Circulating extracellular RNAs, myocardial remodeling, and heart failure in patients with acute coronary syndrome
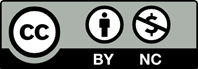
Background: Given high on-treatment mortality in heart failure (HF), identifying molecular pathways that underlie adverse cardiac remodeling may offer novel biomarkers and therapeutic avenues. Circulating extracellular RNAs (ex-RNAs) regulate important biological processes and are emerging as biomarkers of disease but less is known about their role in the acute setting, particularly in the setting of heart failure.
Methods: We examined the ex-RNA profiles of 296 acute coronary syndrome (ACS) survivors enrolled in the Transitions, Risks, and Actions in Coronary Events Center for Outcomes Research and Education (TRACE-CORE) Cohort. We measured 374 ex-RNAs selected a priori, based on previous findings from a large population study. We employed a two-step, mechanism-driven approach to identify ex-RNAs associated with echocardiographic phenotypes (left ventricular [LV] ejection fraction, LV mass, LV end-diastolic volume, left atrial dimension and left atrial volume index) then tested relations of these ex-RNAs with prevalent HF (N=31, 10.5%). We performed further bioinformatics analysis of microRNA predicted targets’ genes ontology categories and molecular pathways.
Results: We identified forty-four ex-RNAs associated with at least one echocardiographic phenotype associated with HF. Of these forty-four exRNAs, miR-29-3p, miR-584-5p, and miR-1247-5p were also associated with prevalent HF. The three microRNAs were implicated in the regulation p53 and TGF- signaling pathways and predicted to be involved in cardiac fibrosis and cell death; miRNA predicted targets were enriched in gene ontology categories including several involving the extracellular matrix and cellular differentiation.
Conclusions: Among ACS survivors, we observed that miR-29-3p, miR-584-5p, and miR-1247-5p were associated with both echocardiographic markers of cardiac remodeling and prevalent HF.
Relevance for patients: miR-29c-3p, miR-584-5p, and miR-1247-5p were associated with echocardiographic phenotypes and prevalent HF and are potential biomarkers for adverse cardiac remodeling in heart failure.
[1] Ziaeian B, Fonarow GC. Epidemiology and Aetiology of Heart Failure. Nat Rev Cardiol 2016;13:368-78.
[2] Mozaffarian D, Benjamin EJ, Go AS, Arnett DK, Blaha MJ, Cushman M, et al. Heart Disease and Stroke Statistics--2015 Update: A Report from the American Heart Association. Circulation 2015;131:e29-322.
[3] Voelkel NF, Quaife RA, Leinwand LA, Barst RJ, McGoon MD, Meldrum DR, et al. Right Ventricular Function and Failure: Report of aNational Heart, Lung, and Blood Institute Working Group on Cellular and Molecular Mechanisms of Right Heart Failure. Circulation 2006;114:1883-91.
[4] Mann DL, Bristow MR. Mechanisms and Models in Heart Failure: The Biomechanical Model and Beyond. Circulation 2005;111:2837-49.
[5] Tanai E, Frantz S. Pathophysiology of Heart Failure. ComprPhysiol 2015;6:187-214.
[6] Bartel DP. Metazoan MicroRNAs. Cell 2018;173:20-51.
[7] McManus DD, Ambros V. Circulating MicroRNAs in Cardiovascular Disease. Circulation 2011;124:1908-10.
[8] Freedman JE, Gerstein M, Mick E, Rozowsky J, Levy D, Kitchen R, et al. Corrigendum: Diverse Human Extracellular RNAs are widely Detected in Human Plasma. Nat Commun 2016;7:11902.
[9] TijsenAJ, Creemers EE, Moerland PD, de Windt LJ, van der Wal AC, Kok WE, et al. MiR423-5p as a Circulating Biomarker for Heart Failure. Circ Res 2010;106:1035-9.
[10] Scrutinio D, Conserva F, Passantino A, Iacoviello M, Lagioia R, Gesualdo L, et al. Circulating MicroRNA- 150-5p as a Novel Biomarker for Advanced Heart Failure: A Genome-wide Prospective Study. J Heart Lung Transplant 2017;36:616-24.
[11] Melman YF, Shah R, Danielson K, Xiao J, Simonson B, Barth A, et al. Circulating MicroRNA-30d is associated with Response to Cardiac Resynchronization Therapy in Heart Failure and Regulates Cardiomyocyte Apoptosis: A Translational Pilot Study. Circulation 2015;131:2202-16.
[12] Shah RV, Rong J, Larson MG, Yeri A, Ziegler O, TanriverdiK, et al. Associations of Circulating Extracellular RNAs with Myocardial Remodeling and Heart Failure. JAMA Cardiol 2018;3:871-6.
[13] Prastaro M, D’Amore C, Paolillo S, Losi M, Marciano C, PerrinoC, et al. Prognostic Role of Transthoracic Echocardiography in Patients affected by Heart Failure and Reduced Ejection Fraction. Heart Fail Rev 2015;20:305-16.
[14] Maurer MS, Koh WJ, Bartz TM, Vullaganti S, Barasch E, Gardin JM, et al. Relation of the Myocardial Contraction Fraction, as Calculated from M-mode Echocardiography, with Incident Heart Failure, Atherosclerotic Cardiovascular Disease and Mortality (Results from the Cardiovascular Health Study). Am J Cardiol 2017;119:923-8.
[15] Zoccola PM, Manigault AW, Figueroa WS, Hollenbeck C, MendleinA,WoodyA,etal.TraitRuminationPredictsElevated Evening Cortisol in Sexual and Gender Minority Young Adults. Int JEnviron Res Public Health 2017;14:E1365.
[16] Almahmoud MF, O’Neal WT, Qureshi W, Soliman EZ. Electrocardiographic Versus Echocardiographic Left Ventricular Hypertrophy in Prediction of Congestive Heart Failure in the Elderly. Clin Cardiol 2015;38:365-70.
[17] Carluccio E, Dini FL, Biagioli P, Lauciello R, Simioniuc A, Zuchi C, et al. The ‘Echo Heart Failure Score’: An Echocardiographic Risk Prediction Score of Mortality in Systolic Heart Failure. Eur J Heart Fail 2013;15:868-76.
[18] Waring ME, McManus RH,Saczynski JS, AnatchkovaMD, McManus DD, Devereaux RS, et al. Transitions, Risks, and Actions in Coronary Events-center for Outcomes Research and Education (TRACE-CORE): Design and Rationale. Circ Cardiovasc Qual Outcomes 2012;5:e44-50.
[19] McManus DD, Saczynski JS, Lessard D, Waring ME, Allison J, Parish DC, et al. Reliability of Predicting Early Hospital Readmission after Discharge for an Acute Coronary Syndrome using Claims-based Data. Am J Cardiol 2016;117:501-7.
[20] McManus DD, Lin H, Tanriverdi K, Quercio M, Yin X, Larson MG,et al. Relations between Circulating MicroRNAs and Atrial Fibrillation: Data from the Framingham Offspring Study. Heart Rhythm 2014;11:663-9.
[21] Lang RM, Badano LP, Mor-Avi V, Afilalo J, Armstrong A, Ernande L, et al. Recommendations for Cardiac Chamber Quantification by Echocardiography in Adults: An Update from the American Society of Echocardiography and the European Association of Cardiovascular Imaging. J Am Soc Echocardiogr 2015;28:1-3.e14.
[22] Devereux RB, Alonso DR, Lutas EM, Gottlieb GJ, Campo E, Sachs I, et al. Echocardiographic Assessment of Left Ventricular Hypertrophy: Comparison to Necropsy Findings. Am J Cardiol 1986;57:450-8.
[23] Wong N, Wang X. MiRDB: An Online Resource for MicroRNA Target Prediction and Functional Annotations. Nucleic Acids Res 2015;43:D146-52.
[24] Wang X. Improving MicroRNA Target Prediction by Modeling with Unambiguously identified MicroRNA- target Pairs from CLIP-ligation Studies. Bioinformatics 2016;32:1316-22.
[25] Subramanian A, Tamayo P, Mootha VK, Mukherjee S, Ebert BL, Gillette MA, et al. Gene Set Enrichment Analysis: A Knowledge-based Approach for Interpreting Genome- wide Expression Profiles. Proc Natl Acad Sci U S A 2005;102:15545-50.
[26] Krämer A, Green J, Pollard J Jr., Tugendreich S. Causal Analysis Approaches in Ingenuity Pathway Analysis. Bioinformatics 2014;30:523-30.
[27] van Rooij E, Sutherland LB, Thatcher JE, DiMaio JM, Naseem RH, Marshall WS, et al. Dysregulation of MicroRNAs after Myocardial Infarction Reveals a Role of miR-29 in Cardiac Fibrosis. Proc Natl Acad Sci U S A 2008;105:13027-32.
[28] Gaasch WH, Zile MR. Left Ventricular Structural Remodeling in Health and Disease: With Special Emphasis on Volume, Mass, and Geometry. J Am Coll Cardiol 2011;58:1733-40.
[29] VermaA, MerisA, SkaliH, Ghali JK,Arnold JM, BourgounM, et al.PrognosticImplicationsofLeftVentricularMassandGeometry following Myocardial Infarction: The VALIANT (VALsartan in Acute Myocardial iNfarcTion) Echocardiographic Study. JACC Cardiovasc Imaging 2008;1:582-91.
[30] Cohn JN, Ferrari R, Sharpe N. Cardiac Remodeling- concepts and Clinical Implications: A Consensus Paper from an International Forum on Cardiac Remodeling. Behalf of an International Forum on Cardiac Remodeling. JAm Coll Cardiol 2000;35:569-82.
[31] Birks EJ, Latif N, Enesa K, Folkvang T, Luong le A, Sarathchandra P, et al. Elevated p53 Expression is associated with Dysregulation of the Ubiquitin-proteasome System in Dilated Cardiomyopathy. Cardiovasc Res 2008;79:472-80.
[32] Crow MT, ManiK,Nam YJ, Kitsis RN. The Mitochondrial Death Pathway and Cardiac Myocyte Apoptosis. Circ Res 2004;95:957-70.
[33] Fujita T, Ishikawa Y. Apoptosis in Heart Failure. The Role of the Beta-adrenergic Receptor-mediated Signaling Pathway and p53-mediated Signaling Pathway in the Apoptosis of Cardiomyocytes. Circ J 2011;75:1811-8.
[34] Song H, Conte JV Jr., Foster AH, McLaughlin JS, Wei C. Increased p53 Protein Expression in Human Failing Myocardium. J Heart Lung Transplant 1999;18:744-9.
[35] Naito AT, Okada S, Minamino T, Iwanaga K, Liu ML, Sumida T, et al. Promotion of CHIP-mediated p53 Degradation Protects the Heart from Ischemic Injury. Circ Res 2010;106:1692-702.
[36] Zalzali H, Nasr B, Harajly M, Basma H, Ghamloush F, Ghayad S, et al. CDK2 Transcriptional Repression is an Essential Effector in p53-dependent Cellular Senescence- implications for Therapeutic Intervention. Mol Cancer Res 2015;13:29-40.
[37] Ito A, Kawaguchi Y, Lai CH, Kovacs JJ, Higashimoto Y, Appella E, et al. MDM2-HDAC1-mediated Deacetylation of p53 is required for its Degradation. EMBO J 2002;21:6236-45.
[38] Lagger G, Doetzlhofer A, Schuettengruber B,HaidwegerE, Simboeck E, Tischler J, et al. The Tumor Suppressor p53 and Histone Deacetylase 1 are Antagonistic Regulators of the Cyclin-dependent Kinase Inhibitor p21/WAF1/CIP1 Gene. Mol Cell Biol 2003;23:2669-79.
[39] Hutvágner G, McLachlan J, Pasquinelli AE, Bálint E, Tuschl T, Zamore PD, et al. A Cellular Function for the RNA-interference Enzyme Dicer in the Maturation of the Let-7 Small Temporal RNA. Science 2001;293:834-8.
[40] Chen JF, Murchison EP, Tang R, Callis TE, Tatsuguchi M, Deng Z, et al. Targeted Deletion of Dicer in the Heart Leads to Dilated Cardiomyopathy and Heart Failure. Proc Natl Acad Sci U SA 2008;105:2111-6.
[41] Border WA, Noble NA. Transforming Growth Factor Beta in Tissue Fibrosis. N Engl J Med 1994;331:1286-92.
[42] Khalil H, Kanisicak O, Prasad V, Correll RN, Fu X, Schips T, et al. Fibroblast-Specific TGF-β-smad2/3 Signaling Underlies Cardiac Fibrosis. J Clin Invest 2017;127:3770-83.
[43] Zeisberg EM, Tarnavski O, Zeisberg M, Dorfman AL, McMullen JR, Gustafsson E, et al. Endothelial-to- mesenchymal Transition Contributes to Cardiac Fibrosis. Nat Med 2007;13:952-61.
[44] Ayça B, Sahin I, Kucuk SH, Akin F, Kafadar D, Avşar M, et al. Increased Transforming Growth Factor-β Levels associated with Cardiac Adverse Events in Hypertrophic Cardiomyopathy. Clin Cardiol 2015;38:371-7.
[45] DobaczewskiM,Chen W, FrangogiannisNG. Transforming Growth Factor (TGF)-β Signaling in Cardiac Remodeling. J Mol Cell Cardiol 2011;51:600-6.
[46] Li JM, Brooks G. Differential Protein Expression and Subcellular Distribution of TGFbeta1, beta2 and beta3 in Cardiomyocytes during Pressure Overload-induced Hypertrophy. J Mol Cell Cardiol 1997;29:2213-24.
[47] Watabe T, Miyazono K. Roles of TGF-beta Family Signaling in Stem Cell Renewal and Differentiation. Cell Res 2009;19:103-15.
[48] Bhandary B, Meng Q, James J, Osinska H, Gulick J, Valiente-Alandi I, et al. Cardiac Fibrosis in Proteotoxic Cardiac Disease is Dependent upon Myofibroblast TGF-β Signaling. JAm Heart Assoc 2018;7:e010013.
[49] Wong LL, Armugam A, Sepramaniam S, Karolina DS, Lim KY, Lim JY, et al. Circulating MicroRNAs in Heart Failure with Reduced and Preserved Left Ventricular Ejection Fraction. Eur J Heart Fail 2015;17:393-404.
[50] Mick E, Shah R, Tanriverdi K, Murthy V, Gerstein M, Rozowsky J, et al. Stroke and Circulating Extracellular RNAs. Stroke 2017;48:828-34.