Navigating the complex landscape of cardiac metabolism in health and disease states
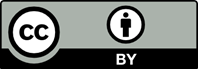
The intricate interplay between cardiovascular health and metabolic regulation forms a critical junction in understanding the complexities of heart-related conditions. Cardiometabolic regulation orchestrates a sophisticated network of factors governing energy utilization, substrate metabolism, and cellular processes within the cardiovascular system. Balancing these mechanisms is pivotal for optimal heart function, considering the substantial energy demands for both contractile and non-contractile activities. In a healthy heart, fatty acids (FAs) derived from FA β-oxidation contribute to approximately 70% of total energy production. However, emerging evidence sheds light on pathological changes in the heart that lead to profound metabolic alterations. These alterations involve a shift from predominant FA utilization to alternative substrates such as glucose and ketone bodies, accompanied by an increased reliance on FAs. This metabolic remodeling extends beyond substrate metabolism, encompassing changes in transporter expression, the activity of metabolic-related proteins, hormonal functions, and cardiac mitochondrial energetics. This comprehensive review article delves into the intricate web of cardiometabolic regulation, elucidating the multifaceted factors influencing cardiac metabolism across diverse states encompassing health, metabolic disorders, and heart diseases. Unraveling the molecular intricacies and interconnected pathways shaping cardiac metabolism in various physiological and pathological conditions provides critical insights into the adaptive mechanisms and dysregulations associated with heart-related conditions. Furthermore, the exploration of these regulatory mechanisms offers promising avenues for targeted therapeutic interventions and diagnostic strategies in cardiovascular medicine. Integrating multidisciplinary approaches and leveraging advanced technologies will facilitate a deeper understanding of cardiac metabolism, paving the way for innovative interventions to mitigate metabolic dysregulation and optimize cardiac health.
1. Stanley WC, Chandler MP. Energy metabolism in the normal and failing heart: Potential for therapeutic interventions. Heart Fail Rev. 2002;7(2):115-130. doi: 10.1023/a:1015320423577
2. Taegtmeyer H. Energy metabolism of the heart: From basic concepts to clinical applications. Curr Probl Cardiol. 1994;19(2):59-113. doi: 10.1016/0146-2806(94)90008-6
3. Bing RJ, Siegel A, Ungar I, Gilbert M. Metabolism of the human heart. II. Studies on fat, ketone and amino acid metabolism. Am J Med. 1954;16(4):504-515. doi: 10.1016/0002-9343(54)90365-4
4. Wisneski JA, Gertz EW, Neese RA, Mayr M. Myocardial metabolism of free fatty acids. Studies with 14C-labeled substrates in humans. J Clin Invest. 1987;79(2):359-366. doi: 10.1172/JCI112820
5. Lehman JJ, Kelly DP. Transcriptional activation of energy metabolic switches in the developing and hypertrophied heart. Clin Exp Pharmacol Physiol. 2002;29(4):339-345. doi: 10.1046/j.1440-1681.2002.03655.x
6. Sack MN, Disch DL, Rockman HA, Kelly DP. A role for Sp and nuclear receptor transcription factors in a cardiac hypertrophic growth program. Proc Natl Acad Sci U S A. 1997;94(12):6438-6443. doi: 10.1073/pnas.94.12.6438
7. Stanley WC, Recchia FA, Lopaschuk GD. Myocardial substrate metabolism in the normal and failing heart. Physiol Rev. 2005;85(3):1093-1129. doi: 10.1152/physrev.00006.2004
8. Fillmore N, Mori J, Lopaschuk GD. Mitochondrial fatty acid oxidation alterations in heart failure, ischaemic heart disease and diabetic cardiomyopathy. Br J Pharmacol. 2014;171(8):2080-2090. doi: 10.1111/bph.12475
9. Liu Q, Docherty JC, Rendell JC, Clanachan AS, Lopaschuk GD. High levels of fatty acids delay the recovery of intracellular pH and cardiac efficiency in post-ischemic hearts by inhibiting glucose oxidation. J Am Coll Cardiol. 2002;39(4):718-725. doi: 10.1016/s0735-1097(01)01803-4
10. Randle PJ, England PJ, Denton RM. Control of the tricarboxylate cycle and its interactions with glycolysis during acetate utilization in rat heart. Biochem J. 1970;117(4):677-695. doi: 10.1042/bj1170677
11. Randle PJ, Garland PB, Hales CN, Newsholme EA. The glucose fatty-acid cycle. Its role in insulin sensitivity and the metabolic disturbances of diabetes mellitus. Lancet. 1963;1(7285):785-789. doi: 10.1016/s0140-6736(63)91500-9
12. Tanajak P, Sa-Nguanmoo P, Wang X, et al. Fibroblast growth factor 21 (FGF21) therapy attenuates left ventricular dysfunction and metabolic disturbance by improving FGF21 sensitivity, cardiac mitochondrial redox homoeostasis and structural changes in pre-diabetic rats. Acta Physiol (Oxf). 2016;217(4):287-299. doi: 10.1111/apha.12698
13. Chen WJ, Diamant M, de Boer K, et al. Effects of exenatide on cardiac function, perfusion, and energetics in type 2 diabetic patients with cardiomyopathy: A randomized controlled trial against insulin glargine. Cardiovasc Diabetol. 2017;16(1):67. doi: 10.1186/s12933-017-0549-z
14. Lincoff AM, Bhasin S, Flevaris P, et al. Cardiovascular safety of testosterone-replacement therapy. N Engl J Med. 2023;389(2):107-117. doi: 10.1056/NEJMoa2215025
15. Christakis GT, Weisel RD, Mickle DA, et al. Right ventricular function and metabolism. Circulation. 1990;82(5 Suppl):IV332-IV340.
16. Sato K, Kashiwaya Y, Keon CA, et al. Insulin, ketone bodies, and mitochondrial energy transduction. FASEB J. 1995;9(8):651-658. doi: 10.1096/fasebj.9.8.7768357
17. Mudaliar S, Alloju S, Henry RR. Can a shift in fuel energetics explain the beneficial cardiorenal outcomes in the EMPA-REG OUTCOME study? A unifying hypothesis. Diabetes Care. 2016;39(7):1115-1122. doi: 10.2337/dc16-0542
18. Ferrannini E, Mark M, Mayoux E. CV protection in the EMPA-REG OUTCOME Trial: A “thrifty substrate” hypothesis. Diabetes Care. 2016;39(7):1108-1114. doi: 10.2337/dc16-0330
19. Shao D, Tian R. Glucose transporters in cardiac metabolism and hypertrophy. Compr Physiol. 2015;6(1):331-351. doi: 10.1002/cphy.c150016
20. Lopaschuk GD, Ussher JR, Folmes CD, Jaswal JS, Stanley WC. Myocardial fatty acid metabolism in health and disease. Physiol Rev. 2010;90(1):207-258. doi: 10.1152/physrev.00015.2009
21. Szablewski L. Glucose transporters in healthy heart and in cardiac disease. Int J Cardiol. 2017;230:70-75. doi: 10.1016/j.ijcard.2016.12.083
22. Schwenk RW, Luiken JJ, Bonen A, Glatz JF. Regulation of sarcolemmal glucose and fatty acid transporters in cardiac disease. Cardiovasc Res. 2008;79(2):249-258. doi: 10.1093/cvr/cvn116
23. Ussher JR, Folmes CD, Keung W, et al. Inhibition of serine palmitoyl transferase I reduces cardiac ceramide levels and increases glycolysis rates following diet-induced insulin resistance. PLoS One. 2012;7(5):e37703. doi: 10.1371/journal.pone.0037703
24. Dyck JR, Cheng JF, Stanley WC, et al. Malonyl coenzyme a decarboxylase inhibition protects the ischemic heart by inhibiting fatty acid oxidation and stimulating glucose oxidation. Circ Res. 2004;94(9):e78-e84. doi: 10.1161/01.RES.0000129255.19569.8f
25. Kolwicz SC Jr., Olson DP, Marney LC, Garcia-Menendez L, Synovec RE, Tian R. Cardiac-specific deletion of acetyl CoA carboxylase 2 prevents metabolic remodeling during pressure-overload hypertrophy. Circ Res. 2012;111(6):728-738. doi: 10.1161/CIRCRESAHA.112.268128
26. Gosteli-Peter MA, Schmid C, Zapf J. Triiodothyronine increases glucose transporter isotype 4 mRNA expression, glucose transport, and glycogen synthesis in adult rat cardiomyocytes in long-term culture. Biochem Biophys Res Commun. 1996;221(3):521-524. doi: 10.1006/bbrc.1996.0629
27. Becker C, Sevilla L, Tomas E, Palacin M, Zorzano A, Fischer Y. The endosomal compartment is an insulin-sensitive recruitment site for GLUT4 and GLUT1 glucose transporters in cardiac myocytes. Endocrinology. 2001;142(12):5267-5276. doi: 10.1210/endo.142.12.8555
28. Fischer Y, Thomas J, Sevilla L, et al. Insulin-induced recruitment of glucose transporter 4 (GLUT4) and GLUT1 in isolated rat cardiac myocytes. Evidence of the existence of different intracellular GLUT4 vesicle populations. J Biol Chem. 1997;272(11):7085-7092. doi: 10.1074/jbc.272.11.7085
29. Mueckler M, Thorens B. The SLC2 (GLUT) family of membrane transporters. Mol Aspects Med. 2013;34(2-3):121-138. doi: 10.1016/j.mam.2012.07.001
30. Zhou L, Cryan EV, D’Andrea MR, Belkowski S, Conway BR, Demarest S. Human cardiomyocytes express high level of Na+/glucose cotransporter 1 (SGLT1). J Cell Biochem. 2003;90(2):339-346. doi: 10.1002/jcb.10631
31. Banerjee SK, McGaffin KR, Pastor-Soler NM, Ahmad F. SGLT1 is a novel cardiac glucose transporter that is perturbed in disease states. Cardiovasc Res. 2009;84(1):111-118. doi: 10.1093/cvr/cvp190.
32. Laffel L. Ketone bodies: A review of physiology, pathophysiology and application of monitoring to diabetes. Diabetes Metab Res Rev. 1999;15(6):412-426. doi: 10.1002/(sici)1520-7560(199911/12)15:6<412::aid-dmrr72>3.0.co;2-8
33. Akram M. A focused review of the role of ketone bodies in health and disease. J Med Food. 2013;16(11):965-967. doi: 10.1089/jmf.2012.2592
34. Cahill GF Jr. Fuel metabolism in starvation. Annu Rev Nutr. 2006;26:1-22. doi: 10.1146/annurev.nutr.26.061505.111258
35. Veech RL. The therapeutic implications of ketone bodies: the effects of ketone bodies in pathological conditions: Ketosis, ketogenic diet, redox states, insulin resistance, and mitochondrial metabolism. Prostaglandins Leukot Essent Fatty Acids. 2004;70(3):309-319. doi: 10.1016/j.plefa.2003.09.007
36. Aubert G, Martin OJ, Horton JL, et al. The failing heart relies on ketone bodies as a fuel. Circulation. 2016;133(8):698-705. doi: 10.1161/CIRCULATIONAHA.115.017355
37. Kanikarla-Marie P, Jain SK. Hyperketonemia and ketosis increase the risk of complications in type 1 diabetes. Free Radic Biol Med. 2016;95:268-277. doi: 10.1016/j.freeradbiomed.2016.03.020
38. Sugden MC, Holness MJ. Recent advances in mechanisms regulating glucose oxidation at the level of the pyruvate dehydrogenase complex by PDKs. Am J Physiol Endocrinol Metab. 2003;284(5):E855-E862. doi: 10.1152/ajpendo.00526.2002
39. Jaswal JS, Keung W, Wang W, Ussher JR, Lopaschuk GD. Targeting fatty acid and carbohydrate oxidation--a novel therapeutic intervention in the ischemic and failing heart. Biochim Biophys Acta. 2011;1813(7):1333-1350. doi: 10.1016/j.bbamcr.2011.01.015
40. Fisher FM, Chui PC, Antonellis PJ, et al. Obesity is a fibroblast growth factor 21 (FGF21)-resistant state. Diabetes. 2010;59(11):2781-2789. doi: 10.2337/db10-0193
41. Atkinson LL, Kozak R, Kelly SE, Besikci AO, Russell JC, Lopaschuk GD. Potential mechanisms and consequences of cardiac triacylglycerol accumulation in insulin-resistant rats. Am J Physiol Endocrinol Metab. 2003;284(5):E923-E930. doi: 10.1152/ajpendo.00360.2002
42. Zeng K, Tian L, Patel R, et al. Diet polyphenol curcumin stimulates hepatic Fgf21 production and restores its sensitivity in high-fat-diet-fed male mice. Endocrinology. 2017;158(2):277-292. doi: 10.1210/en.2016-1596
43. Supakul L, Pintana H, Apaijai N, Chattipakorn S, Shinlapawittayatorn K, Chattipakorn N. Protective effects of garlic extract on cardiac function, heart rate variability, and cardiac mitochondria in obese insulin-resistant rats. Eur J Nutr. 2014;53(3):919-928. doi: 10.1007/s00394-013-0595-6
44. Scarpace PJ, Zhang Y. Leptin resistance: A prediposing factor for diet-induced obesity. Am J Physiol Regul Integr Comp Physiol. 2009;296(3):R493-R500. doi: 10.1152/ajpregu.90669.2008
45. Vasselli JR, Scarpace PJ, Harris RB, Banks WA. Dietary components in the development of leptin resistance. Adv Nutr. 2013;4(2):164-175. doi: 10.3945/an.112.003152
46. Sainz N, Barrenetxe J, Moreno-Aliaga MJ, Martinez JA. Leptin resistance and diet-induced obesity: Central and peripheral actions of leptin. Metabolism. 2015;64(1):35-46. doi: 10.1016/j.metabol.2014.10.015
47. Pratchayasakul W, Chattipakorn N, Chattipakorn SC. Effects of estrogen in preventing neuronal insulin resistance in hippocampus of obese rats are different between genders. Life Sci. 2011;89(19-20):702-707. doi: 10.1016/j.lfs.2011.08.011
48. Montessuit, C, Lerch R. Regulation and dysregulation of glucose transport in cardiomyocytes. Biochim Biophys Acta. 2013;1833(4):848-856. doi: 10.1016/j.bbamcr.2012.08.009
49. Stratmann B, Tschoepe D. The diabetic heart: Sweet, fatty and stressed. Expert Rev Cardiovasc Ther. 2011;9(9):1093-1096. doi: 10.1586/erc.11.109
50. Carley AN, Atkinson LL, Bonen A, et al. Mechanisms responsible for enhanced fatty acid utilization by perfused hearts from type 2 diabetic db/db mice. Arch Physiol Biochem. 2007;113(2):65-75. doi: 10.1080/13813450701422617
51. Coort SL, Hasselbaink DM, Koonen DP, et al. Enhanced sarcolemmal FAT/CD36 content and triacylglycerol storage in cardiac myocytes from obese zucker rats. Diabetes. 2004;53(7):1655-1663. doi: 10.2337/diabetes.53.7.1655
52. Chabowski A, Coort SL, Calles-Escandon J, et al. The subcellular compartmentation of fatty acid transporters is regulated differently by insulin and by AICAR. FEBS Lett. 2005;579(11):2428-2432. doi: 10.1016/j.febslet.2004.11.118
53. Planavila A, Redondo I, Hondares E, et al. Fibroblast growth factor 21 protects against cardiac hypertrophy in mice. Nat Commun. 2013;4:2019. doi: 10.1038/ncomms3019
54. Buchanan J, Mazumder PK, Hu P, et al. Reduced cardiac efficiency and altered substrate metabolism precedes the onset of hyperglycemia and contractile dysfunction in two mouse models of insulin resistance and obesity. Endocrinology. 2005;146(12):5341-5349. doi: 10.1210/en.2005-0938
55. Westermeier F, Navarro-Marquez M, Lopez-Crisosto C, et al. Defective insulin signaling and mitochondrial dynamics in diabetic cardiomyopathy. Biochim Biophys Acta. 2015;1853(5):1113-1118. doi: 10.1016/j.bbamcr.2015.02.005
56. Schrauwen-Hinderling VB, Kooi ME, Schrauwen P. Mitochondrial function and diabetes: Consequences for skeletal and cardiac muscle metabolism. Antioxid Redox Signal. 2016;24(1):39-51. doi: 10.1089/ars.2015.6291
57. Young LH, Coven DL, Russell RR 3rd. Cellular and molecular regulation of cardiac glucose transport. J Nucl Cardiol. 2000;7(3):267-276. doi: 10.1016/s1071-3581(00)70016-x
58. Young LH, Renfu Y, Russell R, et al. Low-flow ischemia leads to translocation of canine heart GLUT-4 and GLUT-1 glucose transporters to the sarcolemma in vivo. Circulation. 1997;95(2):415-422. doi: 10.1161/01.cir.95.2.415
59. Brosius FC 3rd, Nguyen N, Egert S, et al. Increased sarcolemmal glucose transporter abundance in myocardial ischemia. Am J Cardiol. 1997;80(3A):77A-84A. doi: 10.1016/s0002-9149(97)00460-8
60. Apstein CS, Opie LH. A challenge to the metabolic approach to myocardial ischaemia. Eur Heart J. 2005;26(10):956-959. doi: 10.1093/eurheartj/ehi200
61. Selker HP, Beshansky JR, Griffith JL, et al. Study design for the immediate myocardial metabolic enhancement during initial assessment and treatment in emergency care (IMMEDIATE) trial: A double-blind randomized controlled trial of intravenous glucose, insulin, and potassium for acute coronary syndromes in emergency medical services. Am Heart J. 2012;163(3):315-322. doi: 10.1016/j.ahj.2012.02.002
62. Selker HP, Udelson JE, Massaro JM, et al. One-year outcomes of out-of-hospital administration of intravenous glucose, insulin, and potassium (GIK) in patients with suspected acute coronary syndromes (from the IMMEDIATE [Immediate myocardial metabolic enhancement during initial assessment and treatment in emergency care] trial). Am J Cardiol. 2014;113(10):1599-1605. doi: 10.1016/j.amjcard.2014.02.010
63. Wahlstrom KL, Hansen HF, Kvist M, et al. Effect of remote Ischaemic preconditioning on perioperative endothelial dysfunction in non-cardiac surgery: A randomised clinical trial. Cells. 2023;12(6):911. doi: 10.3390/cells12060911
64. Kakavand H, Saadatagah S, Naderian M, et al. Evaluating the role of intravenous pentoxifylline administration on primary percutaneous coronary intervention success rate in patients with ST-elevation myocardial infarction (PENTOS-PCI). Naunyn Schmiedebergs Arch Pharmacol. 2023;396(3):557-565. doi: 10.1007/s00210-022-02368-3
65. Frey N, Katus HA, Olson EN, Hill JA. Hypertrophy of the heart: A new therapeutic target? Circulation. 2004;109(13):1580-1589. doi: 10.1161/01.CIR.0000120390.68287.BB
66. Meerson FZ. On the mechanism of compensatory hyperfunction and insufficiency of the heart. Cor Vasa. 1961;3:161-177.
67. Sandler H, Dodge HT. Left ventricular tension and stress in man. Circ Res. 1963;13:91-104. doi: 10.1161/01.res.13.2.91
68. Hood WP Jr., Rackley CE, Rolett EL. Wall stress in the normal and hypertrophied human left ventricle. Am J Cardiol. 1968;22(4):550-558. doi: 10.1016/0002-9149(68)90161-6
69. Grossman W, Jones D, McLaurin LP. Wall stress and patterns of hypertrophy in the human left ventricle. J Clin Invest. 1975;56(1):56-64. doi: 10.1172/JCI108079
70. Levy D, Garrison RJ, Savage DD, Kannel WB, Castelli WP. Prognostic implications of echocardiographically determined left ventricular mass in the Framingham Heart Study. N Engl J Med. 1990;322(22):1561-1566. doi: 10.1056/NEJM199005313222203
71. Koren MJ, Devereux RB, Casale PN, Savage DD, Laragh JH. Relation of left ventricular mass and geometry to morbidity and mortality in uncomplicated essential hypertension. Ann Intern Med. 1991;114(5):345-352. doi: 10.7326/0003-4819-114-5-345
72. Christe ME, Rodgers RL. Altered glucose and fatty acid oxidation in hearts of the spontaneously hypertensive rat. J Mol Cell Cardiol. 1994;26(10):1371-1375. doi: 10.1006/jmcc.1994.1155
73. Zhang YB, Meng YH, Chang S, Zhang RY, Shi C. High fructose causes cardiac hypertrophy via mitochondrial signaling pathway. Am J Transl Res. 2016;8(11):4869-4880.
74. Lionetti V, Stanley WC, Recchia FA. Modulating fatty acid oxidation in heart failure. Cardiovasc Res. 2011;90(2):202-209. doi: 10.1093/cvr/cvr038
75. Neubauer S. The failing heart--an engine out of fuel. N Engl J Med. 2007;356(11):1140-1151. doi: 10.1056/NEJMra063052
76. Beer M, Seyfarth T, Sandstede J, et al. Absolute concentrations of high-energy phosphate metabolites in normal, hypertrophied, and failing human myocardium measured noninvasively with (31) P-SLOOP magnetic resonance spectroscopy. J Am Coll Cardiol. 2002;40(7):1267-1274. doi: 10.1016/s0735-1097(02)02160-5
77. Neubauer S, Remkes H, Spindler M, et al. Downregulation of the Na(+)-creatine cotransporter in failing human myocardium and in experimental heart failure. Circulation. 1999;100(18):1847-1850. doi: 10.1161/01.cir.100.18.1847
78. Kato T, Niizuma S, Inuzuka Y, et al. Analysis of metabolic remodeling in compensated left ventricular hypertrophy and heart failure. Circ Heart Fail. 2010;3(3):420-430. doi: 10.1161/CIRCHEARTFAILURE.109.888479
79. Degens H, de Brouwer KF, Gilde AJ, et al. Cardiac fatty acid metabolism is preserved in the compensated hypertrophic rat heart. Basic Res Cardiol. 2006;101(1):17-26. doi: 10.1007/s00395-005-0549-0
80. Akki A, Smith K, Seymour AM. Compensated cardiac hypertrophy is characterised by a decline in palmitate oxidation. Mol Cell Biochem. 2008;311(1-2):215-224. doi: 10.1007/s11010-008-9711-y
81. Dai DF, Hsieh EJ, Liu Y, et al. Mitochondrial proteome remodelling in pressure overload-induced heart failure: The role of mitochondrial oxidative stress. Cardiovasc Res. 2012;93(1):79-88. doi: 10.1093/cvr/cvr274
82. Berthiaume JM, Young ME, Chen X, McElfresh TA, Yu X, Chandler MP. Normalizing the metabolic phenotype after myocardial infarction: Impact of subchronic high fat feeding. J Mol Cell Cardiol. 2012;53(1):125-133. doi: 10.1016/j.yjmcc.2012.04.005
83. Berthiaume JM, Bray MS, McElfresh TA, et al. The myocardial contractile response to physiological stress improves with high saturated fat feeding in heart failure. Am J Physiol Heart Circ Physiol. 2010;299(2):H410-H421. doi: 10.1152/ajpheart.00270.2010
84. Reid IR, Birstow SM, Bolland MJ, Reid IR, Birstow SM, Bolland MJ. Calcium and cardiovascular disease. Endocrinol Metab (Seoul). 2017;32(3):339-349. doi: 10.3803/EnM.2017.32.3.339
85. Sutanto H, Lyon A, Lumens J, Schotten U, Dobrev D, Heijman J. Cardiomyocyte calcium handling in health and disease: Insights from in vitro and in silico studies. Prog Biophys Mol Biol. 2020;157:54-75. doi: 10.1016/j.pbiomolbio.2020.02.008