancer. Cell Rep 2017;20:2408-23.
[75] Casella G, Munk R, Kim KM, Piao Y, De S, Abdelmohsen K, et al. Transcriptome Signature of Cellular Senescence. Nucleic Acids Res 2019;47:7294-305.