Are Plant-derived Flavonoids the Emerging Anti-coronavirus Agents?
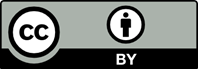
The current outbreak of coronavirus disease 2019 (COVID-19), which is an infectious disease caused by severe acute respiratory syndrome coronavirus 2 (SARS-CoV-2), has negatively impacted the global health and economy. Severe acute respiratory syndrome coronavirus 2 (SARS-CoV-2) shares many similarities with SARS-CoV and Middle East respiratory syndrome-related coronavirus. Within the past 20 years, these three highly pathogenic and deadly viruses have caused serious global infections and mortalities. It has been identified that the 3C-like protease (3CLpro) enzyme in coronaviruses can be a major therapeutic target for combating these serious infections. Therefore, flavonoids are believed to hold high potential in eliminating the viruses and infections. Flavonoids are polyphenolic secondary metabolites found in plants and have been demonstrated for their notable benefits for health. The antiviral activity of flavonoids has been reported in recent studies. Flavonoids, such as apigenin, quercetin, luteolin, amentoflavone, epigallocatechin gallate, gallocatechin gallate, and kaempferol, are known to be able to fight against coronaviruses by reducing the 3CLpro activity, according to the docking studies. Besides, we also found that several flavonoids have the potential to suppress the inflammatory cytokines, which are generally expressed in the lungs of coronavirus-infected individuals. However, the studies utilizing 3CLpro using various scaffolds of flavonoids need to be performed for better understanding on the antiviral potential of flavonoid derivatives against 3CLpro.
Zhang, L.; Liu, Y. Potential Interventions for Novel Coronavirus in China: A Systematic Review. J. Med. Virol., 2020, 92, 479–90.
Drosten, C.; Günther, S., Preiser, W.; van der Werf, S.; Brodt, H.R.; Becker, S.; Rabenau, H.; Panning, M.; Kolesnikova, L.; Fouchier, R.A.M.; Berger, A.; Burguière, A.M.; Cinatl, J.; Eickmann, M.; Escriou, N.; Grywna, K.; Kramme, S.; Manuguerra, J.C.; Müller, S.; Rickerts, V.; Stürmer, M.; Vieth, S.; Klenk, H.D.; Osterhaus, A.D.M.; Schmitz, H.; Doerr, H.W. Identification of a Novel Coronavirus in Patients with Severe Acute Respiratory Syndrome. N. Engl. J. Med., 2003, 348, 1967–76.
Donnelly, C.A.; Ghani, A.C.; Leung, G.M.; Hedley, A.J.; Fraser, C.; Riley, S.; Abu-Raddad, L.J.; Ho, L.M.; Thach, T.Q.; Chau, P.; Chan, K.P.; Lam, T.H.; Tse, L.Y.; Tsang, T.; Liu, S.H.; Kong, J.H.B.; Lau, E.M.C.; Ferguson, N.M.; Anderson, R.M. Epidemiological Determinants of Spread of Causal Agent of Severe Acute Respiratory Syndrome in Hong Kong. Lancet, 2003, 361, 1761–6.
Ren, Z.; Yan, L.; Zhang, N.; Guo, Y.; Yang, C.; Lou, Z.; Rao, Z. The Newly Emerged SARS-like Coronavirus HCoV-EMC also has an “Achilles’ Heel”: Current Effective Inhibitor Targeting a 3C-Like Protease. Protein. Cell, 2013, 4, 248–50.
Kilianski, A.; Mielech, A.M.; Deng, X.; Baker, S.C. Assessing Activity and Inhibition of Middle East Respiratory Syndrome Coronavirus Papain-like and 3C-Like Proteases Using Luciferase[1]Based Biosensors. J. Virol., 2013, 87, 11955–62.
Nielsen, S.F.; Boesen, T.; Larsen, M.; Schønning, K.; Kromann, H. Antibacterial Chalcones Bioisosteric Replacement of the 4’-hydroxy Group. Bioorg. Med. Chem., 2004, 12(11), 3047–54.
Spencer, J.P., Mohsen, M.M.A.; Minihane, A.M.; Mathers, J.C. Biomarkers of the intake of Dietary Polyphenols: Strengths, Limitations and Application in Nutrition Research. Br. J. Nutr., 2008, 99(1), 12–22.
Wu, F.; Zhao, S.; Yu, B.; Chen, Y.M.; Wang, W.; Song, Z.G.; Hu, Y.; Tao, Z.W.; Tian, J.H.; Pei, Y.Y.; Yuan, M.L.; Zhang, Y.L.; Dai, F.H.; Liu, Y.; Wang, Q.M.; Zheng, J.J.; Xu, L.; Holmes, E.C.; Zhang, Y.Z. A New Coronavirus Associated with Human Respiratory Disease in China. Nature, 2020, 579, 265–82.
Jo, S.; Kim, S.; Shin, D.H.; Kim, M.S. Inhibition of SARS-CoV 3CL Protease by Flavonoids. J. Enzyme. Inhib. Med. Chem., 2020, 35(1), 145–51.
Shen, W.; Lu, Y.H. Molecular Docking of Citrus Flavonoids with some Targets Related to Diabetes. Bangladesh. J. Pharmacol., 2013, 8(2), 156–70.
Madeswaran, A.; Umamaheswari, M; Asokkumar, K.; Sivashanmugam, T.; Subhadradevi, V.; Jagannath, P. Docking Studies: In Silico Phosphodiesterase Inhibitory Activity of Commercially Available Flavonoids. Bangladesh. J. Pharmacol. 2012, 7(1), 70–5.
Nguyen, T.T.; Woo, H.J.; Kang, H.K.; Nguyen, V.D.; Kim, Y.M.; Kim, D.W.; Ahn, S.A.; Xia, Y.; Kim, D. Flavonoid-mediated Inhibition of SARS Coronavirus 3C-like Protease Expressed in Pichia pastoris. Biotechnol. Lett., 2012, 34(5), 831–8.
Ryu, Y.B.; Jeong, H.J.; Kim, J.H.; Kim, Y.M.; Park, J.Y.; Kim, D.; Nguyen, T.T.H.; Park, S.J.; Chang, J.S.; Park, K.H.; Rho, M.C.; Lee, W.S. Biflavonoids from Torreya nucifera Displaying SARS[1]CoV 3CL(pro) Inhibition. Bioorg. Med. Chem. 2010, 18(22), 7940–7.
Lin, C.W.; Tsai, F.J.; Tsai, C.H; Lai, C.C.; Wan, L.; Ho, T.Y.; Hsieh, C.C.; Chao, P.D.L. Anti-SARS Coronavirus 3C-like Protease Effects of Isatis indigotica Root and Plant-derived Phenolic Compounds. Antiviral. Res. 2005, 68, 36–42.
Cheng, L.; Zheng, W.; Li. M.; Huang, J.; Bao, S.; Xu, Q.; Ma, Z. Citrus Fruits Are Rich in Flavonoids for Immunoregulation and Potential Targeting ACE2. Preprints, 2020, 2020, 020313.
Schwarz, S.; Sauter, D.; Wang, K. Kaempferol Derivatives as Antiviral Drugs against the 3a Channel Protein of Coronavirus. Planta. Med., 2014, 80(2-3), 177–82.
Ngwa, W.; Kumar, R.; Thompson, D.; Lyerly, W.; Moore, R.; Reid, T.E.; Loew, H.; Toyang, N. Potential of Flavonoid-inspired Phytomedicines against COVID-19. Molecules. 2020, 25, 2707.
Pandey, P.; Khan, F.; Rana, A.K.; Srivastava, Y.; Jha, S.K.; Jha, N.K. A Drug Repurposing Approach Towards Elucidating the Potential of Flavonoids as COVID-19 Spike Protein Inhibitors. Biointerface. Res. Appl. Chem. 2021, 11, 8482–501.
Hoffmann, M.; Kleine-Weber, H.; Schroeder, S.; Krüger, N.; Herrler, T.; Erichsen, S.; Schiergens, T.S.; Herrler, G.; Wu, N.H.; Nitsche, A.; Müller, M.A.; Drosten, C.; Pöhlmann, S. SARS-CoV-2 Cell Entry Depends on ACE2 and TMPRSS2 and is Blocked by a Clinically Proven Protease Inhibitor. Cell. 2020, 181, 271-80.
Chikhale, R.V.; Gupta, V.K.; Eldesoky, G.E.; Wabaidur, S.M.; Patil, S.A.; Islam, M.A. Identification of Potential anti-TMPRSS2 Natural Products through Homology Modelling, Virtual Screening and Molecular Dynamics Simulation Studies. J. Biomol. Struct. Dyn. 2020,
Jha, N.K.; Jeyaraman, M.; Rachamalla, M.; Ojha, S.; Dua, K.; Chellappan, D.K.; Muthu, S.; Sharma, A.; Jha, S.K.; Jain, R.; Jeyaraman, N.; Gs, P.; Satyam, R.; Khan, F.; Pandey, Verama, N.; Sing, S.K.; Roychoudhury, S.; Dholpuria, S.; Ruokolainen, J.; Kesari, K.K. Current understanding of novel coronavirus: Molecular pathogenesis, diagnosis, and treatment approaches. Immuno, 2021, 1, 30–66.
Colunga, B.R.M.; Berrill, M.; Catravas, J.D.; Marik, P.E. Quercetin and Vitamin C: An Experimental, Synergistic Therapy for the Prevention and Treatment of SARS-CoV-2 Related Disease (COVID-19). Front. Immunol., 2020, 11, 1451.
Báez-Santos, Y.M.; John, S.E.S.; Mesecar, A.D. The SARS[1]coronavirus Papain-like Protease: Structure, Function and Inhibition by Designed Antiviral Compounds. Antiviral. Res. 2015, 115, 21–38.
Yoshida, H.; Takamura, N.; Shuto, T.; Ogata, K.; Tokunaga, J.; Kawai, K.; Kai, H. The Citrus Flavonoids Hesperetin and Naringenin Block the Lipolytic Actions of TNF-alpha in Mouse Adipocytes. Biochem. Biophys. Res. Commun., 2010, 394, 728–32.
Ma, H.; Feng, X.; Ding, S. Hesperetin Attenuates Ventilator[1]induced Acute Lung Injury through Inhibition of NF-κB-mediated Inflammation. Eur. J. Pharmacol., 2015, 769, 333–41.
Ye, J.; Guan, M.; Lu, Y.; Zhang, D.; Li, C.; Li, Y.; Zhou, C. Protective Effects of Hesperetin on Lipopolysaccharide-induced Acute Lung Injury by Targeting MD2. Eur. J. Pharmacol., 2019, 852, 151–8.
Peng, H.L.; Huang, W.C.; Cheng, S.C.; Liou, C.J. Fisetin Inhibits the Generation of Inflammatory Mediators in Interleukin-1beta[1]induced Human Lung Epithelial Cells by Suppressing the NF-κB and ERK1/2 Pathways. Int. Immunopharmacol., 2018, 60, 202–10.
Tutunchi, H.; Naeini, F.; Ostadrahimi, A.; Hosseinzadeh-Attar, M.J. Naringenin, a Flavanone with Antiviral and Anti-inflammatory Effects: A Promising Treatment Strategy against COVID-19. Phytother. Res. 2020, 34, 3137–47.
Ali, R.; Shahid, A.; Ali, N.; Hasan, S.K.; Majed, F.; Sultana, S. Amelioration of Benzo[a]pyrene-induced Oxidative Stress and Pulmonary Toxicity by Naringenin in Wistar Rats: A Plausible Role of COX-2 and NF-κB. Hum. Exp. Toxicol., 2017, 36, 349–64.
Fouad, A.A.; Albuali, W.H.; Jresat, I. Protective Effect of Naringenin against Lipopolysaccharide-induced Acute Lung Injury in Rats. Pharmacology, 2016, 97, 224–32.
Coz, Y.A.O.; Simpson, S.Q. Coronavirus Disease 2019 Sepsis: A Nudge toward Antibiotic Stewardship. Chest, 2020, 158, 1833–4.