Modulation of Mitochondrial Function in Elderly Brain: Involvement of Autophagy and Apoptosis
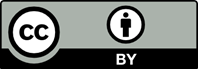
Mitochondria are crucial cellular organelles that regulate energy production, oxidative balance, and calcium homeostasis, among other things. Aging is a natural, multifactorial, and multi-organic process, in which both pathological and physiological changes occur gradually over time. Autophagy capacity and mitochondrial processes such as mitophagy, biogenesis, and dynamics are associated with aging. These processes are important for maintaining the structural integrity of mitochondria and thus cell life since mitochondrial dysfunction leads to an impairment in energy metabolism and an increased production of reactive oxygen species, both of which may stimulate mechanisms of cellular senescence and apoptotic cell death. Furthermore, in both model organisms and humans, a decline in mitochondrial activity can contribute to age-related disease phenotypes. As people age, mitochondrial damage and malfunction may induce apoptosis, thus explaining the rise in cell death. Many aging processes are believed to be driven by oxidative stress. Taken together, the evidence strongly suggests a relation between mitochondrial function and a wide spectrum of aging processes. Although there is no general consensus among the studies reviewed, it appears that aging reduces mitochondrial biogenesis and dynamics, as well as the mitophagy capacity of the organism involved. This review describes the involvement of autophagy and apoptosis in the modulation of mitochondrial function in the brain.
Anderson, N.D.; Craik, F.I.M. 50 Years of Cognitive Aging Theory. J. Gerontol. B Psychol. Sci. Soc. Sci., 2017, 72(1), 1–6.
Lindenberger, U.; Nagel, I.E.; Chicherio, C.; Li, S.C.; Heekeren, H.R.; Bäckman, L. Age-Related Decline in Brain Resources Modulates Genetic Effects on Cognitive Functioning. Front. Neurosci., 2008, 2(2), 234–44.
López-Otín, C.; Blasco, M.A.; Partridge, L.; Serrano, M.; Kroemer, G. The Hallmarks of Aging. Cell, 2013, 153(6), 1194–217.
Harada, C.N.; Love, M.C.N.; Triebel, K.L. Normal Cognitive Aging. Clin. Geriatr. Med., 2013, 29(4), 737–52.
Bratic, A.; Larsson, N.G. The Role of Mitochondria in Aging. J. Clin. Invest., 2013, 123(3), 951–57.
Mejías-Peña, Y.; Estébanez, B.; Rodriguez-Miguelez, P.; Fernandez-Gonzalo, R.; Almar, M.; de Paz, J.A.; González- Gallego, J.; Cuevas, M.J. Impact of Resistance Training on the Autophagy-Inflammation-Apoptosis Crosstalk in Elderly Subjects. Aging (Albany NY), 2017, 9(2), 408–18.
Schiavi, A.; Ventura, N. The Interplay between Mitochondria and Autophagy and its Role in the Aging Process. Exp. Gerontol., 2014, 56, 147–53.
Palikaras, K.; Tavernarakis, N. Mitophagy in Neurodegeneration and Aging. Front. Genet., 2012, 3, 297.
Payne, B.A.I.; Chinnery, P.F. Mitochondrial Dysfunction in Aging: Much Progress but Many Unresolved Questions. Biochim. Biophys. Acta, 2015, 1847(11), 1347–53.
Parihar, M.S.; Brewer, G.J. Simultaneous Age-Related Depolarization of Mitochondrial Membrane Potential and Increased Mitochondrial Reactive Oxygen Species Production Correlate with Age-Related Glutamate Excitotoxicity in Rat Hippocampal Neurons. J. Neurosci. Res., 2007, 85(5), 1018–32.
Elmore, S.P.; Qian, T.; Grissom, S.F.; Lemasters, J.J. The Mitochondrial Permeability Transition Initiates Autophagy in Rat Hepatocytes. FASEB J., 2001, 15(12), 2286–7.
Palikaras, K.; Lionaki, E.; Tavernarakis, N. Coupling Mitogenesis and Mitophagy for Longevity. Autophagy, 2015, 11(8), 1428–30.
Kerr, J.F.; Wyllie, A.H.; Currie, A.R. Apoptosis: A Basic Biological Phenomenon with Wide-Ranging Implications in Tissue Kinetics. Br. J. Cancer, 1972, 26(4), 239–57.
Pollack, M.; Phaneuf, S.; Dirks, A.; Leeuwenburgh, C. The Role of Apoptosis in the Normal Aging Brain, Skeletal Muscle, and Heart. Ann. N. Y. Acad. Sci., 2002, 959, 93–107.
Harman, D. Aging: A Theory Based on Free Radical and Radiation Chemistry. J. Gerontol., 1956, 11(3), 298–300.
Harman, D. The Biologic Clock: The Mitochondria? J. Am. Geriatr. Soc., 1972, 20(4), 145–7.
Miquel, J.; Economos, A.C.; Fleming, J.; Johnson, J.E. Jr. Mitochondrial Role in Cell Aging. Exp. Gerontol., 1980, 15(6), 575–91.
Pak, J.W.; Herbst, A.; Bua, E.; Gokey, N.; McKenzie, D.; Aiken, J.M. Mitochondrial DNA Mutations as a Fundamental Mechanism in Physiological Declines Associated with Aging. Aging Cell, 2003, 2(1), 1–7.
Wei, Y.H. Mitochondrial DNA Alterations as Ageing-Associated Molecular Events. Mutat. Res., 1992, 275(3-6), 145–55.
Larsson, N.G. Somatic Mitochondrial DNA Mutations in Mammalian Aging. Annu. Rev. Biochem., 2010, 79, 683–706.
Marde, V.S.; Atkare, U.A.; Gawali, S.V.; Tiwari, P.L.; Badole, S.P.; Wankhede, N.L.; Taksande, B.G.; Upaganlawar, A.B.; Umekar, M.J.; Kale, M.B. Alzheimer’s Disease and Sleep Disorders: Insights into the Possible Disease Connections and the Potential Therapeutic Targets. Asian J. Psychiatr., 2022, 68, 102961.
Linnane, A.W.; Ozawa, T.; Marzuki, S.; Tanaka, M. Mitochondrial DNA Mutations as an Important Contributor to Ageing and Degenerative Diseases. Lancet, 1989, 1(8639), 642–5.
Judge, S.; Leeuwenburgh, C. Cardiac Mitochondrial Bioenergetics, Oxidative Stress, and Aging. Am. J. Physiol. Cell Physiol., 2007, 292(6), C1983–92.
Kale, M.; Nimje, N.; Aglawe, M.M.; Umekar, M.; Taksande, B.; Kotagale, N. Agmatine Modulates Anxiety and Depression-Like Behaviour in Diabetic Insulin-Resistant Rats. Brain Res., 2020, 1747, 147045.
Sanz, A. Mitochondrial Reactive Oxygen Species: Do They Extend or Shorten Animal Lifespan? Biochim. Biophys. Acta, 2016, 1857(8), 1116–26.
Novak, I. Mitophagy: A Complex Mechanism of Mitochondrial Removal. Antioxid. Redox Signal., 2012, 17(5), 794–802.
Carter, H.N.; Chen, C.C.W.; Hood, D.A. Mitochondria, Muscle Health, and Exercise with Advancing Age. Physiology (Bethesda), 2015, 30(3), 208–23.
Palikaras, K.; Tavernarakis, N. Mitochondrial Homeostasis: The Interplay between Mitophagy and Mitochondrial Biogenesis. Exp. Gerontol., 2014, 56, 182–8.
Mishra, P.; Chan, D.C. Metabolic Regulation of Mitochondrial Dynamics. J. Cell Biol., 2016, 212(4), 379–87.
Kauppila, T.E.S.; Kauppila, J.H.K.; Larsson, N.G. Mammalian Mitochondria and Aging: An Update. Cell Metab., 2017, 25(1), 57–71.
Tiwari, P.; Wankhede, N.; Badole, S.; Umare, M.; Taksande, B.; Upaganlawar, A; Umekar, M.; Kale, M. Mitochondrial Dysfunction in Ageing: Involvement of Oxidative Stress and Role of Melatonin. Bull. Env. Pharmacol. Life Sci., 2021, 10(2), 156–72.
Schrepfer, E.; Scorrano, L. Mitofusins, from Mitochondria to Metabolism. Mol. Cell, 2016, 61(5), 683–94.
Hekimi, S.; Wang, Y.; Noë, A. Mitochondrial ROS and the Effectors of the Intrinsic Apoptotic Pathway in Aging Cells: The Discerning Killers! Front. Genet., 2016, 7, 161.
Poulose, N.; Raju, R. Sirtuin Regulation in Aging and Injury. Biochim. Biophys. Acta, 2015, 1852(11), 2442–55.
Hamacher-Brady, A.; Brady, N.R. Mitophagy Programs: Mechanisms and Physiological Implications of Mitochondrial Targeting by Autophagy. Cell. Mol. Life Sci., 2016, 73(4), 775–95.
Springer, M.Z.; Macleod, K.F. In Brief: Mitophagy: Mechanisms and Role in Human Disease. J. Pathol., 2016, 240(3), 253–5.
Gouspillou, G.; Sgarioto, N.; Norris, B.; Barbat-Artigas, S.; Aubertin-Leheudre, M.; Morais, J.A.; Burelle, Y.; Taivassalo, T.; Hepple, R.T. The Relationship between Muscle Fiber Type- Specific PGC-1α Content and Mitochondrial Content Varies between Rodent Models and Humans. PLoS One, 2014, 9(8), e103044
Ding, W.X.; Yin, X.M. Mitophagy: Mechanisms, Pathophysiological Roles, and Analysis. Biol. Chem., 2012, 393(7), 547–64.
Eiyama, A.; Okamoto, K. PINK1/Parkin-Mediated Mitophagy in Mammalian Cells. Curr. Opin. Cell Biol., 2015, 33, 95–101.
Taksande, B.G.; Sharma, O.; Aglawe, M.M.; Kale, M.B.; Gawande, D.Y.; Umekar, M.J.; Kotagale, N.R. Acute Orexigenic Effect of Agmatine Involves Interaction between Central α2- Adrenergic and GABAergic Receptors. Biomed. Pharmacother., 2017, 93, 939–47.
Durcan, T.M.; Tang, M.Y.; Pérusse, J.R.; Dashti, E.A.; Aguileta, M.A.; McLelland, G.L.; Gros, P.; Shaler, T.A.; Faubert, D.; Coulombe, B.; Fon, E.A. USP8 Regulates Mitophagy by Removing K6-Linked Ubiquitin Conjugates from Parkin. EMBO J., 2014, 33(21), 2473–21.
Kale, M.B.; Rai, K.R.; Wankhede, N.L.; Awandekar, N.B.; Umekar, M.J. The Use of Convalescent Plasma as a Novel Treatment in Pandemic Disease: A Review. J. Glob. Trends Pharm. Sci., 2020, 11(2), 7953–9.
Gomes, L.C.; Scorrano, L. Mitochondrial Morphology in Mitophagy and Macroautophagy. Biochim. Biophys. Acta, 2013, 1833(1), 205–12.
Ney, P.A. Mitochondrial Autophagy: Origins, Significance, and Role of BNIP3 and NIX. Biochim. Biophys. Acta, 2015, 1853 (10 Pt B), 2775–83.
Matsuda, N. Phospho-Ubiquitin: Upending the PINK-Parkin- Ubiquitin Cascade. J. Biochem., 2016, 159(4), 379–85.
Gottlieb, R.A.; Carreira, R.S. Autophagy in Health and Disease. 5. Mitophagy as a Way of Life. Am. J. Physiol. Cell Physiol., 2010, 299(2), C203–10.
Liu, K.E.; Frazier, W.A. Phosphorylation of the BNIP3 C-Terminus Inhibits Mitochondrial Damage and Cell Death without Blocking Autophagy. PLoS One, 2015, 10(6), e0129667
Kale, M.B.; Bajaj, K.; Umare, M.; Wankhede, N.L.; Taksande, B.G.; Umekar, M.J.; Upaganlawar, A. Exercise and Nutraceuticals: Eminent Approach for Diabetic Neuropathy. Curr. Mol. Pharmacol., 2021, 15(1), 108–28.
Gioscia-Ryan, R.A.; Battson, M.L.; Cuevas, L.M.; Zigler, M.C.; Sindler, A.L.; Seals, D.R. Voluntary Aerobic Exercise Increases Arterial Resilience and Mitochondrial Health with Aging in Mice. Aging (Albany. NY), 2016, 8(11), 2897–914.
Joseph, A.M.; Adhihetty, P.J.; Buford, T.W.; Wohlgemuth, S.E.; Lees, H.A.; Nguyen, L.M.D.; Aranda, J.M.; Sandesara, B.D.; Pahor, M.; Manini, T.M.; Marzetti, E.; Leeuwenburgh, C. The Impact of Aging on Mitochondrial Function and Biogenesis Pathways in Skeletal Muscle of Sedentary High-and Low- Functioning Elderly Individuals. Aging Cell, 2012, 11(5), 801–9.
Wankhede, N.L.; Kale, M.B.; Upaganlawar, A.B.; Taksande, B.G.; Umekar, M.J.; Behl, T.; Abdellatif, A.A.H.; Bhaskaran, P.M.; Dachani, S.R.; Sehgal, A.; Singh, S.; Sharma, N.; Makeen, H.A.; Albratty, M.; Dailah, H.G.; Bhatia, S.; Al-Harrasi, A.; Bungau, S. Involvement of Molecular Chaperone in Protein-Misfolding Brain Diseases. Biomed. Pharmacother., 2022, 147, 112647.
Viña, J.; Gomez-Cabrera, M.C.; Borras, C.; Froio, T.; Sanchis- Gomar, F.; Martinez-Bello, V.E.; Pallardo, F.V. Mitochondrial Biogenesis in Exercise and in Ageing. Adv. Drug Deliv. Rev., 2009, 61(14), 1369–74.
Derbré, F.; Gomez-Cabrera, M.C.; Nascimento, A.L.; Sanchis- Gomar, F.; Martinez-Bello, V.E.; Tresguerres, J.A.F.; Fuentes, T.; Gratas-Delamarche, A.; Monsalve, M.; Viña, J. Age Associated Low Mitochondrial Biogenesis may be Explained by Lack of Response of PGC-1α to Exercise Training. Age (Dordr), 2012, 34(3), 669–79.
O’Leary, M.F.; Vainshtein, A.; Iqbal, S.; Ostojic, O.; Hood, D.A. Adaptive Plasticity of Autophagic Proteins to Denervation in Aging Skeletal Muscle. Am. J. Physiol. Cell Physiol., 2013, 304(5), C422–30.
Capitanio, D.; Vasso, M.; De Palma, S.; Fania, C.; Torretta, E.; Cammarata, F.P.; Magnaghi, V.; Procacci, P.; Gelfi, C. Specific Protein Changes Contribute to the Differential Muscle Mass Loss during Ageing. Proteomics, 2016, 16(4), 645–56.
Umare, M.D.; Wankhede, N.L.; Bajaj, K.K.; Trivedi, R.V.; Taksande, B.G.; Umekar, M.J.; Mahore, J.G.; Kale, M.B. Interweaving of Reactive Oxygen Species and Major Neurological and Psychiatric Disorders. Ann. Pharm. Fr., 2022, 80(4), 409–25.
Leduc-Gaudet, J.P.; Picard, M.; Pelletier, F.S.J.; Sgarioto, N.; Auger, M.J.; Vallée, J.; Robitaille, R.; St-Pierre, D.H.; Gouspillou, G. Mitochondrial Morphology is Altered in Atrophied Skeletal Muscle of Aged Mice. Oncotarget, 2015, 6(20), 17923–37.
Stauch, K.L.; Purnell, P.R.; Fox, H.S. Aging Synaptic Mitochondria Exhibit Dynamic Proteomic Changes While Maintaining Bioenergetic Function. Aging (Albany. NY), 2014, 6(4), 320–34.
Bossy-Wetzel, E.; Barsoum, M.J.; Godzik, A.; Schwarzenbacher, R.; Lipton, S.A. Mitochondrial Fission in Apoptosis, Neurodegeneration and Aging. Curr. Opin. Cell Biol., 2003, 15(6), 706–16.
Upaganlawar, A.B.; Dabhekar, S.V.; Chandurkar, P.A.; Kale, M.B.; Wankhede, N.L.; Taksande, B.G.; Umekar, M.J.; Upaganlawar, A.B. Herbal Medicine in the Treatment of Alzheimer’s Disease and Dementia: Phytoconstituent and their Possible Pharmacological Activities. United States: MedDocs Publishers, 2022.
Sebastián, D.; Zorzano, A. When MFN2 (Mitofusin 2) Met Autophagy: A New Age for Old Muscles. Autophagy, 2016, 12(11), 2250–51.
Scarffe, L.A.; Stevens, D.A.; Dawson, V.L.; Dawson, T.M. Parkin and PINK1: Much More than Mitophagy. Trends Neurosci., 2014, 37(6), 315–24.
Krestinina, O.; Azarashvili, T.; Baburina, Y.; Galvita, A.; Grachev, D.; Stricker, R.; Reiser, G. In Aging, the Vulnerability of Rat Brain Mitochondria Is Enhanced Due to Reduced Level of 2’,3’-Cyclic Nucleotide-3’-Phosphodiesterase (CNP) and Subsequently Increased Permeability Transition in Brain Mitochondria in Old Animals. Neurochem. Int., 2015, 80, 41–50.
Rubinsztein, D.C.; Shpilka, T.; Elazar, Z. Mechanisms of Autophagosome Biogenesis. Curr. Biol., 2012, 22(1), R29–34
Hailey, D.W.; Rambold, A.S.; Satpute-Krishnan, P.; Mitra, K.; Sougrat, R.; Kim, P.K.; Lippincott-Schwartz, J. Mitochondria Supply Membranes for Autophagosome Biogenesis during Starvation. Cell, 2010, 141(4), 656–67.
Twig, G.; Shirihai, O.S. The Interplay between Mitochondrial Dynamics and Mitophagy. Antioxid. Redox Signal., 2011, 14(10), 1939–51.
Barnett, A.; Brewer, G.J. Autophagy in Aging and Alzheimer’s Disease: Pathologic or Protective? J. Alzheimers. Dis., 2011, 25(3), 385–94.
Kawashima, T.; Inuzuka, Y.; Okuda, J.; Kato, T.; Niizuma, S.; Tamaki, Y.; Iwanaga, Y.; Kawamoto, A.; Narazaki, M.; Matsuda, T.; Adachi, S.; Takemura, G.; Kita, T.; Kimura, T.; Shioi, T. Constitutive SIRT1 Overexpression Impairs Mitochondria and Reduces Cardiac Function in Mice. J. Mol. Cell. Cardiol., 2011, 51(6), 1026–36.
Takahashi, Y.; Coppola, D.; Matsushita, N.; Cualing, H.D.; Sun, M.; Sato, Y.; Liang, C.; Jung, J.U.; Cheng, J.Q.; Mulé, J.J.; Pledger, W.J.; Wang, H.G. Bif-1 Interacts with Beclin 1 through UVRAG and Regulates Autophagy and Tumorigenesis. Nat. Cell Biol., 2007, 9(10), 1142–51.
Takahashi, Y.; Meyerkord, C.L.; Hori, T.; Runkle, K.; Fox, T.E.; Kester, M.; Loughran, T.P.; Wang, H.G. Bif-1 Regulates Atg9 Trafficking by Mediating the Fission of Golgi Membranes during Autophagy. Autophagy, 2011, 7(1), 61–73.
Aglawe, M.M.; Kale, M.B.; Rahangdale, S.R.; Kotagale, N.R.; Umekar, M.J.; Taksande, B.G. Agmatine Improves the Behavioral and Cognitive Impairments Associated with Chronic Gestational Ethanol Exposure in Rats. Brain Res. Bull., 2021, 167, 37–47.
Takahashi, Y.; Meyerkord, C.L.; Wang, H.G. BARgaining Membranes for Autophagosome Formation: Regulation of Autophagy and Tumorigenesis by Bif-1/Endophilin B1. Autophagy, 2008, 4(1), 121–24.
Marde, V.S.; Tiwari, P.L.; Wankhede, N.L.; Taksande, B.G.; Upaganlawar, A.B.; Umekar, M.J.; Kale, M.B. Neurodegenerative Disorders Associated with Genes of Mitochondria. Futur. J. Pharm. Sci., 2021, 7(1), 66.
Lee, I.H.; Cao, L.; Mostoslavsky, R.; Lombard, D.B.; Liu, J.; Bruns, N.E.; Tsokos, M.; Alt, F.W.; Finkel, T. A Role for the NAD-Dependent Deacetylase Sirt1 in the Regulation of Autophagy. Proc. Natl. Acad. Sci. U. S. A., 2008, 105(9), 3374–9.
Reef, S.; Zalckvar, E.; Shifman, O.; Bialik, S.; Sabanay, H.; Oren, M.; Kimchi, A. A Short Mitochondrial Form of P19ARF Induces Autophagy and Caspase-Independent Cell Death. Mol. Cell, 2006, 22(4), 463–75.
Betin, V.M.S.; Lane, J.D. Caspase Cleavage of Atg4D Stimulates GABARAP-L1 Processing and Triggers Mitochondrial Targeting and Apoptosis. J. Cell Sci., 2009, 122(Pt 14), 2554–66.
Terman, A.; Kurz, T.; Navratil, M.; Arriaga, E.A.; Brunk, U.T. Mitochondrial Turnover and Aging of Long-Lived Postmitotic Cells: The Mitochondrial-Lysosomal Axis Theory of Aging. Antioxid. Redox Signal., 2010, 12 (4), 503–35.
Wankhede, N.; Samrit, S.; Umare, M.; Bajaj, K.; Trivedi, R.; Umekar, M.; Kale, M. Biomarkers of Cancer: A Comprehensive Review. Adv. Biores., 2021, 12 (1), 221–233.
Los, M.; Wesselborg, S.; Schulze-Osthoff, K. The Role of Caspases in Development, Immunity, and Apoptotic Signal Transduction: Lessons from Knockout Mice. Immunity, 1999, 10(6), 629–39.
Rashedi, I.; Panigrahi, S.; Ezzati, P.; Ghavami, S.; Los, M. Autoimmunity and Apoptosis--Therapeutic Implications. Curr. Med. Chem., 2007, 14(29), 3139–51.
Ghavami, S.; Hashemi, M.; Ande, S.R.; Yeganeh, B.; Xiao, W.; Eshraghi, M.; Bus, C.J.; Kadkhoda, K.; Wiechec, E.; Halayko, A.J.; Los, M. Apoptosis and Cancer: Mutations within Caspase Genes. J. Med. Genet., 2009, 46(8), 497–510.
Stroh, C.; Cassens, U.; Samraj, A.K.; Sibrowski, W.; Schulze- Osthoff, K.; Los, M. The Role of Caspases in Cryoinjury: Caspase Inhibition Strongly Improves the Recovery of Cryopreserved Hematopoietic and Other Cells. FASEB J., 2002, 16(12), 1651–3.
Taksande, B.G.; Kotagale, N.R.; Patel, M.R.; Shelkar, G.P.; Ugale, R.R.; Chopde, C.T. Agmatine, an Endogenous Imidazoline Receptor Ligand Modulates Ethanol Anxiolysis and Withdrawal Anxiety in Rats. Eur. J. Pharmacol., 2010, 637(1-3), 89–101.
Ghavami, S.; Mutawe, M.M.; Schaafsma, D.; Yeganeh, B.; Unruh, H.; Klonisch, T.; Halayko, A.J. Geranylgeranyl Transferase 1 Modulates Autophagy and Apoptosis in Human Airway Smooth Muscle. Am. J. Physiol. Lung Cell. Mol. Physiol., 2012, 302(4), L420–8.
Los, M.; Van de Craen, M.; Penning, L.C.; Schenk, H.; Westendorp, M.; Baeuerle, P.A.; Dröge, W.; Krammer, P.H.; Fiers, W.; Schulze- Osthoff, K. Requirement of an ICE/CED-3 Protease for Fas/APO-1- Mediated Apoptosis. Nature, 1995, 375(6526), 81–3.
Fuchs, Y.; Steller, H. Programmed Cell Death in Animal Development and Disease. Cell, 2011, 147(4), 742–58.
Hashemi, M.; Fazaeli, A.; Ghavami, S.; Eskandari-Nasab, E.; Arbabi, F.; Mashhadi, M.A.; Taheri, M.; Chaabane, W.; Jain, M.V.; Łos, M.J. Functional Polymorphisms of FAS and FASL Gene and Risk of Breast Cancer-Pilot Study of 134 Cases. PLoS One, 2013, 8(1), e53075.
Ghavami, S.; Yeganeh, B.; Stelmack, G.L.; Kashani, H.H.; Sharma, P.; Cunnington, R.; Rattan, S.; Bathe, K.; Klonisch, T.; Dixon, I.M.C.; Freed, D.H.; Halayko, A.J. Apoptosis, Autophagy and ER Stress in Mevalonate Cascade Inhibition-Induced Cell Death of Human Atrial Fibroblasts. Cell Death Dis., 2012, 3(6), e330.
Adams, J.M.; Cory, S. Apoptosomes: Engines for Caspase Activation. Curr. Opin. Cell Biol., 2002, 14(6), 715–20.
Ghavami, S.; Kerkhoff, C.; Chazin, W.J.; Kadkhoda, K.; Xiao, W.; Zuse, A.; Hashemi, M.; Eshraghi, M.; Schulze-Osthoff, K.; Klonisch, T.; Los, M. S100A8/9 Induces Cell Death Via a Novel, RAGE-Independent Pathway that Involves Selective Release of Smac/DIABLO and Omi/HtrA2. Biochim. Biophys. Acta, 2008, 1783(2), 297–311.
Gottfried, Y.; Rotem, A.; Lotan, R.; Steller, H.; Larisch, S. The Mitochondrial ARTS Protein Promotes Apoptosis through Targeting XIAP. EMBO J., 2004, 23(7), 1627–35.
Taksande, B.G.; Kotagale, N.R.; Nakhate, K.T.; Mali, P.D.; Kokare, D.M.; Hirani, K.; Subhedar, N.K.; Chopde, C.T.; Ugale, R.R. Agmatine in the Hypothalamic Paraventricular Nucleus Stimulates Feeding in Rats: Involvement of Neuropeptide Y. Br. J. Pharmacol., 2011, 164(2b), 704–18.
Candé, C.; Cecconi, F.; Dessen, P.; Kroemer, G. Apoptosis- Inducing Factor (AIF): Key to the Conserved Caspase-Independent Pathways of Cell Death? J. Cell Sci., 2002, 115 (Pt 24), 4727–34.
Daugas, E.; Susin, S.A.; Zamzami, N.; Ferri, K.F.; Irinopoulou, T.; Larochette, N.; Prévost, M.C.; Leber, B.; Andrews, D.; Penninger, J.; Kroemer, G. Mitochondrio‐Nuclear Translocation of AIF in Apoptosis and Necrosis. FASEB J., 2000, 14(5), 729–39.
Green, D.; Kroemer, G. The Central Executioners of Apoptosis: Caspases or Mitochondria? Trends Cell Biol., 1998, 8(7), 267–71.
Kluck, R.M.; Bossy-Wetzel, E.; Green, D.R.; Newmeyer, D.D. The Release of Cytochrome C from Mitochondria: A Primary Site for Bcl-2 Regulation of Apoptosis. Science, 1997, 275(5303), 1132–6.
Green, D.R.; Reed, J.C. Mitochondria and Apoptosis. Science, 1998, 281(5381), 1309–12.
Patterson, S.D.; Spahr, C.S.; Daugas, E.; Susin, S.A.; Irinopoulou, T.; Koehler, C.; Kroemer, G. Mass Spectrometric Identification of Proteins Released from Mitochondria Undergoing Permeability Transition. Cell Death Differ., 2000, 7(2), 137–44.
Li, P.; Nijhawan, D.; Budihardjo, I.; Srinivasula, S.M.; Ahmad, M.; Alnemri, E.S.; Wang, X. Cytochrome C and dATP-Dependent Formation of Apaf-1/Caspase-9 Complex Initiates an Apoptotic Protease Cascade. Cell, 1997, 91(4), 479–89.
Shimizu, S.; Narita, M.; Tsujimoto, Y. Bcl-2 Family Proteins Regulate the Release of Apoptogenic Cytochrome c by the Mitochondrial Channel VDAC. Nature, 1999, 399(6735), 483–7.
Hengartner, M.O. The Biochemistry of Apoptosis. Nature, 2000, 407(6805), 770–6.
Harman, D. Free Radical Theory of Aging. Mutat. Res., 1992, 275 (3-6), 257–66.
Harman, D. Free Radical Theory of Aging: History. EXS, 1992, 62, 1–10.
Harman, D. Free-Radical Theory of Aging. Increasing the Functional Life Span. Ann. N. Y. Acad. Sci., 1994, 717(1), 1–15.
Cadenas, E.; Davies, K.J. Mitochondrial Free Radical Generation, Oxidative Stress, and Aging. Free Radic. Biol. Med., 2000, 29(3- 4), 222–30.
Giulivi, C.; Poderoso, J.J.; Boveris, A. Production of Nitric Oxide by Mitochondria. J. Biol. Chem., 1998, 273(18), 11038–43.
Leeuwenburgh, C.; Heinecke, J.W. Oxidative Stress and Antioxidants in Exercise. Curr. Med. Chem., 2001, 8(7), 829–38.
Upaganlawar, A.B.; Wankhede, N.L.; Kale, M.B.; Umare, M.D.; Sehgal, A.; Singh, S.; Bhatia, S.; Al-Harrasi, A.; Najda, A.; Nurzyńska-Wierdak, R.; Bungau, S.; Behl, T. Interweaving Epilepsy and Neurodegeneration: Vitamin E as a Treatment Approach. Biomed. Pharmacother., 2021, 143, 112146.
Boveris, A.; Costa, L.E.; Poderoso, J.J.; Carreras, M.C.; Cadenas, E. Regulation of Mitochondrial Respiration by Oxygen and Nitric Oxide. Ann. N. Y. Acad. Sci., 2000, 899, 121–35.
Sastre, J.; Pallardö, F.V.; Viña, J. Mitochondrial Oxidative Stress Plays a Key Role in Aging and Apoptosis. IUBMB Life, 2000, 49(5), 427–35.
Beckman, K.B.; Ames, B.N. Mitochondrial Aging: Open Questions. Ann. N. Y. Acad. Sci., 1998, 854, 118–27.
Beckman, K.B.; Ames, B.N. Endogenous Oxidative Damage of mtDNA. Mutat. Res., 1999, 424(1-2), 51–8.
Barja, G.; Herrero, A. Oxidative Damage to Mitochondrial DNA is Inversely Related to Maximum Life Span in the Heart and Brain of Mammals. FASEB J., 2000, 14(2), 312–8.
Aglawe, M.M.; Taksande, B.G.; Kuldhariya, S.S.; Chopde, C.T.; Umekar, M.J.; Kotagale, N.R. Participation of Central Imidazoline Binding Sites in Antinociceptive Effect of Ethanol and Nicotine in Rats. Fundam. Clin. Pharmacol., 2014, 28(3), 284–93.
Sohal, R.S.; Agarwal, S.; Sohal, B.H. Oxidative Stress and Aging in the Mongolian Gerbil (Meriones Unguiculatus). Mech. Ageing Dev., 1995, 81(1), 15–25.
Sohal, R.S.; Orr, W.C. Relationship between Antioxidants, Prooxidants, and the Aging Process. Ann. N. Y. Acad. Sci., 1992, 663(1), 74–84.
Barja, G. The Flux of Free Radical Attack through Mitochondrial DNA is Related to Aging Rate. Aging (Milano)., 2000, 12(5), 342–55.
Warner, H.R.; Hodes, R.J.; Pocinki, K. What Does Cell Death Have to Do with Aging? J. Am. Geriatr. Soc. 1997, 45(9), 1140–6.
Phaneuf, S.; Leeuwenburgh, C. Apoptosis and Exercise. Med. Sci. Sports Exerc., 2001, 33(3), 393–6.
Warner, H.R. Apoptosis: A Two-Edged Sword in Aging. Ann. N. Y. Acad. Sci., 1999, 887, 1–11.
Costantini, P.; Chernyak, B.V.; Petronilli, V.; Bernardi, P. Modulation of the Mitochondrial Permeability Transition Pore by Pyridine Nucleotides and Dithiol Oxidation at Two Separate Sites. J. Biol. Chem., 1996, 271(12), 6746–51.
Zoratti, M.; Szabò, I. The Mitochondrial Permeability Transition. Biochim. Biophys. Acta, 1995, 1241(2), 139–76.
Bernardi, P. The Permeability Transition Pore. Control Points of a Cyclosporin A-Sensitive Mitochondrial Channel Involved in Cell Death. Biochim. Biophys. Acta, 1996, 1275(1-2), 5–9.
Mather, M.; Rottenberg, H. Aging Enhances the Activation of the Permeability Transition Pore in Mitochondria. Biochem. Biophys. Res. Commun., 2000, 273(2), 603–8.
Nitahara, J.A.; Cheng, W.; Liu, Y.; Li, B.; Leri, A.; Li, P.; Mogul, D.; Gambert, S.R.; Kajstura, J.; Anversa, P. Intracellular Calcium, DNase Activity and Myocyte Apoptosis in Aging Fischer 344 Rats. J. Mol. Cell. Cardiol., 1998, 30(3), 519–35.
Beck, K.F.; Eberhardt, W.; Frank, S.; Huwiler, A.; Messmer, U.K.; Mühl, H.; Pfeilschifter, J. Inducible NO Synthase: Role in Cellular Signalling. J. Exp. Biol., 1999, 202(Pt 6), 645–53.
Boveris, A.; Cadenas, E. Mitochondrial Production of Hydrogen Peroxide Regulation by Nitric Oxide and the Role of Ubisemiquinone. IUBMB Life, 2000, 50(4-5), 245–50.