Precision medicine in neurosurgery: The evolving role of theranostics
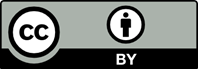
Theranostics in neurosurgery is a rapidly advancing field of precision medicine that combines diagnostic and therapeutic modalities to optimize patient outcomes. This approach has the potential to provide real-time feedback during therapy and diagnose a condition while simultaneously providing treatment. One such form of theranostics is focused ultrasound, which has been found to be effective in inducing neuroablation and neuromodulation and improving the efficacy of chemotherapy drugs by disrupting the blood–brain barrier. Targeted radionuclide therapy, which pairs positron emission tomography tracers with therapeutic effects and imaging modalities, is another promising form of theranostics for neurosurgery. Automated pathology analysis is yet another form of theranostics that can provide real-time feedback during the surgical resection of tumors. Electrical stimulation has also shown promise in optimizing therapies for patients with cerebral palsy. Overall, theranostics is a cost-effective way to optimize medical care for patients in neurosurgery. It is a relatively new field, but the advancements made so far show great promise for improving patient outcomes.
- Tan L, Jiang T, Yu JT, et al., 2016, Toward precision medicine in neurological diseases. Ann Transl Med, 4: 104. https://doi.org/10.21037/atm.2016.03.26
- Kelkar SS, Reineke TM, 2011, Theranostics: Combining imaging and therapy. Bioconjug Chem, 22: 1879–1903. https://doi.org/10.1021/bc200151q
- Marin JF, Nunes RF, Coutinho AM, et al., 2020, Theranostics in nuclear medicine: Emerging and re-emerging integrated imaging and therapies in the era of precision oncology. Radiographics, 40: 1715–1740. https://doi.org/10.1148/rg.2020200021
- Meng Y, Pople CB, Lea-Banks H, et al., 2021, Focused ultrasound neuromodulation. Int Rev Neurobiol, 159: 221–240. https://doi.org/10.1016/bs.irn.2021.06.004
- Barca C, Griessinger CM, Faust A, et al., 2021, Expanding theranostic radiopharmaceuticals for tumor diagnosis and therapy. Pharmaceuticals (Basel), 15: 13. https://doi.org/10.3390/ph15010013
- Lacruz CR, Catalina-Fernández I, Bardales RH, et al., 2015, Intraoperative consultation on pediatric central nervous system tumors by squash cytology. Cancer Cytopathol, 123: 331–346. https://doi.org/10.1002/cncy.21537
- Shideler BL, Bulea TC, Chen J, et al., 2020, Toward a hybrid exoskeleton for crouch gait in children with cerebral palsy: Neuromuscular electrical stimulation for improved knee extension. J Neuroeng Rehabil, 17: 121. https://doi.org/10.1186/s12984-020-00738-7
- European Society of Radiology (ESR), 2015, Medical imaging in personalised medicine: A white paper of the research committee of the European Society of Radiology (ESR). Insights Imaging, 6: 141–155. https://doi.org/10.1007/s13244-015-0394-0
- Overview. Focused Ultrasound Foundation, 2022. Available from: https://www.fusfoundation.org/the-technology/ overview [Last accessed on 2032 Mar 23].
- Etame AB, Diaz RJ, Smith CA, et al., 2012, Focused ultrasound disruption of the blood-brain barrier: A new frontier for therapeutic delivery in molecular neurooncology. Neurosurg Focus, 32: E3. https://doi.org/10.3171/2011.10.focus11252
- Sprowls SA, Arsiwala TA, Bumgarner JR, et al., 2019, Improving CNS delivery to brain metastases by blood-tumor barrier disruption. Trends Cancer, 5: 495–505. https://doi.org/10.1016/j.trecan.2019.06.003
- Lucas VS, Burk RS, Creehan S, et al., 2014, Utility of high-frequency ultrasound: Moving beyond the surface to detect changes in skin integrity. Plast Surg Nurs, 34: 34–38. https://doi.org/10.1097/psn.0000000000000031
- Beer R, O’Gorman C, Horwood K, et al., 2021, A case of IVIg responsive paraneoplastic SOX1 peripheral neuropathy in a male with breast carcinoma. J Neuroimmunol, 352: 577492. https://doi.org/10.1016/j.jneuroim.2021.577492
- Hynynen K, McDannold N, Vykhodtseva N, et al., 2006, Focal disruption of the blood-brain barrier due to 260-kHz ultrasound bursts: A method for molecular imaging and targeted drug delivery. J Neurosurg, 105: 445–454. https://doi.org/10.3171/jns.2006.105.3.445
- Dasgupta A, Liu M, Ojha T, et al., 2016, Ultrasound-mediated drug delivery to the brain: Principles, progress and prospects. Drug Discov Today Technol, 20: 41–48. https://doi.org/10.1016/j.ddtec.2016.07.007
- Jin Q, Kang ST, Chang YC, et al., 2016, Inertial cavitation initiated by polytetrafluoroethylene nanoparticles under pulsed ultrasound stimulation. Ultrason Sonochem, 32: 1–7. https://doi.org/10.1016/j.ultsonch.2016.02.009
- Liu HL, Hua MY, Yang HW, et al., 2010, Magnetic resonance monitoring of focused ultrasound/magnetic nanoparticle targeting delivery of therapeutic agents to the brain. Proc Natl Acad Sci U S A, 107: 15205–15210. https://doi.org/10.1073/pnas.1003388107
- Cloughesy TF, Mochizuki AY, Orpilla JR, et al., 2019, Neoadjuvant anti-PD-1 immunotherapy promotes a survival benefit with intratumoral and systemic immune responses in recurrent glioblastoma. Nat Med, 25: 477–486. https://doi.org/10.1038/s41591-018-0337-7
- Choi HJ, Han M, Seo H, et al., 2022, The new insight into the inflammatory response following focused ultrasound-mediated blood-brain barrier disruption. Fluids Barriers CNS, 19: 103. https://doi.org/10.1186/s12987-022-00402-3
- Arulpragasam AR, van ‘t Wout-Frank M, Barredo J, et al., 2022, Low intensity focused ultrasound for non-invasive and reversible deep brain neuromodulation-a paradigm shift in psychiatric research. Front Psychiatry, 13: 825802. https://doi.org/10.3389/fpsyt.2022.825802
- Rabut C, Yoo S, Hurt RC, et al., 2020, Ultrasound technologies for imaging and modulating neural activity. Neuron, 108: 93–110. https://doi.org/10.1016/j.neuron.2020.09.003
- Kubanek J, 2018, Neuromodulation with transcranial focused ultrasound. Neurosurg Focus, 44: E14. https://doi.org/10.3171/2017.11.focus17621
- Guo H, Hamilton M 2nd, Offutt SJ, et al., 2018, Ultrasound produces extensive brain activation via a cochlear pathway. Neuron, 98: 1020–1030.e4. https://doi.org/10.1016/j.neuron.2018.04.036
- Zhou YF, 2011, High intensity focused ultrasound in clinical tumor ablation. World J Clin Oncol, 2: 8–27. https://doi.org/10.5306/wjco.v2.i1.8
- Bunevicius A, McDannold NJ, Golby AJ, 2020, Focused ultrasound strategies for brain tumor therapy. Oper Neurosurg (Hagerstown), 19: 9–18. https://doi.org/10.1093/ons/opz374
- Hersh AM, Bhimreddy M, Weber-Levine C, et al., 2022, Applications of focused ultrasound for the treatment of glioblastoma: A new frontier. Cancers (Basel), 14: 4920. https://doi.org/10.3390/cancers14194920
- Maesawa S, Nakatsubo D, Tsugawa T, et al., 2021, Magnetic resonance-guided focused ultrasound ablation: Techniques and neurological applications. No Shinkei Geka, 49: 847–856. https://doi.org/10.11477/mf.1436204463
- Patel NA, Nycz CJ, Carvalho PA, et al., 2020, An integrated robotic system for MRI-guided neuroablation: Preclinical evaluation. IEEE Trans Biomed Eng, 67: 2990–2999. https://doi.org/10.1109/tbme.2020.2974583
- Morris ZS, Wang AZ, Knox SJ, 2021, The radiobiology of radiopharmaceuticals. Semin Radiat Oncol, 31: 20–27. https://doi.org/10.1016/j.semradonc.2020.07.002
- Sgouros G, Bodei L, McDevitt MR, et al., 2020, Radiopharmaceutical therapy in cancer: Clinical advances and challenges. Nat Rev Drug Discov, 19: 589–608. https://doi.org/10.1038/s41573-020-0073-9
- Gupta A, Sood A, Fuhrer E, et al., 2022, Polysaccharide-based theranostic systems for combined imaging and cancer therapy: Recent advances and challenges. ACS Biomater Sci Eng, 8: 2281–2306. https://doi.org/10.1021/acsbiomaterials.1c01631
- Palmisciano P, Watanabe G, Conching A, et al., 2022, The role of [68Ga]Ga-DOTA-SSTR PET radiotracers in brain tumors: A systematic review of the literature and ongoing clinical trials. Cancers (Basel), 14: 2925. https://doi.org/10.3390/cancers14122925
- Novruzov F, Aliyev JA, Jaunmuktane Z, et al., 2015, The use of (68)Ga DOTATATE PET/CT for diagnostic assessment and monitoring of (177)Lu DOTATATE therapy in pituitary carcinoma. Clin Nucl Med, 40: 47–49. https://doi.org/10.1097/rlu.0000000000000589
- Milker-Zabel S, Zabel-du Bois A, Henze M, et al., 2006, Improved target volume definition for fractionated stereotactic radiotherapy in patients with intracranial meningiomas by correlation of CT, MRI, and [68Ga]- DOTATOC-PET. Int J Radiat Oncol Biol Phys, 65: 222–227. https://doi.org/10.1016/j.ijrobp.2005.12.006
- Gehler B, Paulsen F, Oksüz MO, et al., 2009, [68Ga]- DOTATOC-PET/CT for meningioma IMRT treatment planning. Radiat Oncol, 4: 56. https://doi.org/10.1186/1748-717X-4-56
- Kowalski ES, Khairnar R, Gryaznov AA, et al., 2021, 68Ga-DOTATATE PET-CT as a tool for radiation planning and evaluating treatment responses in the clinical management of meningiomas. Radiat Oncol, 16: 151. https://doi.org/10.1186/s13014-021-01875-6
- Marincek N, Radojewski P, Dumont RA, et al., 2015, Somatostatin receptor-targeted radiopeptide therapy with 90Y-DOTATOC and 177Lu-DOTATOC in progressive meningioma: Long-term results of a phase II clinical trial. J Nucl Med, 56: 171–176. https://doi.org/10.2967/jnumed.114.147256
- Gerster-Gilliéron K, Forrer F, Maecke H, et al., 2015, 90Y-DOTATOC as a therapeutic option for complex recurrent or progressive meningiomas. J Nucl Med, 56: 1748–1751. https://doi.org/10.2967/jnumed.115.155853
- Bartolomei M, Bodei L, De Cicco C, et al., 2009, Peptide receptor radionuclide therapy with (90)Y-DOTATOC in recurrent meningioma. Eur J Nucl Med Mol Imaging, 36: 1407–1416. https://doi.org/10.1007/s00259-009-1115-z
- Otte A, Herrmann R, Heppeler A, et al., 1999, Yttrium-90 DOTATOC: First clinical results. Eur J Nucl Med, 26: 1439–1447. https://doi.org/10.1007/s002590050476
- Seystahl K, Stoecklein V, Schüller U, et al., 2016, Somatostatin receptor-targeted radionuclide therapy for progressive meningioma: Benefit linked to 68Ga-DOTATATE/-TOC uptake. Neuro Oncol, 18: 1538–1547. https://doi.org/10.1093/neuonc/now060
- Nabulsi NB, Mercier J, Holden D, et al., 2016, Synthesis and preclinical evaluation of 11C-UCB-J as a PET tracer for imaging the synaptic vesicle glycoprotein 2A in the brain. J Nucl Med, 57: 777–784. https://doi.org/10.2967/jnumed.115.168179
- Aqsa A, Droubi S, Al-Moussawi H, et al., 2022, Gastric signet ring cell adenocarcinoma presenting as intermittent volvulus of small bowel: A case presentation and review of the literature. Cureus, 14: e23228. https://doi.org/10.7759/cureus.23228
- Laxton AW, Stone S, Lozano AM, 2014, The neurosurgical treatment of Alzheimer’s disease: A review. Stereotact Funct Neurosurg, 92: 269–281. https://doi.org/10.1159/000364914
- Bertoglio D, Verhaeghe J, Miranda A, et al., 2020, Validation and noninvasive kinetic modeling of [11C]UCB-J PET imaging in mice. J Cereb Blood Flow Metab, 40: 1351–1362. https://doi.org/10.1177/0271678X19864081
- Wilson H, Pagano G, de Natale ER, et al., 2020, Mitochondrial complex 1, sigma 1, and synaptic vesicle 2A in early drug-naive Parkinson’s disease. Mov Disord, 35: 1416–1427. https://doi.org/10.1002/mds.28064
- Chen MK, Mecca AP, Naganawa M, et al., 2021, Comparison of [11C]UCB-J and [18F]FDG PET in Alzheimer’s disease: A tracer kinetic modeling study. J Cereb Blood Flow Metab, 41: 2395–2409. https://doi.org/10.1177/0271678X211004312
- Acuff SN, Mathotaarachchi S, Zukotynski K, et al., 2020, Clinical and technical considerations for brain PET imaging for dementia. J Nucl Med Technol, 48: 5–8. https://doi.org/10.2967/jnmt.118.220087
- Rogers L, Barani I, Chamberlain M, et al., 2015, Meningiomas: Knowledge base, treatment outcomes, and uncertainties. A RANO review. J Neurosurg, 122: 4–23. https://doi.org/10.3171/2014.7.JNS131644
- Park JH, de Lomana AL, Marzese DM, et al., 2021, A systems approach to brain tumor treatment. Cancers (Basel), 13: 3152. https://doi.org/10.3390/cancers13133152
- Aziz SJ, Zeman-Pocrnich CE, 2022, Tissue processing. Methods Mol Biol, 2422: 47–63. https://doi.org/10.1007/978-1-0716-1948-3_4
- Slaoui M, Bauchet AL, Fiette L, 2017, Tissue sampling and processing for histopathology evaluation. Methods Mol Biol, 1641: 101–114. https://doi.org/10.1007/978-1-4939-7172-5_4
- Bui MM, Smith P, Agresta SV, et al., 2008, Practical issues of intraoperative frozen section diagnosis of bone and soft tissue lesions. Cancer Control, 15: 7–12. https://doi.org/10.1177/107327480801500102
- Howat WJ, Wilson BA, 2014, Tissue fixation and the effect of molecular fixatives on downstream staining procedures. Methods, 70: 12–19. https://doi.org/10.1016/j.ymeth.2014.01.022
- Grizzle WE, 2009, Special symposium: Fixation and tissue processing models. Biotech Histochem, 84: 185–193. https://doi.org/10.3109/10520290903039052
- Fischer AH, Jacobson KA, Rose J, et al., 2008, Hematoxylin and eosin staining of tissue and cell sections. CSH Protoc, 2008. https://doi.org/10.1101/pdb.prot4986
- Einstein EH, Ablyazova F, Rosenberg A, et al., 2022, Stimulated Raman histology facilitates accurate diagnosis in neurosurgical patients: A one-to-one noninferiority study. J Neurooncol, 159: 369–375. https://doi.org/10.1007/s11060-022-04071-y
- Blokker M, de Witt Hamer PC, Wesseling P, et al., 2022, Fast intraoperative histology-based diagnosis of gliomas with third harmonic generation microscopy and deep learning. Sci Rep, 12: 11334. https://doi.org/10.1038/s41598-022-15423-z
- Freudiger CW, Min W, Saar BG, et al., 2008, Label-free biomedical imaging with high sensitivity by stimulated Raman scattering microscopy. Science, 322: 1857–1861. https://doi.org/10.1126/science.1165758
- Lee M, Herrington CS, Ravindra M, et al., 2021, Recent advances in the use of stimulated Raman scattering in histopathology. Analyst, 146: 789–802. https://doi.org/10.1039/d0an01972k
- Hollon TC, Pandian B, Adapa AR, et al., 2020, Near real-time intraoperative brain tumor diagnosis using stimulated Raman histology and deep neural networks. Nat Med, 26: 52–58. https://doi.org/10.1038/s41591-019-0715-9
- Tofte K, Berger C, Torp SH, et al., 2014, The diagnostic properties of frozen sections in suspected intracranial tumors: A study of 578 consecutive cases. Surg Neurol Int, 5: 170. https://doi.org/10.4103/2152-7806.146153
- Martirosyan NL, Georges J, Eschbacher JM, et al., 2014, Potential application of a handheld confocal endomicroscope imaging system using a variety of fluorophores in experimental gliomas and normal brain. Neurosurg Focus, 36: E16. https://doi.org/10.3171/2013.11.focus13486
- Abramov I, Park MT, Gooldy TC, et al., 2022, Real-time intraoperative surgical telepathology using confocal laser endomicroscopy. Neurosurgical Focus, 52: E9. https://doi.org/10.3171/2022.3.focus2250
- Chien CH, 2020, Thinking Beyond Fluorescence: Biological Imaging with SHG and THG Microscopy. Discovery Blog. Japan: Olympus Life Science. Available from: https://www. olympus-lifescience.com/en/discovery/thinking-beyond-fluorescence-biological-imaging-with-shg-and-thg-microscopy [Last accessed on 2023 Mar 24].
- Weigelin B, Bakker GJ, Friedl P, 2016, Third harmonic generation microscopy of cells and tissue organization. J Cell Sci, 129: 245–255. https://doi.org/10.1242/jcs.152272
- Gavgiotaki E, Filippidis G, Tsafas V, et al., 2020, Third Harmonic Generation microscopy distinguishes malignant cell grade in human breast tissue biopsies. Sci Rep, 10: 11055. https://doi.org/10.1038/s41598-020-67857-y
- Izadyyazdanabadi M, Belykh E, Mooney MA, et al., 2018, Prospects for theranostics in neurosurgical imaging: Empowering confocal laser endomicroscopy diagnostics via deep learning. Front Oncol, 8: 240. https://doi.org/10.3389/fonc.2018.00240
- Epe B, Pflaum M, Boiteux S, 1993, DNA damage induced by photosensitizers in cellular and cell-free systems. Mutat Res, 299: 135–145. https://doi.org/10.1016/0165-1218(93)90091-q
- Ge J, Wood DK, Weingeist DM, et al., 2013, Standard fluorescent imaging of live cells is highly genotoxic. Cytometry A, 83: 552–560. https://doi.org/10.1002/cyto.a.22291
- Martirosyan NL, Eschbacher JM, Kalani MY, et al., 2016, Prospective evaluation of the utility of intraoperative confocal laser endomicroscopy in patients with brain neoplasms using fluorescein sodium: Experience with 74 cases. Neurosurgical Focus, 40: E11. https://doi.org/10.3171/2016.1.focus15559
- Vitrikas K, Dalton H, Breish D, 2020, Cerebral palsy: An overview. Am Fam Physician, 101: 213–220.
- Damiano DL, Prosser LA, Curatalo LA, et al., 2013, Muscle plasticity and ankle control after repetitive use of a functional electrical stimulation device for foot drop in cerebral palsy. Neurorehabil Neural Repair, 27: 200–207. https://doi.org/10.1177/1545968312461716
- Pool D, Valentine J, Bear N, et al., 2015, The orthotic and therapeutic effects following daily community applied functional electrical stimulation in children with unilateral spastic cerebral palsy: A randomised controlled trial. BMC Pediatr, 15: 154. https://doi.org/10.1186/s12887-015-0472-y
- Fang Y, Lerner ZF, 2021, Feasibility of augmenting ankle exoskeleton walking performance with step length biofeedback in individuals with cerebral palsy. IEEE Trans Neural Syst Rehabil Eng, 29: 442–449. https://doi.org/10.1109/TNSRE.2021.3055796
- Willerslev-Olsen M, Andersen JB, Sinkjaer T, et al., 2014, Sensory feedback to ankle plantar flexors is not exaggerated during gait in spastic hemiplegic children with cerebral palsy. J Neurophysiol, 111: 746–754. https://doi.org/10.1152/jn.00372.2013
- Moll I, Marcellis RG, Coenen ML, et al., 2022, A randomized crossover study of functional electrical stimulation during walking in spastic cerebral palsy: The FES on participation (FESPa) trial. BMC Pediatr, 22: 37. https://doi.org/10.1186/s12887-021-03037-9
- Frisk RF, Jensen P, Kirk H, et al., 2017, Contribution of sensory feedback to plantar flexor muscle activation during push-off in adults with cerebral palsy. J Neurophysiol, 118: 3165–3174. https://doi.org/10.1152/jn.00508.2017
- MacIntosh A, Desailly E, Vignais N, et al., 2020, A biofeedback-enhanced therapeutic exercise video game intervention for young people with cerebral palsy: A randomized single-case experimental design feasibility study. PLoS One, 15: e0234767. https://doi.org/10.1371/journal.pone.0234767
- Wood KC, Lathan CE, Kaufman KR, 2013, Feasibility of gestural feedback treatment for upper extremity movement in children with cerebral palsy. IEEE Trans Neural Syst Rehabil Eng, 21: 300–305. https://doi.org/10.1109/tnsre.2012.2227804
- Susko T, Swaminathan K, Krebs HI, 2016, MIT-skywalker: A novel gait neurorehabilitation robot for stroke and cerebral palsy. IEEE Trans Neural Syst Rehabil Eng, 24: 1089–1099. https://doi.org/10.1109/tnsre.2016.2533492
- Sukal-Moulton T, Clancy T, Zhang LQ, et al., 2014, Clinical application of a robotic ankle training program for cerebral palsy compared to the research laboratory application: Does it translate to practice? Arch Phys Med Rehabil, 95: 1433–1440. https://doi.org/10.1016/j.apmr.2014.04.010
- Moreau NG, Bodkin AW, Bjornson K, et al., 2016, Effectiveness of rehabilitation interventions to improve gait speed in children with cerebral palsy: Systematic review and meta-analysis. Phys Ther, 96: 1938–1954. https://doi.org/10.2522/ptj.20150401
- Gillick B, Rich T, Nemanich S, et al., 2018, Transcranial direct current stimulation and constraint-induced therapy in cerebral palsy: A randomized, blinded, sham-controlled clinical trial. Eur J Paediatr Neurol, 22: 358–368. https://doi.org/10.1016/j.ejpn.2018.02.001
- Ertzgaard P, Alwin J, Sörbo A, et al., 2018, Evaluation of a self-administered transcutaneous electrical stimulation concept for the treatment of spasticity: A randomized placebo-controlled trial. Eur J Phys Rehabil Med, 54: 507–517. https://doi.org/10.23736/S1973-9087.17.04791-8
- Saleem GT, Crasta JE, Slomine BS, et al., 2019, Transcranial direct current stimulation in pediatric motor disorders: A systematic review and meta-analysis. Arch Phys Med Rehabil, 100: 724–738. https://doi.org/10.1016/j.apmr.2018.10.011
- Grecco LA, Duarte ND, de Mendonça ME, et al., 2013, Effect of transcranial direct current stimulation combined with gait and mobility training on functionality in children with cerebral palsy: Study protocol for a double-blind randomized controlled clinical trial. BMC Pediatr, 13: 168. https://doi.org/10.1186/1471-2431-13-168
- Chen XL, Yu LP, Zhu Y, et al., 2021, Combined effect of hydrotherapy and transcranial direct-current stimulation on children with cerebral palsy: A protocol for a randomized controlled trial. Medicine (Baltimore), 100: e27962. https://doi.org/10.1097/md.0000000000027962
- Inguaggiato E, Bolognini N, Fiori S, et al., 2019, Transcranial direct current stimulation (tDCS) in unilateral cerebral palsy: A pilot study of motor effect. Neural Plast, 2019: 2184398. https://doi.org/10.1155/2019/2184398
- Rich TL, Nemanich S, Chen M, et al., 2018, Transcranial direct current stimulation (tDCS) paired with occupation-centered bimanual training in children with unilateral cerebral palsy: A preliminary study. Neural Plast, 2018: 9610812. https://doi.org/10.1155/2018/9610812
- Moura RC, Santos CA, Grecco LA, et al., 2016, Transcranial direct current stimulation combined with upper limb functional training in children with spastic, hemiparetic cerebral palsy: Study protocol for a randomized controlled trial. Trials, 17: 405. https://doi.org/10.1186/s13063-016-1534-7
- Perides S, Lin JP, Lee G, et al., 2020, Deep brain stimulation reduces pain in children with dystonia, including in dyskinetic cerebral palsy. Dev Med Child Neurol, 62: 917–925. https://doi.org/10.1111/dmcn.14555
- Lin S, Zhang C, Li H, et al., 2020, High frequency deep brain stimulation of superior cerebellar peduncles in a patient with cerebral palsy. Tremor Other Hyperkinet Mov (N Y), 10: 38. https://doi.org/10.5334/tohm.551
- Diniz JM, Cury RG, Iglesio RF, et al., 2021, Dentate nucleus deep brain stimulation: Technical note of a novel methodology assisted by tractography. Surg Neurol Int, 12: 400. https://doi.org/10.25259/sni_338_2021
- Akakin A, Peris-Celda M, Kilic T, et al., 2014, The dentate nucleus and its projection system in the human cerebellum: The dentate nucleus microsurgical anatomical study. Neurosurgery, 74: 401–424; discussion 424–425. https://doi.org/10.1227/NEU.0000000000000293
- Choi S, Shin YB, Kim SY, et al., 2018, A novel sensor-based assessment of lower limb spasticity in children with cerebral palsy. J Neuroeng Rehabil, 15: 45. https://doi.org/10.1186/s12984-018-0388-5
- Stanslaski SR, Case MA, Giftakis JE, et al., 2022, Long term performance of a bi-directional neural interface for deep brain stimulation and recording. Front Hum Neurosci, 16: 916627. https://doi.org/10.3389/fnhum.2022.916627
- Starr PA, 2018, Totally implantable bidirectional neural prostheses: A flexible platform for innovation in neuromodulation. Front Neurosci, 12: 619. https://doi.org/10.3389/fnins.2018.00619
- Lopes EM, Rego R, Rito M, et al., 2022, Estimation of ANT-DBS electrodes on target positioning based on a new percept™ PC LFP signal analysis. Sensors (Basel), 22: 6601. https://doi.org/10.3390/s22176601