Mitochondria: The master regulator of aging
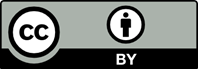
Mitochondria are ATP-producing organelles in eukaryotic organisms that serve as the cell’s power plants. Besides, mitochondria are integral to regulating cellular homeostasis and metabolism as a result of their essential roles in reactive oxygen species (ROS) production, bioenergetics, catabolism and anabolism, heme and iron-sulfur biosynthesis, iron and calcium homeostasis, apoptosis and signal transduction, as well as immunity and inflammation. It is well accepted that mitochondria are evolutionarily derived from endosymbiotic alphaproteobacteria within eukaryotic cells adapted for effective energy transduction. Although most of the mitochondrial DNA (mtDNA) is thought to have been transported to the eukaryotic nucleus during evolution, mitochondria may have preserved protein-coding genes within their own DNA. Accumulating data show that a progressive decline of mitochondria regulates aging. The present review aims to outline the role of mitochondria in various aspects of aging, including unfolded protein response, generation of ROS, and the contribution of somatic mtDNA mutations as well as inflammation in aging. Moreover, we propose mitochondria-targeted nanoparticles and mitochondrial genome editing as novel tools to modify mitochondrial genome aberrations.
- Flatt TJ. A new definition of aging? Front Genet. 2012;3:148. doi: 10.3389/fgene.2012.00148
- Liguori I, Russo G, Curcio F, et al. Oxidative stress, aging, and diseases. Clin Interv Aging. 2018:757-772. doi: 10.2147/cia.s158513
- López-Otín C, Blasco MA, Partridge L, Serrano M, Kroemer G. The hallmarks of aging. Cell. 2013;153(6):1194-1217. doi: 10.1016/j.cell.2013.05.039
- Petersen KF, Befroy D, Dufour S, et al. Mitochondrial dysfunction in the elderly: Possible role in insulin resistance. Science. 2003;300(5622):1140-1142. doi: 10.1126/science.1082889
- Pagiatakis C, Musolino E, Gornati R, Bernardini G, Papait R. Epigenetics of aging and disease: A brief overview. Aging Clin Exp Res. 2021;33:737-745. doi: 10.1007/s40520-019-01430-0
- Zhang S, Zhu N, Gu J, et al. Crosstalk between lipid rafts and aging: New frontiers for delaying aging. Aging Dis. 2022;13(4):1042. doi: 10.14336/ad.2022.0116
- Roger AJ, Muñoz-Gómez SA, Kamikawa R. The origin and diversification of mitochondria. Curr Biol. 2017;27(21):R1177-R1192. doi: 10.1016/j.cub.2017.09.015
- Cavalier-Smith T. Origin of mitochondria by intracellular enslavement of a photosynthetic purple bacterium. Proc Biol Sci. 2006;273(1596):1943-1952. doi: 10.1098/rspb.2006.3531
- Brown WM, George M Jr., Wilson AC. Rapid evolution of animal mitochondrial DNA. Proc Natl Acad Sci U S A. 1979;76(4):1967-1971. doi: 10.1073/pnas.76.4.1967
- Lax NZ, Turnbull DM, Reeve AK. Mitochondrial mutations: Newly discovered players in neuronal degeneration. Neuroscientist. 2011;17(6):645-658. doi: 10.1177/1073858411385469
- Ng MYW, Wai T, Simonsen A. Quality control of the mitochondrion. Dev Cell. 2021;56(7):881-905. doi: 10.1016/j.devcel.2021.02.009
- Patananan AN, Sercel AJ, Teitell MA. More than a powerplant: The influence of mitochondrial transfer on the epigenome. Curr Opin Physiol. 2018;3:16-24. doi: 10.1016/j.cophys.2017.11.006
- Ryan MT, Hoogenraad NJ. Mitochondrial-nuclear communications. Annu Rev Biochem. 2007;76:701-722. doi: 10.1146/annurev.biochem.76.052305.091720
- Singh B, Modica-Napolitano JS, Singh KK. Defining the Momiome: Promiscuous Information Transfer by Mobile Mitochondria and the Mitochondrial Genome. Netherlands: Elsevier; 2017. p. 1-17. doi: 10.1016/j.semcancer.2017.05.004
- Pugh TD, Conklin MW, Evans TD, et al. A shift in energy metabolism anticipates the onset of sarcopenia in rhesus monkeys. Aging Cell. 2013;12(4):672-681. doi: 10.1111/acel.12091
- Nunnari J, Suomalainen A. Mitochondria: In sickness and in health. Cell. 2012;148(6):1145-1159. doi: 10.1016/j.cell.2012.02.035
- Giles RE, Blanc H, Cann HM, Wallace DC. Maternal inheritance of human mitochondrial DNA. Proc Natl Acad Sci U S A. 1980;77(11):6715-6719. doi: 10.1073/pnas.77.11.6715
- Wallace DC, Chalkia D. Mitochondrial DNA genetics and the heteroplasmy conundrum in evolution and disease. Cold Spring Harb Perspect Biol. 2013;5(11):a021220. doi: 10.1101/cshperspect.a021220
- Gyllensten U, Wharton D, Josefsson A, Wilson AC. Paternal inheritance of mitochondrial DNA in mice. Nature. 1991;352(6332):255-257. doi: 10.1038/352255a0
- Schwartz M, Vissing J. Paternal inheritance of mitochondrial DNA. N Engl J Med. 2002;347(8):576-580. doi: 10.1056/nejmoa020350
- Kvist L, Martens J, Nazarenko AA, Orell M. Paternal leakage of mitochondrial DNA in the great tit (Parus major). Mol Biol Evol. 2003;20(2):243-247. doi: 10.1093/molbev/msg025
- Satoh M, Kuroiwa T. Organization of multiple nucleoids and DNA molecules in mitochondria of a human cell. Exp Cell Res. 1991;196(1):137-140. doi: 10.1016/0014-4827(91)90467-9
- Lee SR, Han J. Mitochondrial nucleoid: Shield and switch of the mitochondrial genome. Oxid Med Cell Longev. 2017;2017:8060949. doi: 10.1155/2017/8060949
- Legros F, Malka F, Frachon P, Lombès A, Rojo M. Organization and dynamics of human mitochondrial DNA. J Cell Sci. 2004;117(13):2653-2662. doi: 10.1242/jcs.01134
- Kukat C, Wurm CA, Spåhr H, Falkenberg M, Larsson NG, Jakobs S. Super-resolution microscopy reveals that mammalian mitochondrial nucleoids have a uniform size and frequently contain a single copy of mtDNA. Proc Natl Acad Sci. 2011;108(33):13534-13539. doi: 10.1073/pnas.1109263108
- Sasaki T, Sato Y, Higashiyama T, Sasaki N. Live imaging reveals the dynamics and regulation of mitochondrial nucleoids during the cell cycle in Fucci2-HeLa cells. Sci Rep. 2017;7(1):11257. doi: 10.1038/s41598-017-10843-8
- Iborra FJ, Kimura H, Cook P. The functional organization of mitochondrial genomes in human cells. BMC Biol. 2004;2:9. doi: 10.1186/1741-7007-2-9
- Tauber J, Dlasková A, Šantorová J, et al. Distribution of mitochondrial nucleoids upon mitochondrial network fragmentation and network reintegration in HEPG2 cells. Int J Biochem Cell Biol. 2013;45(3):593-603. doi: 10.1016/j.biocel.2012.11.019
- Jajoo R, Jung Y, Huh D, et al. Accurate concentration control of mitochondria and nucleoids. Science. 2016;351(6269):169-172. doi: 10.1126/science.aaa8714
- Rajala N, Gerhold JM, Martinsson P, Klymov A, Spelbrink JN. Replication factors transiently associate with mtDNA at the mitochondrial inner membrane to facilitate replication. Nucleic Acids Res. 2013;42(2):952-967. doi: 10.1093/nar/gkt988
- Garrido N, Griparic L, Jokitalo E, Wartiovaara J, van der Bliek AM, Spelbrink JN. Composition and dynamics of human mitochondrial nucleoids. Mol Biol Cell. 2003;14(4):1583-1596. doi: 10.1091/mbc.e02-07-0399
- Bouda E, Stapon A, Garcia‐Diaz M. Mechanisms of mammalian mitochondrial transcription. Protein Sci. 2019;28(9):1594-1605. doi: 10.1002/pro.3688
- Yu R, Lendahl U, Nistér M, Zhao J. Regulation of mammalian mitochondrial dynamics: Opportunities and challenges. Front Endocrinol (Lausanne). 2020;11:374. doi: 10.3389/fendo.2020.00374
- Bulthuis EP, Adjobo-Hermans MJW, Willems PHGM, Koopman WJH. Mitochondrial morphofunction in mammalian cells. Antioxid Redox Signal. 2019;30:2066-2109. doi: 10.1089/ars.2018.7534
- Stojanovski D, Johnston AJ, Streimann I, Hoogenraad NJ, Ryan MT. Import of nuclear-encoded proteins into mitochondria. Exp Physiol. 2003;88(1):57-64. doi: 10.1113/eph8802501
- Walker BR, Moraes CT. Nuclear-mitochondrial interactions. Biomolecules. 2022;12(3):427. doi: 10.3390/biom12030427
- Shokolenko IN, Alexeyev MF. Mitochondrial transcription in mammalian cells. Front Biosci (Landmark Ed). 2017;22:835. doi: 10.2741/4520
- Wallace DC. A mitochondrial paradigm of metabolic and degenerative diseases, aging, and cancer: A dawn for evolutionary medicine. Annu Rev Genet. 2005;39:359-407. doi: 10.1146/annurev.genet.39.110304.095751
- Taanman JW. The mitochondrial genome: Structure, transcription, translation and replication. Biochim Biophys Acta. 1999;1410(2):103-123. doi: 10.1016/s0005-2728(98)00161-3
- Chan SSL, Copeland WC. DNA polymerase gamma and mitochondrial disease: Understanding the consequence of POLG mutations. Biochim Biophys Acta. 2009;1787(5):312-319. doi: 10.1016/j.bbabio.2008.10.007
- Asahara H, Li Y, Fuss J, et al. Stimulation of human DNA polymerase ϵ by MDM2. Nucleic Acids Res. 2003;31(9):2451-2459. doi: 10.1093/nar/gkg342
- Shcherbakova PV, Bebenek K, Kunkel TA. Functions of eukaryotic DNA polymerases. Sci Aging Knowledge Environ. 2003;2003(8):RE3. doi: 10.1126/sageke.2003.8.re3
- Druzhyna NM, Wilson GL, LeDoux SP. Mitochondrial DNA repair in aging and disease. Mech Ageing Dev. 2008;129(7-8):383-390. doi: 10.1016/j.mad.2008.03.002
- Yakes FM, Van Houten B. Mitochondrial DNA damage is more extensive and persists longer than nuclear DNA damage in human cells following oxidative stress. Proc Natl Acad Sci U S A. 1997;94(2):514-519. doi: 10.1073/pnas.94.2.514
- Ide T, Tsutsui H, Hayashidani S, et al. Mitochondrial DNA damage and dysfunction associated with oxidative stress in failing hearts after myocardial infarction. Circ Res. 2001;88(5):529-535. doi: 10.1161/01.res.88.5.529
- Larsen NB, Rasmussen M, Rasmussen LJ. Nuclear and mitochondrial DNA repair: Similar pathways? Mitochondrion 2005;5(2):89-108. doi: 10.1016/j.mito.2005.02.002
- Bohr VA, Dianov GL. Oxidative DNA damage processing in nuclear and mitochondrial DNA. Biochimie. 1999;81(1-2):155-160. doi: 10.1016/S0300-9084(99)80048-0
- Hamilton ML, Van Remmen H, Drake JA, et al. Does oxidative damage to DNA increase with age? Proc Natl Acad Sci U S A. 2001;98(18):10469-10474. doi: 10.1073/pnas.171202698
- Catalán M, Olmedo I, Faúndez J, Jara JA. Medicinal chemistry targeting mitochondria: From new vehicles and pharmacophore groups to old drugs with mitochondrial activity. Int J Mol Sci. 2020;21(22):8684. doi: 10.3390/ijms21228684
- Singer TP, Ramsay RR. Mechanism of the neurotoxicity of MPTP: An update. FEBS Lett. 1990;274(1-2):1-8. doi: 10.1016/0014-5793(90)81315-f
- Cho H, Cho YY, Shim MS, Lee JY, Lee HS, Kang HC. Mitochondria-targeted drug delivery in cancers. Biochim Biophys Acta Mol Basis Dis. 2020;1866(8):165808. doi: 10.1016/j.bbadis.2020.165808
- Filograna R, Mennuni M, Alsina D, Larsson NG. Mitochondrial DNA copy number in human disease: The more the better? FEBS Lett. 2021;595(8):976-1002. doi: 10.1002/1873-3468.14021
- Kong M, Guo L, Xu W, et al. Aging-associated accumulation of mitochondrial DNA mutations in tumor origin. Life Med. 2022;1(2):149-167. doi: 10.1093/lifemedi/lnac014
- Rossignol R, Malgat M, Mazat JP, Letellier T. Threshold effect and tissue specificity: Implication for mitochondrial cytopathies. J Biol Chem. 1999;274(47):33426-33432. doi: 10.1074/jbc.274.47.33426
- Rossignol R, Faustin B, Rocher C, Malgat M, Mazat JP, Letellier T. Mitochondrial threshold effects. Biochem J. 2003;370(3):751-762. doi: 10.1042/bj20021594
- Sanchez-Contreras M, Kennedy SR. The complicated nature of somatic mtDNA mutations in aging. Front Aging. 2022;2:805126. doi: 10.3389/fragi.2021.805126
- Stewart JB, Chinnery PF. The dynamics of mitochondrial DNA heteroplasmy: Implications for human health and disease. Nat Rev Genet. 2015;16(9):530-542. doi: 10.1038/nrg3966
- Filograna R, Koolmeister C, Upadhyay M, et al. Modulation of mtDNA copy number ameliorates the pathological consequences of a heteroplasmic mtDNA mutation in the mouse. Sci Adv. 2019;5(4):eaav9824. doi: 10.1126/sciadv.aav9824
- Mancuso M, Orsucci D, Angelini C, et al. The m. 3243A> G mitochondrial DNA mutation and related phenotypes. A matter of gender? J Neurol. 2014;261:504-510. doi: 10.1007/s00415-013-7225-3
- Li D, Liang C, Zhang T, et al. Pathogenic mitochondrial DNA 3243A> G mutation: From genetics to phenotype. Front Genet. 2022;13:951185. doi: 10.3389/fgene.2022.951185
- Goto Y, Nonaka I, Horai S. A mutation in the tRNALeu (UUR) gene associated with the MELAS subgroup of mitochondrial encephalomyopathies. Nature. 1990;348(6302):651-653. doi: 10.1038/348651a0
- Arbeithuber B, Cremona MA, Hester J, et al. Advanced age increases frequencies of de novo mitochondrial mutations in macaque oocytes and somatic tissues. Proc Natl Acad Sci U S A. 2022;119(15):e2118740119. doi: 10.1073/pnas.2118740119
- Cortopassi GA, Arnheim N. Detection of a specific mitochondrial DNA deletion in tissues of older humans. Nucleic Acids Res. 1990;18(23):6927-6933. doi: 10.1093/nar/18.23.6927
- Pikó L, Hougham AJ, Bulpitt KJ. Studies of sequence heterogeneity of mitochondrial DNA from rat and mouse tissues: Evidence for an increased frequency of deletions/ additions with aging. Mech Ageing Dev. 1988;43(3):279-293. doi: 10.1016/0047-6374(88)90037-1
- Khrapko K, Vijg J. Mitochondrial DNA mutations and aging: Devils in the details? Trends Genet. 2009;25(2):91-98. doi: 10.1016/j.tig.2008.11.007
- Kujoth GC, Hiona A, Pugh TD, et al. Mitochondrial DNA mutations, oxidative stress, and apoptosis in mammalian aging. Science. 2005;309(5733):481-484. doi: 10.1126/science.1112125
- Trifunovic A, Wredenberg A, Falkenberg M, et al. Premature ageing in mice expressing defective mitochondrial DNA polymerase. Nature. 2004;429(6990):417-423. doi: 10.1038/nature02517
- Bailey LJ, Cluett TJ, Reyes A, et al. Mice expressing an error-prone DNA polymerase in mitochondria display elevated replication pausing and chromosomal breakage at fragile sites of mitochondrial DNA. Nucleic Acids Res. 2009;37(7):2327-2335. doi: 10.1093/nar/gkp091
- Williams SL, Huang J, Edwards YJK, et al. The mtDNA mutation spectrum of the progeroid Polg mutator mouse includes abundant control region multimers. Cell Metab. 2010;12(6):675-682. doi: 10.1016/j.cmet.2010.11.012
- Harman D. Aging: A theory based on free radical and radiation chemistry. J Gerontol. 1956;11(3):298-300. doi: 10.1093/geronj/11.3.298
- Bandy B, Davison AJ. Mitochondrial mutations may increase oxidative stress: Implications for carcinogenesis and aging? Free Radic Biol Med. 1990;8(6):523-539. doi: 10.1016/0891-5849(90)90152-9
- Hiona A, Leeuwenburgh C. The role of mitochondrial DNA mutations in aging and sarcopenia: Implications for the mitochondrial vicious cycle theory of aging. Exp Gerontol. 2008;43(1):24-33. doi: 10.1016/j.exger.2007.10.001
- Alexeyev MF, Ledoux SP, Wilson GL. Mitochondrial DNA and aging. Clin Sci (Lond). 2004;107(4):355-364. doi: 10.1042/cs20040148
- Blagosklonny MV. Aging: Ros or tor. Cell Cycle. 2008;7(21):3344-3354. doi: 10.4161/cc.7.21.6965
- Cabreiro F, Ackerman D, Doonan R, et al. Increased life span from overexpression of superoxide dismutase in Caenorhabditis elegans is not caused by decreased oxidative damage. Free Radic Biol Med. 2011;51(8):1575-1582. doi: 10.1016/j.freeradbiomed.2011.07.020
- Gems D, Doonan R. Antioxidant defense and aging in C. elegans: Is the oxidative damage theory of aging wrong? Cell Cycle. 2009;8(11):1681-1687. doi: 10.4161/cc.8.11.8595
- Koc A, Gasch AP, Rutherford JC, Kim HY, Gladyshev VN. Methionine sulfoxide reductase regulation of yeast lifespan reveals reactive oxygen species-dependent and-independent components of aging. Proc Natl Acad Sci U S A. 2004;101(21):7999-8004. doi: 10.1073/pnas.0307929101
- Lapointe J, Hekimi S. When a theory of aging ages badly. Cell Mol Life Sci. 2010;67:1-8. doi: 10.1007/s00018-009-0138-8
- Mockett RJ, Sohal BH, Sohal RS. Expression of multiple copies of mitochondrially targeted catalase or genomic Mn superoxide dismutase transgenes does not extend the life span of Drosophila melanogaster. Free Radic Biol Med. 2010;49(12):2028-2031. doi: 10.1016/j.freeradbiomed.2010.09.029
- Pérez VI, Van Remmen H, Bokov A, Epstein CJ, Vijg J, Richardson A. The overexpression of major antioxidant enzymes does not extend the lifespan of mice. Aging Cell. 2009;8(1):73-75. doi: 10.15252/emmm.201708084
- Speakman JR, Selman C. The free‐radical damage theory: Accumulating evidence against a simple link of oxidative stress to ageing and lifespan. Bioessays. 2011;33(4):255-259. doi: 10.1002/bies.201000132
- Van Raamsdonk JM, Hekimi S. Superoxide dismutase is dispensable for normal animal lifespan. Proc Natl Acad Sci U S A. 2012;109(15):5785-5790. doi: 10.1073/pnas.1116158109
- Ristow M, Schmeisser S. Extending life span by increasing oxidative stress. Free Radic Biol Med. 2011;51(2):327-336. doi: 10.1016/j.freeradbiomed.2011.05.010
- Zarse K, Schmeisser S, Groth M, et al. Impaired insulin/IGF1 signaling extends life span by promoting mitochondrial L-proline catabolism to induce a transient ROS signal. Cell Metab. 2012;15(4):451-465. doi: 10.1016/j.cmet.2012.02.013
- Katayama M, Tanaka M, Yamamoto H, Ohbayashi T, Nimura Y, Ozawa T. Deleted mitochondrial DNA in the skeletal muscle of aged individuals. Biochem Int. 1991;25(1):47-56. doi: 10.1016/j.mito.2005.02.002
- Brierley EJ, Johnson MA, Lightowlers RN, James OF, Turnbull DM. Role of mitochondrial DNA mutations in human aging: Implications for the central nervous system and muscle. Ann Neurol. 1998;43(2):217-223. doi: 10.1002/ana.410430212
- Trifunovic A, Hansson A, Wredenberg A, et al. Somatic mtDNA mutations cause aging phenotypes without affecting reactive oxygen species production. Proc Natl Acad Sci U S A. 2005;102(50):17993-17998. doi: 10.1073/pnas.0508886102
- Shokolenko I, Venediktova N, Bochkareva A, Wilson GL, Alexeyev MF. Oxidative stress induces degradation of mitochondrial DNA. Nucleic Acids Res. 2009;37(8):2539-2548. doi: 10.1093/nar/gkp100
- Kennedy SR, Salk JJ, Schmitt MW, Loeb LA. Ultra-sensitive sequencing reveals an age-related increase in somatic mitochondrial mutations that are inconsistent with oxidative damage. PLoS Genet. 2013;9(9):e1003794. doi: 10.1371/journal.pgen.1003794
- Arbeithuber B, Hester J, Cremona MA, et al. Age-related accumulation of de novo mitochondrial mutations in mammalian oocytes and somatic tissues. PLoS Biol. 2020;18(7):e3000745. doi: 10.1371/journal.pbio.3000745
- Li H, Shen L, Hu P, et al. Aging-associated mitochondrial DNA mutations alter oxidative phosphorylation machinery and cause mitochondrial dysfunctions. Biochim Biophys Acta Mol Basis Dis. 2017;1863(9):2266-2273. doi: 10.1016/j.bbadis.2017.05.022
- Schroeder P, Gremmel T, Berneburg M, Krutmann J. Partial depletion of mitochondrial DNA from human skin fibroblasts induces a gene expression profile reminiscent of photoaged skin. J Invest Dermatol. 2008;128(9):2297-2303. doi: 10.1038/jid.2008.57
- Fukui H, Moraes CT. Mechanisms of formation and accumulation of mitochondrial DNA deletions in aging neurons. Hum Mol Genet. 2009;18(6):1028-1036. doi: 10.1093/hmg/ddn437
- Moreno-Gonzalez I, Soto C. Misfolded protein aggregates: Mechanisms, structures and potential for disease transmission. Semin Cell Dev Biol 2011;22:482-7.
- Haynes CM, Ron D. The mitochondrial UPR-protecting organelle protein homeostasis. J Cell Sci. 2010;123(22):3849-3855. doi: 10.1242/jcs.075119
- Pellegrino MW, Nargund AM, Haynes CM. Signaling the mitochondrial unfolded protein response. Biochim Biophys Acta. 2013;1833(2):410-416. doi: 10.1016/j.bbamcr.2012.02.019
- Bukau B, Weissman J, Horwich A. Molecular chaperones and protein quality control. Cell. 2006;125(3):443-451. doi: 10.1016/j.cell.2006.04.014
- Chacinska A, Koehler CM, Milenkovic D, Lithgow T, Pfanner N. Importing mitochondrial proteins: Machineries and mechanisms. Cell. 2009;138(4):628-644. doi: 10.1016/j.cell.2009.08.005
- Bie AS, Cömert C, Körner R, et al. An inventory of interactors of the human HSP60/HSP10 chaperonin in the mitochondrial matrix space. Cell Stress Chaperones. 2020;25:407-416. doi: 10.1007/s12192-020-01080-6
- Zhou C, Sun H, Zheng C, et al. Oncogenic HSP60 regulates mitochondrial oxidative phosphorylation to support Erk1/2 activation during pancreatic cancer cell growth. Cell Death Dis. 2018;9(2):161. doi: 10.1038/s41419-017-0196-z
- Craig EA. Hsp70 at the membrane: Driving protein translocation. BMC Biol. 2018;16(1):11. doi: 10.1186/s12915-017-0474-3
- Scherer PE, Krieg UC, Hwang ST, Vestweber D, Schatz G. A precursor protein partly translocated into yeast mitochondria is bound to a 70 kd mitochondrial stress protein. EMBO J. 1990;9(13):4315-4322. doi: 10.1002/j.1460-2075.1990.tb07880.x
- Ostermann J, Voos W, Kang PJ, Craig EA, Neupert W, Pfanner N. Precursor proteins in transit through mitochondrial contact sites interact with hsp70 in the matrix. FEBS Lett. 1990;277(1-2):281-284. doi: 10.1016/0014-5793(90)80865-g
- Liu Q, Krzewska J, Liberek K, Craig EA. Mitochondrial Hsp70 Ssc1: Role in protein folding. J Biol Chem. 2001;276(9):6112-6118. doi: 10.1074/jbc.m009519200
- Pimenta de Castro I, Costa A, Lam D, et al. Genetic analysis of mitochondrial protein misfolding in Drosophila melanogaster. Cell Death Differ. 2012;19(8):1308-1316. doi: 10.1038/cdd.2012.5
- Nargund AM, Pellegrino MW, Fiorese CJ, Baker BM, Haynes CM. Mitochondrial import efficiency of ATFS-1 regulates mitochondrial UPR activation. Science. 2012;337(6094):587-590. doi: 10.1126/science.1223560
- Qureshi MA, Haynes CM, Pellegrino M. The mitochondrial unfolded protein response: Signaling from the powerhouse. J Biol Chem. 2017;292(33):13500-13506. doi: 10.1074/jbc.r117.791061
- Runkel ED, Liu S, Baumeister R, Schulze E. Surveillance-activated defenses block the ROS–induced mitochondrial unfolded protein response. PLoS Genet. 2013;9(3):e1003346. doi: 10.1371/journal.pgen.1003346
- Fiorese CJ, Schulz AM, Lin YF, Rosin N, Pellegrino MW, Haynes CM. The transcription factor ATF5 mediates a mammalian mitochondrial UPR. Curr Biol. 2016;26(15):2037-2043. doi: 10.1016/j.cub.2016.06.002
- Gao K, Li Y, Hu S, Liu Y. SUMO peptidase ULP-4 regulates mitochondrial UPR-mediated innate immunity and lifespan extension. Elife. 2019;8:e41792. doi: 10.7554/elife.41792
- Haynes CM, Yang Y, Blais SP, Neubert TA, Ron D. The matrix peptide exporter HAF-1 signals a mitochondrial UPR by activating the transcription factor ZC376. 7 in C. elegans. Mol Cell. 2010;37(4):529-540. doi: 10.1016/j.molcel.2010.01.015
- Tran HC, Van Aken O. Mitochondrial unfolded protein-related responses across kingdoms: Similar problems, different regulators. Mitochondrion. 2020;53:166-177. doi: 10.1016/j.mito.2020.05.009
- Wrobel L, Topf U, Bragoszewski P, et al. Mistargeted mitochondrial proteins activate a proteostatic response in the cytosol. Nature. 2015;524(7566):485-488. doi: 10.1016/j.nbd.2009.08.009
- Benedetti C, Haynes CM, Yang Y, Harding HP, Ron D. Ubiquitin-like protein 5 positively regulates chaperone gene expression in the mitochondrial unfolded protein response. Genetics. 2006;174(1):229-239. doi: 10.1534/genetics.106.061580
- Jovaisaite V, Mouchiroud L, Auwerx J. The mitochondrial unfolded protein response, a conserved stress response pathway with implications in health and disease. J Exp Biol. 2014;217(1):137-143. doi: 10.1242/jeb.090738
- Muñoz-Carvajal F, Sanhueza M. The mitochondrial unfolded protein response: A hinge between healthy and pathological aging. Front Aging Neurosci. 2020;12:581849. doi: 10.3389/fnagi.2020.581849
- Mouchiroud L, Houtkooper RH, Moullan N, et al. The NAD+/sirtuin pathway modulates longevity through activation of mitochondrial UPR and FOXO signaling. Cell. 2013;154(2):430-441. doi: 10.1016/j.cell.2013.06.016
- Dillin A, Hsu AL, Arantes-Oliveira N, et al. Rates of behavior and aging specified by mitochondrial function during development. Science. 2002;298(5602):2398-2401. doi: 10.3390/nu11092221
- Durieux J, Wolff S, Dillin A. The cell-non-autonomous nature of electron transport chain-mediated longevity. Cell. 2011;144(1):79-91. doi: 10.1016/j.cell.2010.12.016
- Han B, Sivaramakrishnan P, Lin CCJ, et al. Microbial genetic composition tunes host longevity. Cell. 2017;169(7):1249- 1262.e13. doi: 10.1016/j.cell.2017.05.036
- Wu Z, Senchuk MM, Dues DJ, et al. Mitochondrial unfolded protein response transcription factor ATFS-1 promotes longevity in a long-lived mitochondrial mutant through activation of stress response pathways. BMC Biol. 2018;16:147. doi: 10.1186/s12915-018-0615-3
- Tian Y, Garcia G, Bian Q, et al. Mitochondrial stress induces chromatin reorganization to promote longevity and UPRmt. Cell. 2016;165(5):1197-1208. doi: 10.1016/j.cell.2016.04.011
- Ono T, Kamimura N, Matsuhashi T, et al. The histone 3 lysine 9 methyltransferase inhibitor chaetocin improves prognosis in a rat model of high salt diet-induced heart failure. Sci Rep. 2017;7(1):39752. doi: 10.1038/srep39752
- Merkwirth C, Jovaisaite V, Durieux J, et al. Two conserved histone demethylases regulate mitochondrial stress-induced longevity. Cell. 2016;165(5):1209-1223. doi: 10.1016/j.cell.2016.04.012
- Weng H, Ma Y, Chen L, et al. A new vision of mitochondrial unfolded protein response to the sirtuin family. Curr Neuropharmacol. 2020;18(7):613-623. doi: 10.2174/1570159x18666200123165002
- Papa L, Germain D. SirT3 regulates the mitochondrial unfolded protein response. Mol Cell Biol. 2014;34(4):699-710. doi: 10.1128/mcb.01337-13
- Cerutti R, Pirinen E, Lamperti C, et al. NAD+-dependent activation of Sirt1 corrects the phenotype in a mouse model of mitochondrial disease. Cell Metab. 2014;19(6):1042-1049. doi: 10.1016/j.cmet.2014.04.001
- Cho EH. SIRT3 as a regulator of non-alcoholic fatty liver disease. J Lifestyle Med. 2014;4(2):80-85. doi: 10.15280/jlm.2014.4.2.80
- Schadel A, Fischer M. Measurement of regional cerebral blood flow and accentuation of the primary auditory cortex with single photon emission computed tomography. Arch Otorhinolaryngol. 1989;246:205-209. doi: 10.1007/bf00453663
- Chen MM, Li Y, Deng SL, et al. Mitochondrial function and reactive oxygen/nitrogen species in skeletal muscle. Front Cell Dev Biol. 2022;10:826981. doi: 10.3389/fcell.2022.826981
- Valko M, Leibfritz D, Moncol J, et al. Free radicals and antioxidants in normal physiological functions and human disease. Int J Biochem Cell Biol. 2007;39(1):44-84. doi: 10.1016/j.biocel.2006.07.001
- Ott M, Gogvadze V, Orrenius S, Zhivotovsky B. Mitochondria, oxidative stress and cell death. Apoptosis. 2007;12:913-922. doi: 10.1007/s10495-007-0756-2
- Fogg VC, Lanning NJ, MacKeigan JP. Mitochondria in cancer: At the crossroads of life and death. Chin J Cancer. 2011;30(8):526. doi: 10.5732/cjc.011.10018
- Milkovic L, Cipak Gasparovic A, Cindric M, Mouthuy PA, Zarkovic N. Short overview of ROS as cell function regulators and their implications in therapy concepts. Cells. 2019;8(8):793. doi: 10.3390/cells8080793
- Rubio K, Hernández-Cruz EY, Rogel-Ayala DG, et al. Nutriepigenomics in environmental-associated oxidative stress. Antioxidants (Basel). 2023;12(3):771. doi: 10.3390/antiox12030771
- Agnihotri PK, Murthy PS, Mukherjee SK. Effect of herbicide banvel on rabbit vaginal mucus membrane. Indian J Exp Biol. 1989;27(12):1090-1091.
- Mills EL, Kelly B, Logan A, et al. Succinate dehydrogenase supports metabolic repurposing of mitochondria to drive inflammatory macrophages. Cell. 2016;167(2):457-470.e13. doi: 10.1016/j.cell.2016.08.064
- Steinert RF, Grene RB. Postoperative management of epikeratoplasty. J Cataract Refract Surg. 1988;14(3):255-264. doi: 10.1016/s0886-3350(88)80113-5
- Chouchani ET, Pell VR, James AM, et al. A unifying mechanism for mitochondrial superoxide production during ischemia-reperfusion injury. Cell Metab. 2016;23(2):254-263. doi: 10.1016/j.cmet.2015.12.009
- Fujimoto Y. Clinical significance of nuclear DNA contents in prostate cancer. Nihon Gan Chiryo Gakkai Shi. 1988;23(6):1265-1276.
- Poillet-Perez L, Despouy G, Delage-Mourroux R, Boyer- Guittaut M. Interplay between ROS and autophagy in cancer cells, from tumor initiation to cancer therapy. Redox Biol. 2015;4:184-192. doi: 10.1016/j.redox.2014.12.003
- Powers SK, Ji LL, Kavazis AN, Jackson MJ. Reactive oxygen species: Impact on skeletal muscle. Compr Physiol. 2011;1(2):941. doi: 10.1002/cphy.c100054
- Venkataraman K, Khurana S, Tai TC. Oxidative stress in aging-matters of the heart and mind. Int J Mol Sci. 2013;14(9):17897-17925. doi: 10.3390/ijms140917897
- Salisbury D, Bronas U. Reactive oxygen and nitrogen species: Impact on endothelial dysfunction. Nurs Res. 2015;64(1):53-66. doi: 10.1097/nnr.0000000000000068
- Genestra M. Oxyl radicals, redox-sensitive signalling cascades and antioxidants. Cell Signal. 2007;19(9):1807-1819. doi: 10.1016/j.cellsig.2007.04.009
- Adams L, Franco MC, Estevez AG. Reactive nitrogen species in cellular signaling. Exp Biol Med. 2015;240(6):711-717. doi: 10.1177/1535370215581314
- Phaniendra A, Jestadi DB, Periyasamy L. Free radicals: Properties, sources, targets, and their implication in various diseases. Indian J Clin Biochem. 2015;30:11-26. doi: 10.1007/s12291-014-0446-0
- Frijhoff J, Winyard PG, Zarkovic N, et al. Clinical relevance of biomarkers of oxidative stress. Antioxid Redox Signal. 2015;23(14):1144-1170. doi: 10.1089/ars.2015.6317
- Birben E, Sahiner UM, Sackesen C, Erzurum S, Kalayci O. Oxidative stress and antioxidant defense. World Allergy Organ J. 2012;5:9-19. doi: 10.1097/wox.0b013e3182439613
- Wu JQ, Kosten TR, Zhang XY. Free radicals, antioxidant defense systems, and schizophrenia. Prog Neuropsychopharmacol Biol Psychiatry. 2013;46:200-206. doi: 10.1016/j.pnpbp.2013.02.015
- Pisoschi AM, Pop A. The role of antioxidants in the chemistry of oxidative stress: A review. Eur J Med Chem. 2015;97:55-74. doi: 10.1016/j.ejmech.2015.04.040
- Lü JM, Lin PH, Yao Q, Chen C. Chemical and molecular mechanisms of antioxidants: Experimental approaches and model systems. J Cell Mol Med. 2010;14(4):840-860. doi: 10.1111/j.1582-4934.2009.00897.x
- Skrzydlewska E, Augustyniak A, Michalak K, Farbiszewski R. Green tea supplementation in rats of different ages mitigates ethanol-induced changes in brain antioxidant abilities. Alcohol. 2005;37(2):89-98. doi: 10.1016/j.alcohol.2005.12.003
- Sawada M, Carlson JC. Changes in superoxide radical and lipid peroxide formation in the brain, heart and liver during the lifetime of the rat. Mech Ageing Dev. 1987;41(1-2):125-137. doi: 10.1016/0047-6374(87)90057-1
- Rao G, Xia E, Richardson A. Effect of age on the expression of antioxidant enzymes in male Fischer F344 rats. Mech Ageing Dev. 1990;53(1):49-60. doi: 10.1016/0047-6374(90)90033-c
- Murali G, Panneerselvam KS, Panneerselvam C. Age-associated alterations of lipofuscin, membrane-bound ATPases and intracellular calcium in cortex, striatum and hippocampus of rat brain: Protective role of glutathione monoester. Int J Dev Neurosci. 2008;26(2):211-215. doi: 10.1016/j.ijdevneu.2007.12.004
- Harman D. Free radical theory of aging. Mutat Res. 1992;275(3-6):257-266. doi: 10.1016/0921-8734(92)90030-S
- Siqueira IR, Fochesatto C, de Andrade A, et al. Total antioxidant capacity is impaired in different structures from aged rat brain. Int J Dev Neurosci. 2005;23(8):663-671. doi: 10.1016/j.ijdevneu.2005.03.001
- Akila VP, Harishchandra H, D’souza V, D’souza B. Age related changes in lipid peroxidation and antioxidants in elderly people. Indian J Clin Biochem. 2007;22:131-134. doi: 10.1007/bf02912896
- Rizvi SI, Maurya PK. Alterations in antioxidant enzymes during aging in humans. Mol Biotechnol. 2007;37:58-61. doi: 10.1007/s12033-007-0048-7
- Wei YH, Lu CY, Lee HC, Pang CY, Ma YS. Oxidative damage and mutation to mitochondrial dna and age‐dependent decline of mitochondrial respiratory function. Ann N Y Acad Sci. 1998;854(1):155-170. doi: 10.1111/j.1749-6632.1998.tb09899.x
- Ashrafian H, Czibik G, Bellahcene M, et al. Fumarate is cardioprotective via activation of the Nrf2 antioxidant pathway. Cell Metab. 2012;15(3):361-371. doi: 10.1016/j.cmet.2012.01.017
- Ryan TA, Hooftman A, Rehill AM, et al. Dimethyl fumarate and 4-octyl itaconate are anticoagulants that suppress tissue factor in macrophages via inhibition of type I interferon. Nat Commun. 2023;14(1):3513. doi: 10.1038/s41467-023-39174-1
- Ellrichmann G, Petrasch-Parwez E, Lee DH, et al. Efficacy of fumaric acid esters in the R6/2 and YAC128 models of Huntington’s disease. PLoS One. 2011;6(1):e16172. doi: 10.1371/journal.pone.0016172
- Hoyle C, Green JP, Allan SM, Brough D, Lemarchand E. Itaconate and fumarate derivatives inhibit priming and activation of the canonical NLRP3 inflammasome in macrophages. Immunology. 2022;165(4):460-480. doi: 10.1111/imm.13454
- Sharkus R, Thakkar R, Kolson DL, Constantinescu CS. Dimethyl fumarate as potential treatment for Alzheimer’s disease: Rationale and clinical trial design. Biomedicines. 2023;11(5):1387. doi: 10.3390/biomedicines11051387
- Scuderi SA, Ardizzone A, Paterniti I, Esposito E, Campolo M. Antioxidant and anti-inflammatory effect of Nrf2 inducer dimethyl fumarate in neurodegenerative diseases. Antioxidants (Basel). 2020;9(7):630. doi: 10.3390/antiox9070630
- Rosito M, Testi C, Parisi G, Cortese B, Baiocco P, Di Angelantonio S. Exploring the use of dimethyl fumarate as microglia modulator for neurodegenerative diseases treatment. Antioxidants (Basel). 2020;9(8):700. doi: 10.3390/antiox9080700
- Harrington LA, Harley CB. Effect of vitamin E on lifespan and reproduction in Caenorhabditis elegans. Mech Ageing Dev. 1988;43(1):71-78. doi: 10.1016/0047-6374(88)90098-x
- Navarro A, Gómez C, Sánchez-Pino MJ, et al. Vitamin E at high doses improves survival, neurological performance, and brain mitochondrial function in aging male mice. Am J Physiol Regul Integr Comp Physiol. 2005;289(5):R1392-R1399. doi: 10.1152/ajpregu.00834.2004
- Schaffer S, Müller WE, Eckert GP. Tocotrienols: Constitutional effects in aging and disease. J Nutr. 2005;135(2):151-154. doi: 10.1093/jn/135.2.151
- Collins JJ, Evason K, Kornfeld K. Pharmacology of delayed aging and extended lifespan of Caenorhabditis elegans. Exp Gerontol. 2006;41(10):1032-1039. doi: 10.1016/j.exger.2006.06.038
- Siler-Marsiglio KI, Pan Q, Paiva M, Madorsky I, Khurana NC, Heaton MB. Mitochondrially targeted vitamin E and vitamin E mitigate ethanol-mediated effects on cerebellar granule cell antioxidant defense systems. Brain Res. 2005;1052(2):202-211. doi: 10.1016/j.brainres.2005.06.030
- La Fata G, van Vliet N, Barnhoorn S, et al. Vitamin E supplementation reduces cellular loss in the brain of a premature aging mouse model. J Prev Alzheimers Dis. 2017;4(4):226-235. doi: 10.14283/jpad.2017.30
- Beal MF. Mitochondria, oxidative damage, and inflammation in Parkinson’s disease. Ann N Y Acad Sci. 2003;991(1):120-131. doi: 10.1111/j.1749-6632.2003.tb07470.x
- Ayunin Q, Miatmoko A, Soeratri W, Erawati T, Susanto J, Legowo D. Improving the anti-ageing activity of coenzyme Q10 through protransfersome-loaded emulgel. Sci Rep. 2022;12(1):906. doi: 10.1038/s41598-021-04708-4
- Hidalgo-Gutiérrez A, González-García P, Díaz-Casado ME, et al. Metabolic targets of coenzyme Q10 in mitochondria. Antioxidants (Basel). 2021;10(4):520. doi: 10.3390/antiox10040520
- Acosta MJ, Fonseca LV, Desbats MA, et al. Coenzyme Q biosynthesis in health and disease. Biochim Biophys Acta. 2016;1857(8):1079-1085. doi: 10.1016/j.bbabio.2016.03.036
- Díaz-Casado ME, Quiles JL, Barriocanal-Casado E, et al. The paradox of coenzyme Q10 in aging. Nutrients. 2019;11(9):2221. doi: 10.3390/nu11092221
- Muta‐Takada K, Terada T, Yamanishi H, et al. Coenzyme Q10 protects against oxidative stress‐induced cell death and enhances the synthesis of basement membrane components in dermal and epidermal cells. Biofactors. 2009;35(5):435-441. doi: 10.1002/biof.56
- Inui M, Ooe M, Fujii K, Matsunaka H, Yoshida M, Ichihashi M. Mechanisms of inhibitory effects of CoQ10 on UVB-induced wrinkle formation in vitro and in vivo. Biofactors. 2008;32(1-4):237-243. doi: 10.1002/biof.5520320128
- Bank G, Kagan D, Madhavi D. Coenzyme Q10: Clinical update and bioavailability. J Evid Based Complement Altern Med. 2011;16(2):129-137. doi: 10.1177/2156587211399438
- Alcázar-Fabra M, Trevisson E, Brea-Calvo G. Clinical syndromes associated with Coenzyme Q10 deficiency. Essays Biochem. 2018;62(3):377-398. doi: 10.1042/ebc20170107
- Desbats MA, Lunardi G, Doimo M, Trevisson E, Salviati L. Genetic bases and clinical manifestations of coenzyme Q 10 (CoQ 10) deficiency. J Inherit Metab Dis. 2015;38:145-156. doi: 10.1007/s10545-014-9749-9
- Quiles JL, Ochoa JJ, Huertas JR, Mataix J. Coenzyme Q supplementation protects from age-related DNA double-strand breaks and increases lifespan in rats fed on a PUFA-rich diet. Exp Gerontol. 2004;39(2):189-194. doi: 10.1016/j.exger.2003.10.002
- Tomasetti M, Alleva R, Collins AR. In vivo supplementation with coenzyme Q10 enhances the recovery of human lymphocytes from oxidative DNA damage. FASEB J. 2001;15(8):1425-1427. doi: 10.1096/fj.00-0694fje
- Lönnrot K, Alho H, Holm P, Lagerstedt A, Huhtala H. The effects of lifelong ubiquinone Q10 supplementation on the Q9 and Q10 tissue concentrations and life span of male rats and mice. IUBMB Life. 1998;44(4):727-737. doi: 10.1080/15216549800201772
- Asencio C, Rodríguez-Aguilera JC, Ruiz-Ferrer M, Vela J, Navas P. Silencing of ubiquinone biosynthesis genes extends life span in Caenorhabditis elegans. FASEB J. 2003;17(9):1135-1137. doi: 10.1096/fj.02-1022fje
- Larsen PL, Clarke CF. Extension of life-span in Caenorhabditis elegans by a diet lacking coenzyme Q. Science. 2002;295(5552):120-123. doi: 10.1126/science.1064653
- Zhou D, Shao L, Spitz DR. Reactive oxygen species in normal and tumor stem cells. Adv Cancer Res. 2014;22:1-67. doi: 10.1016/B978-0-12-420117-0.00001-3
- Chaudhari P, Ye Z, Jang YY. Roles of reactive oxygen species in the fate of stem cells. Antioxid Redox Signal. 2014;20(12):1881-1890. doi: 10.1089/ars.2012.4963
- Ezashi T, Das P, Roberts RM. Low O2 tensions and the prevention of differentiation of hES cells. Proc Natl Acad Sci. 2005;102(13):4783-4788. doi: 10.1073/pnas.0501283102
- Shyh-Chang N, Daley GQ, Cantley LC. Stem cell metabolism in tissue development and aging. Development. 2013;140(12):2535-2547. doi: 10.1242/dev.091777
- Sauer H, Wartenberg M. Reactive oxygen species as signaling molecules in cardiovascular differentiation of embryonic stem cells and tumor-induced angiogenesis. Antioxid Redox Signal. 2005;7(11-12):1423-1434. doi: 10.1089/ars.2005.7.1423
- Ahlqvist KJ, Hämäläinen RH, Yatsuga S, et al. Somatic progenitor cell vulnerability to mitochondrial DNA mutagenesis underlies progeroid phenotypes in Polg mutator mice. Cell Metab. 2012;15(1):100-109. doi: 10.1016/j.cmet.2011.11.012
- Chen ML, Logan TD, Hochberg ML, et al. Erythroid dysplasia, megaloblastic anemia, and impaired lymphopoiesis arising from mitochondrial dysfunction. Blood. 2009;114(19):4045-4053. doi: 10.1182/blood-2008-08-169474
- Hämäläinen RH, Ahlqvist KJ, Ellonen P, et al. mtDNA mutagenesis disrupts pluripotent stem cell function by altering redox signaling. Cell Rep. 2015;11(10):1614-1624. doi: 10.1016/j.celrep.2015.05.009
- Sun N, Youle RJ, Finkel T. The mitochondrial basis of aging. Mol Cell. 2016;61(5):654-666. doi: 10.1016/j.molcel.2016.01.028
- Frezza C. Mitochondrial metabolites: Undercover signalling molecules. Interface Focus. 2017;7(2):20160100. doi: 10.1098/rsfs.2016.0100
- Zhang H, Menzies KJ, Auwerx J. The role of mitochondria in stem cell fate and aging. Development. 2018;145(8):dev143420. doi: 10.1242/dev.143420
- Carey BW, Finley LW, Cross JR, Allis CD, Thompson CB. Intracellular α-ketoglutarate maintains the pluripotency of embryonic stem cells. Nature. 2015;518(7539):413-416. doi: 10.1038/nature13981
- McReynolds MR, Chellappa K, Baur JA. Age-related NAD+ decline. Exp Gerontol. 2020;134:110888. doi: 10.1016/j.exger.2020.110888
- Schultz MB, Sinclair DA. Why NAD+ declines during aging: It’s destroyed. Cell Metab. 2016;23(6):965-966. doi: 10.1016/j.cmet.2016.05.022
- Cantó C, Sauve AA, Bai P. Crosstalk between poly (ADP-ribose) polymerase and sirtuin enzymes. Mol Aspects Med. 2013;34(6):1168-1201. doi: 10.1016/j.mam.2013.01.004
- Petriti B, Williams PA, Lascaratos G, Chau KY, Garway- Heath DF. Neuroprotection in glaucoma: NAD+/NADH redox state as a potential biomarker and therapeutic target. Cells 2021;10(6):1402. doi: 10.3390/cells10061402
- Houtkooper RH, Pirinen E, Auwerx J. Sirtuins as regulators of metabolism and healthspan. Nat Rev Mol Cell Biol. 2012;13(4):225-238. doi: 10.1038/nrm3293
- Gomes AP, Price NL, Ling AJ, et al. Declining NAD+ induces a pseudohypoxic state disrupting nuclear-mitochondrial communication during aging. Cell. 2013;155(7):1624-1638. doi: 10.1016/j.cell.2013.11.037
- Imai S, Guarente L. It takes two to tango: NAD+ and sirtuins in aging/longevity control. NPJ Aging Mech Dis. 2016;2(1):16017. doi: 10.1038/npjamd.2016.17
- Stein LR, Imai SI. Specific ablation of Nampt in adult neural stem cells recapitulates their functional defects during aging. EMBO J. 2014;33(12):1321-1340. doi: 10.1002/embj.201386917
- Rajman L, Chwalek K, Sinclair DA. Therapeutic potential of NAD-boosting molecules: The in vivo evidence. Cell Metab. 2018;27(3):529-547. doi: 10.1016/j.cmet.2018.02.011
- Yoshino J, Mills KF, Yoon MJ, Imai SI. Nicotinamide mononucleotide, a key NAD+ intermediate, treats the pathophysiology of diet-and age-induced diabetes in mice. Cell Metab. 2011;14(4):528-536. doi: 10.1016/j.cmet.2011.08.014
- Lombard DB, Alt FW, Cheng HL, et al. Mammalian Sir2 homolog SIRT3 regulates global mitochondrial lysine acetylation. Mol Cell Biol. 2007;27(24):8807-8814.doi: 10.1128/mcb.01636-07
- Brown K, Xie S, Qiu X, et al. SIRT3 reverses aging-associated degeneration. Cell Rep. 2013;3(2):319-327. doi: 10.1016/j.celrep.2013.01.005
- Shahriyari L, Komarova NL. Symmetric vs. asymmetric stem cell divisions: An adaptation against cancer? PLoS One. 2013;8(10):e76195. doi: 10.1371/journal.pone.0076195
- Evano B, Khalilian S, Le Carrou G, Almouzni G, Tajbakhsh S. Dynamics of asymmetric and symmetric divisions of muscle stem cells in vivo and on artificial niches. Cell Rep. 2020;30(10):3195-3206.e7. doi: 10.1016/j.celrep.2020.01.097
- Casas Gimeno G, Paridaen JTML. The symmetry of neural stem cell and progenitor divisions in the vertebrate brain. Front Cell Dev Biol. 2022;10:885269. doi: 10.3389/fcell.2022.885269
- Yamashita YM, Yuan H, Cheng