Criteria for developing active cellular targeting miRNA oligonucleotide therapeutics with a peptide nucleic acid backbone: Combating cardiometabolic pandemics
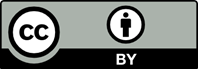
Oligonucleotide therapeutics (ONTs) represent a growing new class of therapeutic agents aimed at addressing chronic diseases that remain untreatable by small molecules and antibodies. Our goal was to establish a selection of several criteria to design and develop miRNA-based ONTs, focusing on improved chemistry, pharmacokinetics/pharmacodynamics (PK/PD) profiles, and safety characteristics to combat cardiometabolic pandemics. By leveraging our own experimental data obtained from experiments involving miR-22-3p antagomirs and a careful review of the literature, we established a set of seven criteria to optimize the design of miRNA ONTs. These criteria prioritize simplified drug synthesis, optimized PK/PD properties, and reduced potential toxicities. This proposed set of seven criteria represents a novel strategy for developing active cellular targeting miRNA ONTs for various therapeutic indications.
- Fire A, Xu S, Montgomery MK, Kostas SA, Driver SE, Mello CC. Potent and specific genetic interference by double-stranded RNA in Caenorhabditis elegans. Nature. 1998;391(6669):806-811. doi: 10.1038/35888
- Seyhan AA. Trials and tribulations of MicroRNA therapeutics. Int J Mol Sci. 2024;25(3):1469. doi: 10.3390/ijms25031469
- Hofman CR, Corey DR. Targeting RNA with synthetic oligonucleotides: Clinical success invites new challenges. Cell Chem Biol. 2024;31(1):125-138. doi: 10.1016/j.chembiol.2023.09.005
- Nappi F. Non-coding RNA-targeted therapy: A state-of-the-art review. Int J Mol Sci. 2024;25(7):3630. doi: 10.3390/ijms25073630
- Dixon SJ, Stockwell BR. Identifying druggable disease-modifying gene products. Curr Opin Chem Biol. 2009;13(5- 6):549-555. doi: 10.1016/j.cbpa.2009.08.003
- Takakusa H, Iwazaki N, Nishikawa M, Yoshida T, Obika S, Inoue T. Drug metabolism and pharmacokinetics of antisense oligonucleotide therapeutics: Typical profiles, evaluation approaches, and points to consider compared with small molecule drugs. Nucleic Acid Ther. 2023;33(2):83-94. doi: 10.1089/nat.2022.0054
- Ranasinghe P, Addison ML, Dear JW, Webb DJ. Small interfering RNA: Discovery, pharmacology and clinical development-An introductory review. Br J Pharmacol. 2023;180(21):2697-2720. doi: 10.1111/bph.15972
- Friedrich M, Aigner A. Therapeutic siRNA: State-of-the-art and future perspectives. BioDrugs. 2022;36(5):549-571. doi: 10.1007/s40259-022-00549-3
- Egli M, Manoharan M. Chemistry, structure and function of approved oligonucleotide therapeutics. Nucleic Acids Res. 2023;51(6):2529-2573. doi: 10.1093/nar/gkad067
- Kulkarni JA, Witzigmann D, Thomson SB, et al. The current landscape of nucleic acid therapeutics. Nat Nanotechnol. 2021;16(6):630-643. doi: 10.1038/s41565-021-00898-0
- Al Shaer D, Al Musaimi O, Albericio F, de la Torre BG. 2023 FDA TIDES (peptides and oligonucleotides) harvest. Pharmaceuticals (Basel). 2024;17(2):243. doi: 10.3390/ph17020243
- De la Torre BG, Albericio F. The pharmaceutical industry in 2023: An analysis of FDA drug approvals from the perspective of molecules. Molecules. 2024;29(3):585. doi: 10.3390/molecules29030585
- Anwar S, Mir F, Yokota T. Enhancing the effectiveness of oligonucleotide therapeutics using Cell-penetrating peptide conjugation, chemical modification, and carrier-based delivery strategies. Pharmaceutics. 2023;15(4):1130. doi: 10.3390/pharmaceutics15041130
- Khvorova A, Watts JK. The chemical evolution of oligonucleotide therapies of clinical utility. Nat Biotechnol. 2017;35(3):238-248. doi: 10.1038/nbt.3765
- Egli M, Schlegel MK, Manoharan M. Acyclic (S)-glycol nucleic acid (S-GNA) modification of siRNAs improves the safety of RNAi therapeutics while maintaining potency. RNA. 2023;29(4):402-414. doi: 10.1261/rna.079526.122
- Puri S, Mazza M, Roy G, et al. Evolution of nanomedicine formulations for targeted delivery and controlled release. Adv Drug Deliv Rev. 2023;200:114962. doi: 10.1016/j.addr.2023.114962
- Penchovsky R, Georgieva AV, Dyakova V, Traykovska M, Pavlova N. Antisense and functional nucleic acids in rational drug development. Antibiotics (Basel). 2024;13(3):221. doi: 10.3390/antibiotics13030221
- Brown CR, Gupta S, Qin J, et al. Investigating the pharmacodynamic durability of GalNAc-siRNA conjugates. Nucleic Acids Res. 2020;48(21):11827-11844. doi: 10.1093/nar/gkaa670
- O’Brien J, Hayder H, Zayed Y, Peng C. Overview of MicroRNA biogenesis, mechanisms of actions, and circulation. Front Endocrinol (Lausanne). 2018;9:402. doi: 10.3389/fendo.2018.00402
- Yedavilli S, Singh AD, Singh D, Samal R. Nano-messengers of the heart: Promising theranostic candidates for cardiovascular maladies. Front Physiol. 2022;13:895322. doi: 10.3389/fphys.2022.895322
- Henning RJ. Cardiovascular exosomes and MicroRNAs in cardiovascular physiology and pathophysiology. J Cardiovasc Transl Res. 2021;14(2):195-212. doi: 10.1007/s12265-020-10040-5
- Li C, Ni YQ, Xu H, et al. Roles and mechanisms of exosomal non-coding RNAs in human health and diseases. Signal Transduct Target Ther. 2021;6(1):383. doi: 10.1038/s41392-021-00779-x
- Shu Z, Tan J, Miao Y, Zhang Q. The role of microvesicles containing microRNAs in vascular endothelial dysfunction. J Cell Mol Med. 2019;23(12):7933-7945. doi: 10.1111/jcmm.14716
- Zhang J, Li S, Li L, et al. Exosome and exosomal microRNA: Trafficking, sorting, and function. Genomics Proteomics Bioinformatics. 2015;13(1):17-24. doi: 10.1016/j.gpb.2015.02.001
- Assmann TS, Milagro FI, Martinez JA. Crosstalk between microRNAs, the putative target genes and the lncRNA network in metabolic diseases. Mol Med Rep. 2019;20(4):3543-3554. doi: 10.3892/mmr.2019.10595
- Bennett CF, Kordasiewicz HB, Cleveland DW. Antisense drugs make sense for neurological diseases. Annu Rev Pharmacol Toxicol. 2021;61:831-852. doi: 10.1146/annurev-pharmtox-010919-023738
- Garofalo M, Condorelli G, Croce CM. MicroRNAs in diseases and drug response. Curr Opin Pharmacol. 2008;8:661-667. doi: 10.1016/j.coph.2008.06.005
- Jae N, Dimmeler S. Noncoding RNAs in vascular diseases. Circ Res. 2020;126(9):1127-1145. doi: 10.1161/CIRCRESAHA.119.315938
- Chandan K, Gupta M, Sarwat M. Role of host and pathogen-derived MicroRNAs in immune regulation during infectious and inflammatory diseases. Front Immunol. 2019;10:3081. doi: 10.3389/fimmu.2019.03081
- Benavides-Aguilar JA, Torres-Copado A, Isidoro-Sanchez J, et al. The regulatory role of MicroRNAs in obesity and obesity-derived ailments. Genes (Basel). 2023;14(11):2070. doi: 10.3390/genes14112070
- Shah V, Shah J. Recent trends in targeting miRNAs for cancer therapy. J Pharm Pharmacol. 2020;72(12):1732-1749. doi: 10.1111/jphp.13351
- Kousar K, Ahmad T, Abduh MS, et al. miRNAs in regulation of tumor microenvironment, chemotherapy resistance, immunotherapy modulation and miRNA therapeutics in cancer. Int J Mol Sci. 2022;23(22):13822. doi: 10.3390/ijms232213822
- Veiga RN, Zambalde EP, Cox L, et al. Regulation of immune cells by microRNAs and microRNA-based cancer immunotherapy. Adv Exp Med Biol. 2022;1385:75-108. doi: 10.1007/978-3-031-08356-3_3
- Otmani K, Rouas R, Lewalle P. OncomiRs as noncoding RNAs having functions in cancer: Their role in immune suppression and clinical implications. Front Immunol. 2022;13:913951. doi: 10.3389/fimmu.2022.913951
- Momin MY, Gaddam RR, Kravitz M, Gupta A, Vikram A. The challenges and opportunities in the development of MicroRNA therapeutics: A multidisciplinary viewpoint. Cells. 2021;10(11):3097. doi: 10.3390/cells10113097
- Smith ES, Whitty E, Yoo B, Moore A, Sempere LF, Medarova Z. Clinical Applications of Short Non-Coding RNA-Based Therapies in the Era of Precision Medicine. Cancers (Basel). 2022;14(6):1588. doi: 10.3390/cancers14061588
- Bajan S, Hutvagner G. RNA-based therapeutics: From antisense oligonucleotides to miRNAs. Cells. 2020;9(1):137. doi: 10.3390/cells9010137
- Weidner J, Kolosionek E, Holmila R, et al. Gymnotic uptake of AntimiRs alter microRNA-34a levels in 2D and 3D epithelial cell culture. Mol Ther Nucleic Acids. 2023;33:898-907. doi: 10.1016/j.omtn.2023.08.014
- Bayraktar E, Bayraktar R, Oztatlici H, Lopez-Berestein G, Amero P, Rodriguez-Aguayo C. Targeting miRNAs and other non-coding RNAs as a therapeutic approach: An update. Noncoding RNA. 2023;9(2):27. doi: 10.3390/ncrna9020027
- Kilikevicius A, Meister G, Corey DR. Reexamining assumptions about miRNA-guided gene silencing. Nucleic Acids Res. 2022;50(2):617-634. doi: 10.1093/nar/gkab1256
- Roberts TC, Langer R, Wood MJA. Advances in oligonucleotide drug delivery. Nat Rev Drug Discov. 2020;19(10):673-694. doi: 10.1038/s41573-020-0075-7
- Kim T, Croce CM. MicroRNA: Trends in clinical trials of cancer diagnosis and therapy strategies. Exp Mol Med. 2023;55(7):1314-1321. doi: 10.1038/s12276-023-01050-9
- Traber GM, Yu AM. RNAi-based therapeutics and novel RNA bioengineering technologies. J Pharmacol Exp Ther. 2023;384(1):133-154. doi: 10.1124/jpet.122.001234
- Corydon IJ, Fabian-Jessing BK, Jakobsen TS, et al. 25 years of maturation: A systematic review of RNAi in the clinic. Mol Ther Nucleic Acids. 2023;33:469-482. doi: 10.1016/j.omtn.2023.07.018
- Baumann V, Winkler J. miRNA-based therapies: Strategies and delivery platforms for oligonucleotide and non-oligonucleotide agents. Future Med Chem. 2014;6(17):1967-1984. doi: 10.4155/fmc.14.116
- Zhang MM, Bahal R, Rasmussen TP, Manautou JE, Zhong XB. The growth of siRNA-based therapeutics: Updated clinical studies. Biochem Pharmacol. 2021;189:114432. doi: 10.1016/j.bcp.2021.114432
- Ambros V. The functions of animal microRNAs. Nature. 2004;431(7006):350-355. doi: 10.1038/nature02871
- Kariuki D, Asam K, Aouizerat BE, Lewis KA, Florez JC, Flowers E. Review of databases for experimentally validated human microRNA-mRNA interactions. Database (Oxford). 2023;2023:baad014. doi: 10.1093/database/baad014
- Alles J, Fehlmann T, Fischer U, et al. An estimate of the total number of true human miRNAs. Nucleic Acids Res. 2019;47(7):3353-3364. doi: 10.1093/nar/gkz097
- Hammond SM. An overview of microRNAs. Adv Drug Deliv Rev. 2015;87:3-14. doi: 10.1016/j.addr.2015.05.001
- Mohr AM, Mott JL. Overview of microRNA biology. Semin Liver Dis. 2015;35(1):3-11. doi: 10.1055/s-0034-1397344
- Hagedorn PH, Hansen BR, Koch T, Lindow M. Managing the sequence-specificity of antisense oligonucleotides in drug discovery. Nucleic Acids Res. 2017;45(5):2262-2282. doi: 10.1093/nar/gkx056
- Thibonnier M, Esau C. Metabolic benefits of Microrna-22 inhibition. Nucleic Acid Ther. 2020;30(2):104-116. doi: 10.1089/nat.2019.0820
- Thibonnier M, Esau C, Ghosh S, Wargent E, Stocker C. Metabolic and energetic benefits of microRNA-22 inhibition. BMJ Open Diabetes Res Care. 2020;8(1):e001478. doi: 10.1136/bmjdrc-2020-001478
- Keller A, Groger L, Tschernig T, et al. miRNATissueAtlas2: An update to the human miRNA tissue atlas. Nucleic Acids Res. 2022;50(D1):D211-D221. doi: 10.1093/nar/gkab808
- Thibonnier M, Ghosh S. Strategy for pre-clinical development of active targeting MicroRNA oligonucleotide therapeutics for unmet medical needs. Int J Mol Sci. 2023;24(8):7126. doi: 10.3390/ijms24087126
- Yang Z, Qin W, Huo J, Zhuo Q, Wang J, Wang L. MiR-22 modulates the expression of lipogenesis-related genes and promotes hepatic steatosis in vitro. FEBS Open Bio. 2021;11(1):322-332. doi: 10.1002/2211-5463.13026
- Kaur K, Vig S, Srivastava R, et al. Elevated hepatic miR- 22-3p expression impairs gluconeogenesis by silencing the wnt-responsive transcription factor Tcf7. Diabetes. 2015;64(11):3659-3669. doi: 10.2337/db14-1924
- Hu Y, Liu HX, Jena PK, Sheng L, Ali MR, Wan YY. miR-22 inhibition reduces hepatic steatosis via FGF21 and FGFR1 induction. JHEP Rep. 2020;2(2):100093. doi: 10.1016/j.jhepr.2020.100093
- Castano C, Novials A, Parrizas M. Exosomes from short-term high-fat or high-sucrose fed mice induce hepatic steatosis through different pathways. Cells. 2022;12(1):169. doi: 10.3390/cells12010169
- Panella R, Petri A, Desai BN, et al. MicroRNA-22 is a key regulator of lipid and metabolic homeostasis. Int J Mol Sci. 2023;24(16):12870. doi: 10.3390/ijms241612870
- Bennett CF, Baker BF, Pham N, Swayze E, Geary RS. Pharmacology of antisense drugs. Annu Rev Pharmacol Toxicol. 2017;57:81-105. doi: 10.1146/annurev-pharmtox-010716-104846
- Crooke ST, Baker BF, Crooke RM, Liang XH. Antisense technology: An overview and prospectus. Nat Rev Drug Discov. 2021;20:427-453. doi: 10.1038/s41573-021-00162-z
- Crooke ST, Liang XH, Baker BF, Crooke RM. Antisense technology: A review. J Biol Chem. 2021;296:100416. doi: 10.1016/j.jbc.2021.100416
- Goyenvalle A, Jimenez-Mallebrera C, van Roon W, et al. Considerations in the preclinical assessment of the safety of antisense oligonucleotides. Nucleic Acid Ther. 2023;33(1):1-16. doi: 10.1089/nat.2022.0061
- Nielsen PE. Sequence-selective DNA recognition by synthetic ligands. Bioconjug Chem. 1991;2(1):1-12. doi: 10.1021/bc00007a001
- Nielsen PE, Egholm M, Berg RH, Buchardt O. Sequence-selective recognition of DNA by strand displacement with a thymine-substituted polyamide. Science. 1991;254(5037):1497-1500. doi: 10.1126/science.1962210
- Pradeep SP, Malik S, Slack FJ, Bahal R. Unlocking the potential of chemically modified peptide nucleic acids for RNA-based therapeutics. RNA. 2023;29(4):434-445. doi: 10.1261/rna.079498.122
- Suparpprom C, Vilaivan T. Perspectives on conformationally constrained peptide nucleic acid (PNA): Insights into the structural design, properties and applications. RSC Chem Biol. 2022;3(6):648-697. doi: 10.1039/d2cb00017b
- Dragulescu-Andrasi A, Rapireddy S, Frezza BM, Gayathri C, Gil RR, Ly DH. A simple gamma-backbone modification preorganizes peptide nucleic acid into a helical structure. J Am Chem Soc. 2006;128(31):10258-10267. doi: 10.1021/ja0625576
- Brazil R. Peptide nucleic acids promise new therapeutics and gene editing tools. ACS Cent Sci. 2023;9(1):3-6. doi: 10.1021/acscentsci.3c00016
- Popella L, Jung J, Do PT, Hayward RJ, Barquist L, Vogel J. Comprehensive analysis of PNA-based antisense antibiotics targeting various essential genes in uropathogenic Escherichia coli. Nucleic Acids Res. 2022;50(11):6435-6452. doi: 10.1093/nar/gkac362
- Jing Z, Qi R, Thibonnier M, Ren P. Molecular dynamics study of the hybridization between RNA and modified oligonucleotides. J Chem Theory Comput. 2019;15(11):6422-6432. doi: 10.1021/acs.jctc.9b00519
- Malik S, Lim J, Slack FJ, Braddock DT, Bahal R. Next generation miRNA inhibition using short anti-seed PNAs encapsulated in PLGA nanoparticles. J Control Release. 2020;327:406-419. doi: 10.1016/j.jconrel.2020.08.026
- Cheng CJ, Bahal R, Babar IA, et al. MicroRNA silencing for cancer therapy targeted to the tumour microenvironment. Nature. 2015;518(7537):107-110. doi: 10.1038/nature13905
- Dhuri K, Gaddam RR, Vikram A, Slack FJ, Bahal R. Therapeutic potential of chemically modified, synthetic, triplex peptide nucleic acid-based oncomir inhibitors for cancer therapy. Cancer Res. 2021;81(22):5613-5624. doi: 10.1158/0008-5472.CAN-21-0736
- Fabani MM, Gait MJ. miR-122 targeting with LNA/2’- O-methyl oligonucleotide mixmers, peptide nucleic acids (PNA), and PNA-peptide conjugates. RNA. 2008;14(2):336-346. doi: 10.1261/rna.844108
- Torres AG, Threlfall RN, Gait MJ. Potent and sustained cellular inhibition of miR-122 by lysine-derivatized peptide nucleic acids (PNA) and phosphorothioate locked nucleic acid (LNA)/2’-O-methyl (OMe) mixmer anti-miRs in the absence of transfection agents. Artif DNA PNA XNA. 2011;2(3):71-78. doi: 10.4161/adna.17731
- Gaddam RR, Dhuri K, Kim YR, et al. γ peptide nucleic acid-based miR-122 inhibition rescues vascular endothelial dysfunction in mice fed a high-fat diet. J Med Chem. 2022;65(4):3332-3342. doi: 10.1021/acs.jmedchem.1c01831
- Kim K, Kim HH, Lee CH, et al. Therapeutic efficacy of modified anti-miR21 in metastatic prostate cancer. Biochem Biophys Res Commun. 2020;529(3):707-713. doi: 10.1016/j.bbrc.2020.05.215
- Dhuri K, Pradeep SP, Shi J, et al. Simultaneous Targeting of multiple oncomiRs with phosphorothioate or PNA-based Anti-miRs in Lymphoma cell lines. Pharm Res. 2022;39(11):2709-2720. doi: 10.1007/s11095-022-03383-y
- Papi C, Gasparello J, Zurlo M, et al. Combined treatment of bronchial epithelial Calu-3 cells with peptide nucleic acids targeting miR-145-5p and miR-101-3p: Synergistic enhancement of the expression of the cystic fibrosis transmembrane conductance regulator (CFTR) gene. Int J Mol Sci. 2022;23(16):9348. doi: 10.3390/ijms23169348
- Fabbri E, Manicardi A, Tedeschi T, et al. Modulation of the biological activity of microRNA-210 with peptide nucleic acids (PNAs). ChemMedChem. 2011;6(12):2192-2202. doi: 10.1002/cmdc.201100270
- Gasparello J, Papi C, Zurlo M, et al. MicroRNAs miR-584-5p and miR-425-3p Are Up-regulated in plasma of colorectal cancer (CRC) patients: Targeting with inhibitor peptide nucleic acids is associated with induction of apoptosis in colon cancer cell lines. Cancers (Basel). 2022;15(1):128. doi: 10.3390/cancers15010128
- Dhuri K, Vyas RN, Blumenfeld L, Verma R, Bahal R. Nanoparticle delivered Anti-miR-141-3p for stroke therapy. Cells. 2021;10(5):1011. doi: 10.3390/cells10051011
- Milani R, Brognara E, Fabbri E, et al. Targeting miR-155-5p and miR-221-3p by peptide nucleic acids induces caspase-3 activation and apoptosis in temozolomide-resistant T98G glioma cells. Int J Oncol. 2019;55(1):59-68. doi: 10.3892/ijo.2019.4810
- Wang Y, Malik S, Suh HW, et al. Anti-seed PNAs targeting multiple oncomiRs for brain tumor therapy. Sci Adv. 2023;9(6):eabq7459. doi: 10.1126/sciadv.abq7459
- Price NL, Miguel V, Ding W, et al. Genetic deficiency or pharmacological inhibition of miR-33 protects from kidney fibrosis. JCI Insight. 2019;4(22):e131102. doi: 10.1172/jci.insight.131102
- Zhang X, Rotllan N, Canfran-Duque A, et al. Targeted suppression of miRNA-33 using pHLIP improves atherosclerosis regression. Circ Res. 2022;131(1):77-90. doi: 10.1161/CIRCRESAHA.121.320296
- Malik S, Kumar V, Liu CH, et al. Head on comparison of Self- and Nano-assemblies of Gamma peptide nucleic acid amphiphiles. Adv Funct Mater. 2022;32(7):2109552. doi: 10.1002/adfm.202109552
- Geary RS, Norris D, Yu R, Bennett CF. Pharmacokinetics, biodistribution and cell uptake of antisense oligonucleotides. Adv Drug Deliv Rev. 2015;87:46-51. doi: 10.1016/j.addr.2015.01.008
- Andersson S, Antonsson M, Elebring M, Jansson-Lofmark R, Weidolf L. Drug metabolism and pharmacokinetic strategies for oligonucleotide- and mRNA-based drug development. Drug Discov Today. 2018;23(10):1733-1745. doi: 10.1016/j.drudis.2018.05.030
- Vervaeke P, Borgos SE, Sanders NN, Combes F. Regulatory guidelines and preclinical tools to study the biodistribution of RNA therapeutics. Adv Drug Deliv Rev. 2022;184:114236. doi: 10.1016/j.addr.2022.114236
- McDougall R, Ramsden D, Agarwal S, et al. The nonclinical disposition and pharmacokinetic/pharmacodynamic properties of N-Acetylgalactosamine-conjugated small interfering RNA are highly predictable and build confidence in translation to human. Drug Metab Dispos. 2022;50(6):781-797. doi: 10.1124/dmd.121.000428
- Fairweather S, Rogers M, Stoulig P, et al. Nuclease resistance and protein recognition properties of DNA and hybrid PNA-DNA four-way junctions. Biophys Chem. 2022;289:106863. doi: 10.1016/j.bpc.2022.106863
- Demidov VV, Potaman VN, Frank-Kamenetskii MD, et al. Stability of peptide nucleic acids in human serum and cellular extracts. Biochem Pharmacol. 1994;48(6):1310-1313. doi: 10.1016/0006-2952(94)90171-6
- Østergaard ME, De Hoyos CL, Wan WB, et al. Understanding the effect of controlling phosphorothioate chirality in the DNA gap on the potency and safety of gapmer antisense oligonucleotides. Nucleic Acids Res. 2020;48(4):1691-1700. doi: 10.1093/nar/gkaa031
- Hall J. Future directions for medicinal chemistry in the field of oligonucleotide therapeutics. RNA. 2023;29(4):423-433. doi: 10.1261/rna.079511.122
- Funder ED, Albaek N, Moisan A, Sewing S, Koch T. Refining LNA safety profile by controlling phosphorothioate stereochemistry. PLoS One. 2020;15(6):e0232603. doi: 10.1371/journal.pone.0232603
- Brooks WH, Guida WC, Daniel KG. The significance of chirality in drug design and development. Curr Top Med Chem. 2011;11(7):760-770. doi: 10.2174/156802611795165098
- McVicker RU, O’Boyle NM. Chirality of new drug approvals (2013-2022): Trends and perspectives. J Med Chem. 2024;67(4):2305-2320. doi: 10.1021/acs.jmedchem.3c02239
- Iwamoto N, Butler DCD, Svrzikapa N, et al. Control of phosphorothioate stereochemistry substantially increases the efficacy of antisense oligonucleotides. Nat Biotechnol. 2017;35(9):845-851. doi: 10.1038/nbt.3948
- Jahns H, Taneja N, Willoughby JLS, et al. Chirality matters: Stereo-defined phosphorothioate linkages at the termini of small interfering RNAs improve pharmacology in vivo. Nucleic Acids Res. 2022;50(3):1221-1240. doi: 10.1093/nar/gkab544
- Liu W, Iwamoto N, Marappan S, et al. Impact of stereopure chimeric backbone chemistries on the potency and durability of gene silencing by RNA interference. Nucleic Acids Res. 2023;51(9):4126-4147. doi: 10.1093/nar/gkad268
- Ryan CA, Rozners E. Impact of chirality and position of lysine conjugation in triplex-forming peptide nucleic acids. ACS Omega. 2020;5(44):28722-28729. doi: 10.1021/acsomega.0c04021
- Del Bene A, D’Aniello A, Tomassi S, et al. Ultrasound-assisted Peptide Nucleic Acids Synthesis (US-PNAS). Ultrason Sonochem. 2023;95:106360. doi: 10.1016/j.ultsonch.2023.106360
- Shen W, De Hoyos CL, Sun H, Vickers TA, Liang XH, Crooke ST. Acute hepatotoxicity of 2’ fluoro-modified 5-10-5 gapmer phosphorothioate oligonucleotides in mice correlates with intracellular protein binding and the loss of DBHS proteins. Nucleic Acids Res. 2018;46(5):2204-2217. doi: 10.1093/nar/gky060
- Shen W, De Hoyos CL, Migawa MT, et al. Chemical modification of PS-ASO therapeutics reduces cellular protein-binding and improves the therapeutic index. Nat Biotechnol. 2019;37(6):640-650. doi: 10.1038/s41587-019-0106-2
- Corey DR, Damha MJ, Manoharan M. Challenges and opportunities for nucleic acid therapeutics. Nucleic Acid Ther. 2022;32(1):8-13. doi: 10.1089/nat.2021.0085
- Mangla P, Vicentini Q, Biscans A. therapeutic oligonucleotides: An outlook on chemical strategies to improve endosomal trafficking. Cells. 2023;12(18):2253. doi: 10.3390/cells12182253
- Wan WB, Seth PP. The medicinal chemistry of therapeutic oligonucleotides. J Med Chem. 2016;59(21):9645-9667. doi: 10.1021/acs.jmedchem.6b00551
- Yavin E. Peptide nucleic acids: Applications in biomedical sciences. Molecules. 2020;25(15):3317. doi: 10.3390/molecules25153317
- Hansen AM, Bonke G, Hogendorf WFJ, et al. Microwave-assisted solid-phase synthesis of antisense acpP peptide nucleic acid-peptide conjugates active against colistin- and tigecycline-resistant E. coli and K. pneumoniae. Eur J Med Chem. 2019;168:134-145. doi: 10.1016/j.ejmech.2019.02.024
- Klabenkova K, Fokina A, Stetsenko D. Chemistry of peptide-Oligonucleotide conjugates: A review. Molecules. 2021;26(17):5420. doi: 10.3390/molecules26175420
- Li C, Zhang G, Mohapatra S, et al. Machine learning guides peptide nucleic acid flow synthesis and sequence design. Adv Sci (Weinh). 2022;9(34):e2201988. doi: 10.1002/advs.202201988
- Li C, Callahan AJ, Phadke KS, et al. Automated flow synthesis of peptide-PNA conjugates. ACS Cent Sci. 2022;8(2):205-213. doi: 10.1021/acscentsci.1c01019
- Hammond SM, Aartsma-Rus A, Alves S, et al. Delivery of oligonucleotide-based therapeutics: Challenges and opportunities. EMBO Mol Med. 2021;13(4):e13243. doi: 10.15252/emmm.202013243
- McCartan R, Khorkova O, Volmar CH, Wahlestedt C. Nucleic acid-based therapeutics for the treatment of central nervous system disorders. Front Genet. 2023;14:1250276. doi: 10.3389/fgene.2023.1250276
- Baker BF, Xia S, Partridge W, et al. Safety and tolerability of GalNAc3-conjugated antisense drugs compared to the same-sequence 2’-O-Methoxyethyl-modified antisense drugs: Results from an integrated assessment of phase 1 clinical trial data. Nucleic Acid Ther. 2024;34:18-25. doi: 10.1089/nat.2023.0026
- Sarkar S, Colon-Roura G, Pearse A, Armitage BA. Targeting a KRAS i-motif forming sequence by unmodified and gamma-modified peptide nucleic acid oligomers. Biopolymers. 2023;114(1):e23529. doi: 10.1002/bip.23529
- Wickline SA, Hou KK, Pan H. Peptide-based nanoparticles for systemic extrahepatic delivery of therapeutic nucleotides. Int J Mol Sci. 2023;24(11):9455. doi: 10.3390/ijms24119455
- Tsylents U, Siekierska I, Trylska J. Peptide nucleic acid conjugates and their antimicrobial applications-a mini-review. Eur Biophys J. 2023;52:533-544. doi: 10.1007/s00249-023-01673-w
- Available from: https://www.olipass.com/front/eng/ competitive/pipeline.do [Last accessed on 2024 July 05].
- Glatz JFC, Luiken J. Dynamic role of the transmembrane glycoprotein CD36 (SR-B2) in cellular fatty acid uptake and utilization. J Lipid Res. 2018;59(7):1084-1093. doi: 10.1194/jlr.R082933
- Glatz JF, Luiken JJ. From fat to FAT (CD36/SR-B2): Understanding the regulation of cellular fatty acid uptake. Biochimie. 2017;136:21-26. doi: 10.1016/j.biochi.2016.12.007
- Wang L, Wang YS, Mugiyanto E, Chang WC, Yvonne Wan YJ. MiR-22 as a metabolic silencer and liver tumor suppressor. Liver Res. 2020;4(2):74-80. doi: 10.1016/j.livres.2020.06.001
- Paul D, Miller MH, Born J, et al. The promising therapeutic potential of oligonucleotides for pulmonary fibrotic diseases. Expert Opin Drug Discov. 2023;18(2):193-206. doi: 10.1080/17460441.2023.2160439
- Bao X, Liang Y, Chang H, et al. Targeting proprotein convertase subtilisin/kexin type 9 (PCSK9): From bench to bedside. Signal Transduct Target Ther. 2024;9(1):13. doi: 10.1038/s41392-023-01690-3
- Katsiki N, Vrablik M, Banach M, Gouni-Berthold I. Inclisiran, Low-density lipoprotein cholesterol and lipoprotein (a). Pharmaceuticals (Basel). 2023;16(4):577. doi: 10.3390/ph16040577
- Huang HYR, Badar S, Said M, et al. The advent of RNA-based therapeutics for metabolic syndrome and associated conditions: A comprehensive review of the literature. Mol Biol Rep. 2024;51(1):493. doi: 10.1007/s11033-024-09457-x
- Nappi F, Avtaar Singh SS, Jitendra V, Alzamil A, Schoell T. The Roles of microRNAs in the Cardiovascular System. Int J Mol Sci. 2023;24(18):14277. doi: 10.3390/ijms241814277
- Lobstein T. World Obesity Atlas 2024; 2024. Available from: https://data.worldobesity.org/publications/?cat=22 [Last accessed 2024 July 05].
- Yang Z, Wang L. Current, emerging, and potential therapies for non-alcoholic steatohepatitis. Front Pharmacol. 2023;14:1152042. doi: 10.3389/fphar.2023.1152042
- Knerr PJ, Mowery SA, Douros JD, et al. Next generation GLP-1/GIP/glucagon triple agonists normalize body weight in obese mice. Mol Metab. 2022;63:101533. doi: 10.1016/j.molmet.2022.101533
- Newsome PN, Ambery P. Incretins (GLP-1 receptor agonists and dual/triple agonists) and the liver. J Hepatol. 2023;79(6):1557-1565. doi: 10.1016/j.jhep.2023.07.033
- Jastreboff AM, Kaplan LM, Frias JP, et al. Triple-hormone-receptor agonist retatrutide for obesity - A phase 2 trial. N Engl J Med. 2023;389:514-526. doi: 10.1056/NEJMoa2301972
- Harrison SA, Taub R, Neff GW, et al. Resmetirom for nonalcoholic fatty liver disease: A randomized, double-blind, placebo-controlled phase 3 trial. Nat Med. 2023;29(11):2919-2928. doi: 10.1038/s41591-023-02603-1
- Karim G, Bansal MB. Resmetirom: An orally administered, smallmolecule, liver-directed, β-selective THR agonist for the treatment of non-alcoholic fatty liver disease and non-alcoholic Steatohepatitis. touchREV Endocrinol. 2023;19(1):60-70. doi: 10.17925/EE.2023.19.1.60
- Harrison SA, Bedossa P, Guy CD, et al. A phase 3, randomized, controlled trial of resmetirom in NASH with liver fibrosis. N Engl J Med. 2024;390(6):497-509. doi: 10.1056/NEJMoa2309000
- Telci Caklili O, Cesur M, Mikhailidis DP, Rizzo M. Novel anti-obesity therapies and their different effects and safety profiles: A critical overview. Diabetes Metab Syndr Obes. 2023;16:1767-1774. doi: 10.2147/DMSO.S392684
- Rakocevic J, Dobric M, Vucic R, et al. Small interfering ribonucleic acid as lipid-lowering therapy: Inclisiran in focus. Int J Mol Sci. 2023;24(6):6012. doi: 10.3390/ijms24066012
- Pirillo A, Catapano AL. Inclisiran: How Widely and When Should We Use It? Curr Atheroscler Rep. 2022;24(10):803-811. doi: 10.1007/s11883-022-01056-0
- Zhang Y, Chen H, Hong L, et al. Inclisiran: A new generation of lipid-lowering siRNA therapeutic. Front Pharmacol. 2023;14:1260921. doi: 10.3389/fphar.2023.1260921
- Desai NR, Campbell C, Electricwala B, et al. Cost effectiveness of inclisiran in atherosclerotic cardiovascular patients with elevated low-density lipoprotein cholesterol despite statin use: A threshold analysis. Am J Cardiovasc Drugs. 2022;22(5):545-556. doi: 10.1007/s40256-022-00534-9
- Mody R, Valentine WJ, Hoog M, Sharland H, Belger M. Tirzepatide 10 and 15 mg vs semaglutide 2.0 mg: A long-term cost-effectiveness analysis in patients with type 2 diabetes in the United States. J Manag Care Spec Pharm. 2024;30(2):153-162. doi: 10.18553/jmcp.2024.30.2.153
- Kim N, Wang J, Burudpakdee C, et al. Cost-effectiveness analysis of semaglutide 2.4 mg for the treatment of adult patients with overweight and obesity in the United States. J Manag Care Spec Pharm. 2022;28(7):740-752. doi: 10.18553/jmcp.2022.28.7.740
- Damase TR, Sukhovershin R, Boada C, Taraballi F, Pettigrew RI, Cooke JP. The limitless future of RNA therapeutics. Front Bioeng Biotechnol. 2021;9:628137. doi: 10.3389/fbioe.2021.628137
- Zhang C, Zhang B. RNA therapeutics: Updates and future potential. Sci China Life Sci. 2023;66(1):12-30. doi: 10.1007/s11427-022-2171-2