Planning and performing image-assisted robotic interventions using personalized, minimally invasive, safe, and precise therapeutics
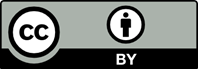
This review aims to analyze complex medical interventions planned and performed using image-guided robots. Such interventions, which may involve surgical or targeted drug delivery, are minimally invasive, precise, and safe therapies. The accuracy of robotic positioning is improved by reducing uncertainty and complexity, which can be achieved by matching real and virtual interventional procedures involving physical and virtual phantoms of the relevant part of the corresponding living tissues. Such tailored training includes personalized, patient, and interventional tool characteristics, and the results enable a real (with patient) intervention controlled by staff and a possible matched autonomous intervention under staff supervision. This paper discusses considerations for selecting appropriate scanners to control and monitor image-guided interventional procedures, planning personalized medical interventions using physical and virtual phantoms, involving staff in the loop, and employing augmented matched digital twins (DTs) for real interventions. Moreover, the paper positions the image-assisted robotic strategy in comparison to laparoscopic surgery. Each topic covered in this article, while self-contained, is supported by examples from the literature to facilitate a deeper understanding. The outcomes of this review highlight the importance of complex medical interventions involving image-assisted robotics or laparoscopic processes involving minimally invasive, nonionizing, and precise interventions. Furthermore, DTs already integrated into healthcare, combined with digital tools, could offer an effective solution for managing image-assisted robotics. This includes planning interventions with phantoms or patients and involving staff in the loop.
- Chinzei K, Hata N, Jolesz FA, Kikinis R. Surgical Assist Robot for the Active Navigation in the Intraoperative MRI: Hardware Design Issues. In: Proceedings of the 2000 IEEE/ RSJ International Conference on Intelligent Robots and Systems (IROS 2000) (Cat. No.00CH37113), Takamatsu, Japan; 2000. p. 727-732. doi: 10.1109/IROS.2000.894690
- Tsekos NV, Khanicheh A, Christoforou E, Mavroidis C. Magnetic resonance-compatible robotic and mechatronics systems for image- guided interventions and rehabilitation: A review study. Annu Rev Biomed Eng. 2007;9:351-387. doi: 10.1146/annurev.bioeng.9.121806.160642
- Razek A. Towards an image-guided restricted drug release in friendly implanted therapeutics. Eur Phys J Appl Phys. 2018;82:31401. doi: 10.1051/epjap/2018180201
- Razek A. Image-guided surgical and pharmacotherapeutic routines as part of diligent medical treatment. Appl Sci. 2023;13:13039. doi: 10.3390/app132413039
- Marrelli D, Piccioni SA, Carbone L, et al. Posterior and para-aortic (D2plus) lymphadenectomy after neoadjuvant/ conversion therapy for locally advanced/oligometastatic gastric cancer. Cancers (Basel). 2024;16(7):1376. doi: 10.3390/cancers16071376
- Faoro G, Maglio S, Pane S, Iacovacci V, Menciassi A. An artificial intelligence-aided robotic platform for ultrasound-guided transcarotid revascularization. IEEE Robot Autom Lett. 2023;8:2349-2356. doi: 10.1109/LRA.2023.3251844
- Su H, Kwok KW, Cleary K, et al. State of the art and future opportunities in MRI-guided robot-assisted surgery and interventions. Proc IEEE Inst Electr Electron Eng. 2022;110:968-992. doi: 10.1109/jproc.2022.3169146
- Padhan J, Tsekos N, Al-Ansari A, et al. Dynamic Guidance Virtual Fixtures for Guiding Robotic Interventions: Intraoperative MRI-guided Transapical Cardiac Intervention Paradigm. In: Proceedings of the 2022 IEEE 22nd International Conference on Bioinformatics and Bioengineering (BIBE), Taichung, Taiwan; 2022. p. 265-270. doi: 10.1109/BIBE55377.2022.00062
- Singh S, Torrealdea F, Bandula S. MR imaging-guided intervention: Evaluation of MR conditional biopsy and ablation needle tip artifacts at 3T using a balanced fast field echo sequence. J Vasc Interv Radiol. 2021;32:1068-1074. doi: 10.1016/j.jvir.2021.03.536
- Xu L, Pacia CP, Gong Y, et al. Characterization of the targeting accuracy of a neuronavigation-guided transcranial FUS system in vitro, in vivo, and in silico. IEEE Trans Biomed Eng. 2023;70(5):1528-1538. doi: 10.1109/TBME.2022.3221887
- Navarro-Becerra JA, Borden MA. Targeted microbubbles for drug, gene, and cell delivery in therapy and immunotherapy. Pharmaceutics. 2023;15(6):1625. doi: 10.3390/pharmaceutics15061625
- Delaney LJ, Isguven S, Eisenbrey JR, et al. Making waves: How ultrasound-targeted drug delivery is changing pharmaceutical approaches. Mater Adv. 2022;3:3023-3040. doi: 10.1039/d1ma01197a
- Razek A. Augmented therapeutic tutoring in diligent image-assisted robotic interventions. AIMS Med Sci. 2024;11(2):210-219. doi: 10.3934/medsci.2024016
- Zhang B, Liu L, Meng D, Kue CS. Medical imaging technology: Principles and systems. INNOSC Theranostics Pharmacol Sci. 2024;7(3):3360. doi: 10.36922/itps.3360
- Kuah T, Vellayappan BA, Makmur A, et al. State-of-the-art imaging techniques in metastatic spinal cord compression. Cancers (Basel). 2022;14:3289. doi: 10.3390/cancers14133289
- Kraus MS, Coblentz AC, Deshpande VS, et al. State-of-the-art magnetic resonance imaging sequences for pediatric body imaging. Pediatr Radiol. 2023;53:1285-1299. doi: 10.1007/s00247-022-05528-y
- Sennimalai K, Selvaraj M, Kharbanda OP, et al. MRI-based cephalometrics: A scoping review of current insights and future perspectives. Dentomaxillofac Radiol. 2023;52:20230024. doi: 10.1259/dmfr.20230024
- Chianca V, Vincenzo B, Cuocolo R, et al. MRI quantitative evaluation of muscle fatty infiltration. Magnetochemistry. 2023;9:111. doi: 10.3390/magnetochemistry9040111
- Sato Y, Takeuchi T, Fuju A, et al. MRI safety for leave-on powdered hair thickeners under 1.5-T and 3.0-T MRI: Measurement of deflection force, MRI artifact, and evaluation of preexamination screening. Phys Eng Sci Med. 2023;46:915-924. doi: 10.1007/s13246-023-01267-y
- Akdogan G, Istanbullu O. Analysing the effects of metallic biomaterial design and imaging sequences on MRI interpretation challenges due to image artefacts. Phys Eng Sci Med. 2022;45:1163-1174. doi: 10.1007/s13246-022-01183-7
- Germann C, Nanz D, Sutter R. Magnetic resonance imaging around metal at 1.5 Tesla: Techniques from basic to advanced and clinical impact. Investig Radiol. 2021;56:734-748. doi: 10.1097/RLI.0000000000000798
- Germann C, Falkowski AL, von Deuster C, et al. Basic and advanced metal-artifact reduction techniques at ultra-high field 7-T magnetic resonance imaging-phantom study investigating feasibility and efficacy. Investig Radiol. 2022;57:387-398. doi: 10.1097/RLI.0000000000000850
- Inaoka T, Kitamura N, Sugeta M, et al. Diagnostic value of advanced metal artifact reduction magnetic resonance imaging for periprosthetic joint infection. J Comput Assist Tomogr. 2022;46:455-463. doi: 10.1097/RCT.0000000000001297
- Haskell MW, Nielsen JF, Noll DC. Off-resonance artifact correction for MRI: A review. NMR Biomed. 2023;36:e4867. doi: 10.1002/nbm.4867
- Spronk T, Kraff O, Kreutner J, et al. Development and evaluation of a numerical simulation approach to predict metal artifacts from passive implants in MRI. MAGMA. 2022;35:485-497. doi: 10.1007/s10334-021-00966-5
- Jia X, Zhang Y, Du H, Yu Y. Experimental study of double cable-conduit driving device for MRI compatible biopsy robots. J Mech Med Biol. 2021;21:2140014. doi: 10.1142/S0219519421400145
- Li X, Young AS, Raman SS, et al. Automatic needle tracking using Mask R-CNN for MRI-guided percutaneous interventions. Int J Comput Assist Radiol Surg. 2020;15:1673-1684. doi: 10.1007/s11548-020-02226-8
- Wartenberg M, Schornak J, Carvalho P, et al. Closed-loop Autonomous Needle Steering during Cooperatively Controlled Needle Insertions for MRI-guided Pelvic Interventions. In: The 10th Hamlyn Symposium on Medical Robotics, 33--34, Imperial College. London, UK; 2017.
- Wu D, Li G, Patel N, et al. Remotely Actuated Needle Driving Device for MRI-Guided Percutaneous Interventions: Force and Accuracy Evaluation. In: Proceedings of the 2019 41st Annual International Conference of the IEEE Engineering in Medicine & Biology Society (EMBC), Berlin, Germany; 2019. doi: 10.1109/ismr.2019.8710176
- Mohith S, Upadhya AR, Navin KP, et al. Recent trends in piezoelectric actuators for precision motion and their applications: A review. Smart Mater Struct. 2020;30:013002. doi: 10.1088/1361-665X/abc6b9
- Gao X, Yang J, Wu J, et al. Piezoelectric actuators and motors: Materials, designs, and applications. Adv Mater Technol. 2020;5:1900716. doi: 10.1002/admt.201900716
- Qiao G, Li H, Lu X, Wen J, Cheng T. Piezoelectric stick-slip actuators with flexure hinge mechanisms: A review. J Intell Mater Syst Struct. 2022;33:1879-1901. doi: 10.1177/1045389X211072244
- Liu J, Gao X, Jin H, et al. Miniaturized electromechanical devices with multi-vibration modes achieved by orderly stacked structure with piezoelectric strain units. Nat Commun. 2022;13:6567. doi: 10.1038/s41467-022-34231-7
- Fu DK, Fan PQ, Yuan T, Wang YS. A novel hybrid mode linear ultrasonic motor with double driving feet. Rev Sci Instrum. 2022;93:025003. doi: 10.1063/5.0057254
- Li Z, Guo Z, Han H, Su Z, Sun H. Design and characteristic analysis of multi-degree-of-freedom ultrasonic motor based on spherical stator. Rev Sci Instrum. 2022;93:025004. doi: 10.1063/5.0074049
- Wang S, Zhou S, Zhang X, et al. Bionic stepping motors driven by piezoelectric materials. J Bionic Eng. 2023;20:858-872. doi: 10.1007/s42235-022-00313-x
- Hernandez C, Bernard Y, Razek A. Design and manufacturing of a piezoelectric traveling-wave pumping device. IEEE Trans Ultrason Ferroelectr Freq Control. 2013;60:1949-1956. doi: 10.1109/TUFFC.2013.2779
- Zhang SJ, Liu Y, Deng J, et al. Piezo robotic hand for motion manipulation from micro to macro. Nat Commun. 2023;14:500. doi: 10.1038/s41467-023-36243-3
- Yang Z, Li X, Tang J, et al. A bionic stick-slip piezo-driven positioning platform designed by imitating the structure and movement of the crab. J Bionic Eng. 2023;20:2590-2600. doi: 10.1007/s42235-023-00411-4
- Traverson M, Heiden M, Stanciu LA, et al. In vivo evaluation of biodegradability and biocompatibility of Fe30Mn alloy. Vet Comp Orthop Traumatol. 2018;31:10-16. doi: 10.3415/VCOT-17-06-0080
- Wang Y, Venezuela J, Dargusch M. Biodegradable shape memory alloys: Progress and prospects. Biomaterials. 2021;279:121215. doi: 10.1016/j.biomaterials.2021.121215
- Li H, Lin G, Wang P, Huang J, Wen C. Nutrient alloying elements in biodegradable metals: A review. J Mater Chem B. 2021;9:9806-9825. doi: 10.1039/D1TB01962G
- Rabeeh VPM, Hanas T. Progress in manufacturing and processing of degradable Fe-based implants: A review. Prog Biomater. 2022;11:163-191. doi: 10.1007/s40204-022-00189-4
- Babacan N, Kochta F, Hoffmann V, et al. Effect of silver additions on the microstructure, mechanical properties and corrosion behavior of biodegradable Fe-30Mn-6Si. Mater Today Commun. 2021;28:102689. doi: 10.1016/j.mtcomm.2021.102689
- Tai CC, Lo HL, Liaw CK, Huang, et al. Biocompatibility and biological performance evaluation of additive-manufactured bioabsorbable iron-based porous suture anchor in a rabbit model. Int J Mol Sci. 2021;22:7368. doi: 10.3390/ijms22147368
- Bakhsheshi-Rad HR, Najafinezhad A, Hadisi Z, et al. Characterization and biological properties of nanostructured clinoenstatite scaffolds for bone tissue engineering applications. Mater Chem Phys. 2021;259:123969. doi: 10.1016/j.matchemphys.2020.123969
- Sun Y, Chen L, Liu N, et al. Laser-modified fe–30 mn surfaces with promoted biodegradability and biocompatibility toward biological applications. J Mater Sci. 2021;56:13772-13784. doi: 10.1007/s10853-021-06139-y
- Hao S, Yang T, Zhang A, et al. Evaluation of biodegradable alloy Fe30Mn0.6N in rabbit femur and cartilage through detecting osteogenesis and autophagy. Biomed Res Int. 2023;18:3626776. doi: 10.1155/2023/3626776
- Biffi CA, Fiocchi J, Bregoli C, et al. Ultrashort laser texturing for tuning surface morphology and degradation behavior of the biodegradable Fe-20Mn alloy for temporary implants. Adv Eng Mater. 2022;24:2101496. doi: 10.1002/adem.202101496
- Putra NE, Leeflang MA, Taheri P, et al. Extrusion-based 3D printing of ex situ-alloyed highly biodegradable MRI-friendly porous iron-manganese scaffolds. Acta Biomater. 2021;134:774-790. doi: 10.1016/j.actbio.2021.07.042
- Williams RP, Karzova MM, Yuldashev PV, et al. Dual-mode 1-D linear ultrasound array for image-guided drug delivery enhancement without ultrasound contrast agents. IEEE Trans Ultrason Ferro Freq Cont. 2023;70:693-707. doi: 10.1109/TUFFC.2023.3268603
- Williams RP, Karzova MM, Yuldashev PV, et al. Microbubble-mediated ultrasound drug-delivery and therapeutic monitoring. Expert Opin Drug Deliv. 2017;14:1031-1043. doi: 10.1080/17425247.2017.1266328
- Nazary Abrbekoh F, Salimi L, Saghati S, et al. Application of microneedle patches for drug delivery, doorstep to novel therapies. J Tissue Eng. 2022;13:20417314221085390. doi: 10.1177/20417314221085390
- Arno MC, Simpson JD, Blackman LD, et al. Enhanced drug delivery to cancer cells through a pH-sensitive polycarbonate platform. Biomater Sci. 2023;11:908-915. doi: 10.1039/D2BM01626E
- Chen W, Cheng CA, Zink JI. Spatial, temporal, and dose control of drug delivery using noninvasive magnetic stimulation. ACS Nano. 2019;13:1292-1308. doi: 10.1021/acsnano.8b06655
- Huang J, Xiao K. Nanoparticles-based strategies to improve the delivery of therapeutic small interfering RNA in precision oncology. Pharmaceutics. 2022;14:1586. doi: 10.3390/pharmaceutics14081586
- Jia M, Zhang D, Zhang C, Li C. Nanoparticle-based delivery systems modulate the tumor microenvironment in pancreatic cancer for enhanced therapy. J Nanobiotechnol. 2021;19:384. doi: 10.1186/s12951-021-01134-6
- Zhao JF, Zou FL, Zhu JF, et al. Nano-drug delivery system for pancreatic cancer: A visualization and bibliometric analysis. Front Pharmacol. 2022;13:1025618. doi: 10.3389/fphar.2022.1025618
- Tao F, Sui F, Liu A, et al. Digital twin-driven product design framework. Int J Prod Res. 2019;57:3935-3953. doi: 10.1080/00207543.2018.1443229
- Fuller A, Fan Z, Day C, Barlow C. Digital twin: Enabling technologies, challenges and open research. IEEE Access. 2020;8:108952-108971. doi: 10.1109/ACCESS.2020.2998358
- Grieves M, Vickers J. Digital twin: Mitigating unpredictable, undesirable emergent behavior in complex systems. In: Trans-disciplinary Perspectives on Complex Systems. Cham, Switzerland: Springer; 2017. p. 85-113. doi: 10.1007/978-3-319-38756-7_4
- Razek A. Monitoring complexity in clean energy systems applications. Clean Energy Sustain. 2024;2:10007. doi: 10.35534/ces.2024.10007
- Sun T, He X, Li Z. Digital twin in healthcare: Recent updates and challenges. Digit Health. 2023;9:20552076221149651. doi: 10.1177/20552076221149651
- Sun T, He X, Song X, et al. The digital twin in medicine: A key to the future of healthcare? Front Med. 2022;9:907066. doi: 10.3389/fmed.2022.907066
- De Benedictis A, Mazzocca N, Somma A, Strigaroet C. Digital twins in healthcare: An architectural proposal and its application in a social distancing case study. IEEE J Biomed Health Inform. 2022;27:5143-5154. doi: 10.1109/JBHI.2022.3205506.
- Haleem A, Javaid M, Singh RP, Suman R. Exploring the revolution in healthcare systems through the applications of digital twin technology. Biomed Technol. 2023;4:28-38. doi: 10.1016/j.bmt.2023.02.001
- Mohamed N, Al-Jaroodi J, Jawhar I, Kesserwan N. Leveraging digital twins for healthcare systems engineering. IEEE Access. 2023;11:69841-69853. doi: 10.1109/ACCESS.2023.3292119
- Mohamed N, Al-Jaroodi J, Jawhar I, Kesserwan N. How Healthcare Systems Engineering Can Benefit from Digital Twins? In: Proceedings of the 2023 IEEE International Systems Conference (SysCon), Vancouver, BC, Canada; 2023. p. 1-6. doi: 10.1109/SysCon53073.2023.10131101
- Ricci A, Croatti A, Montagna S. Pervasive and connected digital twins-a vision for digital health. IEEE Internet Comput. 2022;26:26-32. doi: 10.1109/MIC.2021.3052039
- Okegbile SD, Cai J. Edge-Assisted Human-to-Virtual Twin Connectivity Scheme for Human Digital Twin Frameworks. In: Proceedings of the 2022 IEEE 95th Vehicular Technology Conference: (VTC2022-Spring), Helsinki, Finland; 2022. p. 1-6. doi: 10.1109/VTC2022-Spring54318.2022.9860619
- Das C, Mumu AA, Ali MF, et al. Toward IoRT collaborative digital twin technology enabled future surgical sector: Technical innovations, opportunities and challenges. IEEE Access. 2022;10:129079-129104. doi: 10.1109/ACCESS.2022.3227644
- Carbonaro A, Marfoglia A, Nardini F, Mellone S. CONNECTED: Leveraging digital twins and personal knowledge graphs in healthcare digitalization. Front Digit Health. 2023;5:1322428. doi: 10.3389/fdgth.2023.1322428
- Strobel G, Möller F, van der Valk H. Healthcare in the Era of Digital Twins: Towards a Domain-Specific Taxonomy. Technical Report Healthcare IT, European Conference on Information Systems; 2022. Available from: https://api. semanticscholar.org/CorpusID:251034187 [Last accessed on 2024 Jul 01].
- Humphrey JD. Biological soft tissues. In: Sharpe W, editor. Springer Handbook of Experimental Solid Mechanics. Springer Handbooks. Boston, MA., USA: Springer; 2008. doi: 10.1007/978-0-387-30877-7_7
- Kallin S. Deformation of Human Soft Tissues: Experimental and numerical aspects. Licentiate Thesis, Jönköping University, School of Health and Welfare, HHJ, Department of Rehabilitation; 2019. Available from: https://hj.diva-portal.org/smash/get/diva2:1344790/FULLTEXT01.pdf [Last accessed on 2024 Mar 17].
- Al-Dirini RMA, Reed MP, Hu JW, Thewlis D. Development and validation of a high anatomical fidelity FE model for the buttock and thigh of a seated individual. Ann Biomed Eng. 2016;44(9):2805-2816. doi: 10.1007/s10439-016-1560-3
- Fung YC. Biomechanics: Mechanical Properties of Living Tissues. 2nd ed. New York, Berlin: Springer-Vlg; 1993. doi: 10.1007/978-1-4757-2257-4
- Henninger HB, Reese SP, Anderson AE, Weiss JA. Validation of computational models in biomechanics. Proc Inst Mechan Eng H. 2010;224(7):801-881. doi: 10.1243/09544119JEIM649
- Gabriel S, Lau RW, Gabriel C. The dielectric properties of biological tissues: II. measurements in the frequency range 10 Hz to 20 GHz. Phys Med Biol. 1996;41:2251-2269. doi: 10.1088/0031-9155/41/11/002
- Barchanski A, Steiner T, De Gersem H, Clemens M, Weiland T. Local grid refinement for low-frequency current computations in 3-D human anatomy models. IEEE Trans Magn. 2006;42:1371-1374. doi: 10.1109/TMAG.2006.871449
- Noetscher GM. The CAD-Compatible VHP-Male Computational Phantom. In: Makarov SN, Noetscher GM, Nummenmaa A, editors. Brain and Human Body Modeling 2020: Computational Human Models Presented at EMBC 2019 and the BRAIN Initiative® 2019 Meeting. Cham, Switzerland: Springer; 2020. p. 309-323. doi: 10.1007/978-3-030-45623-8_19
- Makarov SN, Noetscher GM, Yanamadala J, et al. Virtual human models for electromagnetic studies and their applications. IEEE Rev Biomed Eng. 2017;10:95-121. doi: 10.1109/RBME.2017.2722420
- Song Y. Human digital twin, the development and impact on design. J Comput Inf Sci Eng. 2023;23:060819. doi: 10.1115/1.4063132
- Burattini S, Montagna S, Croatti A, et al. An Ecosystem of Digital Twins for Operating Room Management. In: Proceedings of the 2023 IEEE 36th International Symposium on Computer-Based Medical Systems (CBMS), L’Aquila, Italy; 2023. p. 770-775. doi: 10.1109/CBMS58004.2023.00317
- Hagmann K, Hellings-Kuß A, Klodmann J, et al. A digital twin approach for contextual assistance for surgeons during surgical robotics training. Front Robot AI. 2021;8:735566. doi: 10.3389/frobt.2021.735566
- Katsoulakis E, Wang Q, Wu H, et al. Digital twins for health: a scoping review. NPJ Digit Med. 2024;7:77. doi: 10.1038/s41746-024-01073-0
- Cusumano D, Boldrini L, Dhont J, et al. Artificial intelligence in magnetic resonance guided radiotherapy: Medical and physical considerations on state of art and future perspectives. Phys Med. 2021;85:175-191. doi: 10.1016/j.ejmp.2021.05.010
- Seetohul J, Shafiee M, Sirlantzis K. Augmented reality (AR) for surgical robotic and autonomous systems: State of the art, challenges, and solutions. Sensors (Basel). 2023;23:6202. doi: 10.3390/s23136202
- Avrumova F, Lebl DR. Augmented reality for minimally invasive spinal surgery. Front Surg. 2023;9:1086988. doi: 10.3389/fsurg.2022.1086988
- Long Y, Cao J, Deguet A, Taylor RH, Dou Q. Integrating Artificial Intelligence and Augmented Reality in Robotic Surgery: An Initial dVRK Study Using a Surgical Education Scenario. In: Proceedings of the International Symposium on Medical Robotics (ISMR), Atlanta, GA, USA; 2022. p. 1-8.
- Fu J, Rota A, Li S, et al. Recent advancements in augmented reality for robotic applications: A survey. Actuators. 2023;12:323. doi: 10.3390/act12080323
- Qian L, Wu JY, DiMaio SP, et al. A review of augmented reality in robotic-assisted surgery. IEEE Trans Med Robot Bionics. 2020;2:1-16. doi: 10.1109/TMRB.2019.2957061
- Razek A. Matching of an observed event and its virtual model in relation to smart theories, coupled models and supervision of complex procedures-A review. Comptes Rendus Phys. 2024;25:1-16. doi: 10.5802/crphys.184
- Nunes AS, Dular P, Chadebec O, Kuo-Peng P. Subproblems applied to a 3-D magnetostatic facet FEM formulation. IEEE Trans Magnet. 2018;54(8):7402209. doi: 10.1109/TMAG.2018.2828786
- Cicuttin M, Geuzaine C. Numerical investigation of a 3D hybrid high-order method for the indefinite time-harmonic Maxwell problem. Finite Elements Anal Des. 2024;233:104124. doi: 10.1016/j.finel.2024.104124
- Piriou F, Razek A. Numerical simulation of a nonconventional alternator connected to a rectifier. IEEE Trans Energy Convers. 1990;5:512-518. doi: 10.1109/60.105275
- Bernard L. Electrical Characterization of Biological Tissues and Computing of Phenomena Induced in the Human Body by Electromagnetic Fields Below 1 GHz. PhD Thesis, Universities of Ecole Centrale de Lyon, France and Universidade federal de Minas Gerais, Brazil; 2007. Available from: https://theses.hal.science/tel-00179791v3 [Last accessed on 2024 Nov 20].
- Ren Z, Razek A. Force calculation by Maxwell stress tensor in 3D hybrid finite element-boundary integral formulation. IEEE Trans Magnet. 1990;26(5):2774-2776. doi: 10.1109/20.104869
- Freschi F, Giaccone L, Cirimele V, Canova A. Numerical assessment of low-frequency dosimetry from sampled magnetic fields. Phys Med Biol. 2018;63(1):015029. doi: 10.1088/1361-6560/aa9915
- Li C, Ren Z, Razek A. An approach to adaptive mesh refinement for three-dimensional eddy-current computations. IEEE Trans Magn. 1994;30:113-17. doi: 10.1109/20.272523
- Piriou F, Razek A. Calculation of saturated inductances for numerical simulation of synchronous machines. IEEE Trans Magn. 1983;19(6):2628-2631. doi: 10.1109/TMAG.1983
- Madani SS, Schaltz E, Kær SK. Thermal analysis of cold plate with different configurations for thermal management of a lithium-ion battery. Batteries. 2020;6:17. doi: 10.3390/batteries6010017
- Urdaneta-Calzadilla A, Galopin N, Niyonzima I, et al. FEM-BEM modeling of nonlinear magnetoelectric effects in heterogeneous composite structures. IEEE Trans Magn. 2024;60(3):7401704. doi: 10.1109/TMAG.2023.3339088
- Razek A. Coupled models in electromagnetic and energy conversion systems from smart theories paradigm to that of complex events: A review. Appl Sci. 2022;12:4675. doi: 10.3390/app12094675
- Razek A. Strategies for managing models regarding environmental confidence and complexity involved in intelligent control of energy systems - A review. Adv Environ Energies. 2023;2(1):aee020104. doi: 10.58396/aee020104
- Kudela J, Matousek R. Recent advances and applications of surrogate models for finite element method computations: A review. Soft Comput. 2022;26:13709-13733. doi: 10.1007/s00500-022-07362-8
- Koziel S, Pietrenko-Dabrowska A. Fundamentals of Data-driven Surrogate Modeling. Europe: World Scientific Publishing Europe Ltd.; 2022. p. 1-37. doi: 10.1142/9781800610750 0001
- Lebensztajn L, Marretto CAR, Caldora Costa M, Coulomb JL. Kriging: A useful tool for electromagnetic device optimization. IEEE Trans Magn. 2004;40(2):1196-1199. doi: 10.1109/TMAG.2004.824542
- Gaignaire R, Scorretti R, Sabariego RV, Geuzaine C. Stochastic uncertainty quantification of eddy currents in the human body by polynomial chaos decomposition. IEEE Trans Magn. 2012;48(2):451-454. doi: 10.1109/TMAG.2011.2171925
- Bates HW. Contributions to an insect fauna of the amazon valley. Lepidoptera: Heliconidae. Trans Linnean Soc London. 1862;23(3):495-566. doi: 10.1111/j.1096-3642.1860.tb00146.x